Chapter 67 Fluid and Electrolyte Issues in Pediatric Critical Illness
Albumin
Several specific concerns about the safety of the use of human albumin in critical care resulted in a large, prospective, randomized, stratified, and very convincing1 study that demonstrated no difference in mortality or in the number of days spent in the ICU in the hospital undergoing ventilation, or undergoing dialysis between those receiving 4% albumin for volume replacement versus those receiving normal saline solution. While this study provides strong evidence for the safety of albumin administration, its use in some specific patient populations will require further studies of adequate power and design. Evidence of efficacy in patients with certain clinical problems needs to be strengthened and must be weighed against its large cost disadvantage. Developing guidelines in individual ICUs may be useful.2 Recombinant3 and pegylated4 albumin preparations are under study.
Starch
Synthetic colloid solutions are less expensive than human albumin and continue to be of interest. Hydroxyethyl starch 6%, 130/0.4 appears to have an improved safety profile compared with earlier products,5 has Food and Drug Administration approval, and may be safe in limited doses and circumstances. Significant questions about safety remain, however.6
Fluid Balance
In pediatric ICU (PICU) patients with significant cardiopulmonary disease, fluid administration requires judgments about volume as well as content. The evolving area of extravascular fluid volume measurement appears to have value in adults7 and has been extended to the pediatric population.8 Its efficacy in pediatrics remains to be determined.
Diabetic Ketoacidosis/Cerebral Edema
Various regimens for limiting hydration fluids during the first 24 hours of diabetic ketoacidosis (DKA) treatment have been utilized and seem to have had some effect in decreasing the incidence of cerebral edema. Knowledgeable observers are aware that cerebral edema has developed in some patients receiving less than the fluid limit and has not developed in many patients who exceeded the limit. Evidence now suggests that cerebral edema occurs when a significant increase in the permeability of the blood-brain barrier allows excessive transudation of intravascular fluid.9 This degree of this change may be associated with the severity of acidosis, and the overall risk of edema would be multifactorial, consistent with clinical observations. Although not primary or universally sufficient, continuing limitation of initial hydration fluids given adequate hemodynamics is certainly a rational aspect of a DKA protocol (also see Chapter 78).10
Hyponatremia
Mild hyponatremia is common in patients with some renal, hepatic, or cardiac issues and occurs predictably in postoperative patients, particularly those with prolonged general anesthesia. The use of isotonic intravenous fluids postoperatively may decrease the frequency of mild hyponatremia,11,12 but severe SIADH or CRSW may lead to profound hyponatremia despite the use of isotonic intravenous (IV) fluid.13–15 Appropriate monitoring of electrolytes in patients at risk will allow an early and specific response to evolving hyponatremia, whether related to water retention or sodium excretion. Even knowing the underlying diagnosis in severe, acute hyponatremia may not be as important as prompt, careful osmolality management with a continuing cycle of monitoring and therapeutic adjustment.16
Pathophysiology and Etiology
Decreased Total Body Sodium
Loss of total body sodium results in hyponatremia if total body water is retained in relative excess of the sodium loss. Hypovolemic stimulation of ADH release may overwhelm osmotic ADH control, maintaining water retention despite hyponatremia and hypoosmolality. Sodium deficit may occur through extrarenal or renal losses. In children, extrarenal losses most often occur from vomiting and diarrhea. In critically ill patients, large extrarenal losses may result from fluid sequestration occurring with septicemia, peritonitis, pancreatitis, ileus, rhabdomyolysis, and burns. Various skin conditions including burns may cause large transcutaneous losses. Renal losses include diuretic use, osmotic diuresis, various salt-losing renal diseases, CRSW, and adrenal insufficiency.17 If renal function is preserved, these conditions, especially with concurrent thiazide or loop diuretics, may result in hyponatremia with hypovolemia and generally are associated with hypokalemic metabolic alkalosis.18 Concentrated urine is produced by the equilibration of fluid in the collecting tubules with the hyperosmotic medullary interstitium, which in turn is generated by sodium chloride (NaCl) reabsorption without water in the ascending limb of the loop of Henle. Thiazides act in the cortical distal tubule and do not impair the ability of ADH to increase water reabsorption in the collecting tubules and collecting duct,18 resulting in thiazide-associated hyponatremia. Osmotic sodium and water losses occur in a child with uncontrolled hyperglycemia with glucosuria, with mannitol use, and during urea diuresis following relief of urinary tract obstruction. Sodium wasting is greater in the presence of massive ketonuria because the keto acids are anions obligating cation losses. Hyperglycemia and mannitol, in addition to inducing urinary sodium and water losses, produce osmotic water movement from the intracellular fluid (ICF) to the extracellular fluid (ECF), further lowering serum sodium. Sodium levels drop about 1.5 mEq/L for every 100 mg/dL rise in blood glucose level. Significant salt wasting may occur with several intrinsic renal diseases and may result in hyponatremia when associated with decreased access to water and sodium (Box 67-1).
Box 67–1 Causes of Hyponatremia
Decreased Total Body Sodium Level
In the absence of these causes, adrenal insufficiency must be considered. Generally it is associated with hyperkalemia and decreased urinary potassium excretion. In each of the renal salt wasting states, urinary sodium excretion is generally greater than 20 mEq/L (fractional excretion of sodium more than 1%). Cerebral salt wasting was reported in 1950, was attributed to SIADH in the 1960s and 1970s, and then was rediscovered. Despite lingering skepticism, its clinical identity continues to be supported (Table 67-1).19–21
Table 67–1 Cerebral-Renal Salt Wasting Syndrome
Trigger | Subarachnoid hemorrhage or other acute intracranial injury or illness |
Onset | Usually a few days after the injury |
Signs | Falling serum Na+, high urine output, high urine Na+ |
Course | Without treatment, it proceeds to intravascular volume depletion, hypoperfusion, and hypotension |
Treatment | Replace salt and water loss; restoring serum Na+ may require 3% NaCl, furosemide, rarely fludrocortisone |
Resolution | Days to weeks |
Differential diagnosis of SIADH, adrenal insufficiency, osmotic diuresis |
Patients typically have neurologic injury with hemorrhage, infection, or a mass and often undergo surgical procedures. The development of hyponatremia may be attributed to SIADH but appears distinct in that large urine volumes contain very high sodium concentrations that lead to rapid depletion of both sodium and ECF volume. A distinct and otherwise unexplained intravascular contraction is expected for diagnosis, may cause a secondary boost in ADH release, and appears to occur in a variety of CNS disorders. Renal sodium loss is central to this diagnosis. Both atrial natriuretic peptide and brain natriuretic peptide are attractive as mediators, but niether has a proved etiological role.22,23 Both cerebral and renal components are key, but the connecting link is unknown, thus some favor cerebral-renal (CRSW) terminology.24 Distinguishing CRSW from complex SIADH may be difficult; however, in severe hyponatremia cases, it may be temporarily unnecessary because the initail therapy is similar.16 Administration of enough concentrated sodium to result in a small increase in osmolality is appropriate, and support of intravascular volume is required, which may entail administering 5 to 6 mL/kg of 3% NaCl followed by isotonic or hypertonic crystalloid as necessary. The absolutely essential part of therapy is the frequent measurement and evaluation of the results of therapy with treatment adjustments as indicated.
Congestive Heart Failure
Hyponatremia in heart failure is associated with a worse prognosis.25,26 In a low cardiac output animal model, renal sodium excretion is decreased primarily from the associated decrease in “effective” blood volume.27 Stimulation of aortic and carotid vasoreceptors and right and left heart volume receptors may result in sodium retention through increased sympathetic activity and stimulation of the renin-angiotensin-aldosterone axis, producing increased renal vascular resistance, decreased glomerular filtration rate (GFR), and resultant decreased urinary sodium excretion. In addition, decreased aldosterone degradation and altered levels of other vasoactive and nonvasoactive substances result in the primary increased tubular sodium reabsorption. Impaired water excretion occurs from both nonosmotic ADH release and decreased distal renal tubular delivery of fluid.11
Cirrhosis
Early in cirrhosis, increased intrahepatic pressure may initiate renal sodium retention before ascites formation. Later, peripheral vasodilation mediated with nitric oxide, multiple arteriovenous fistulae, and a decrease in the effective blood volume occur. These decompensated patients have higher levels of renin, aldosterone, vasopressin, and norepinephrine than do compensated patients with cirrhosis.28
Nephrotic Syndrome
Humoral factors involved in patients with decreased central volume appear to be similar to decompensated patients with cirrhosis. The cause of hyponatremia in patients reported to have normal central volume status is unclear.
Normal Total Body Sodium
Hyponatremia with no evidence of hypovolemia or edema in the pediatric population is almost exclusively associated with SIADH. Renal concentrating and diluting ability ultimately depends on the presence or absence of ADH to modulate water permeability in the collecting duct. Osmoreceptors for ADH reside in the anterior hypothalamus, responding to changes of as little as 1% in plasma osmolality. The nonosmotic stimuli that induce release are generally associated with changes in autonomic neural tone such as those that occur with physical pain, emotional stress, hypoxia, cardiac failure, adrenal insufficiency, and volume depletion. When stimuli for ADH release are competitive, the volume-sensitive pathway appears to rule. Vasopressin synthesized in the hypothalamus is transported in neurosecretory granules to the axonal bulbs in the median eminence and posterior pituitary gland and is released by exocytosis. After release, binding to V2 receptors occurs at the basolateral membrane of the collecting duct, increasing cyclic adenosine 3′, 5′-monophosphate formation, facilitating phosphorylation of aquaporin-2. Incorporation of aquaporin-2–containing vesicles into the apical (luminal) membrane increases cell permeability to reabsorptive water movement.29
Several categories of clinical diseases have been associated with SIADH, including CNS and pulmonary disorders, malignancies, glucocorticoid deficiency, hypothyroidism, and adverse effects of numerous drugs (Box 67-2).30
Postoperative pediatric ICU patients frequently have increased ADH release and are at risk of (usually mild) hyponatremia.31,32 The appropriate use of isotonic intravenous fluid in these patients will decrease the incidence of mild hyponatremia. For patients with severe SIADH or CRSW, the use of isotonic fluids alone may not be adequate to prevent life-threatening complications. Monitoring sodium and avoiding large amounts of hypotonic fluid is mandatory. SIADH should be considered when hyponatremia occurs in the absence of hypovolemia, edema, endocrine dysfunction, renal failure, or suspect drugs. Urine osmolality is inappropriately high compared with plasma osmolality. A urine osmolality of 200 to 250 mOsm does not rule out the diagnosis because a decrease of 4 to 5 mEq/L below normal in serum sodium should maximally inhibit ADH secretion with a resultant urine osmolality of less than 100 mOsm. The urinary sodium level is generally more than 20 mEq/L; however, it can be much less than 20 mEq/L in patients who are provided a low sodium intake or in whom some degree of volume depletion occurs concurrently.33 Urinary sodium may be generous, but the overall amount of sodium excretion should not grossly exceed intake without provoking consideration of a salt-wasting syndrome.
Signs and Symptoms
The severity of signs and symptoms depends on the rapidity of development. Acute decreases in sodium are associated with lethargy, apathy, and disorientation often accompanied by nausea, vomiting, and muscle cramps. No predictable correlation exists between the degree of hyponatremia and its resulting symptoms. In general, however, most patients exhibit seizures and coma with acute decreases in sodium to less than 120 mEq/L. Other signs include decreased deep tendon reflexes, pathologic reflexes, pseudobulbar palsy, and Cheyne-Stokes respiratory pattern. Signs and symptoms are related to cellular swelling and resultant cerebral edema, which may be severe enough to result in herniation.34 Patients in whom hyponatremia develops over several days to weeks may be symptom free or may have nonspecific symptoms of nausea, vomiting, and lethargy.
Treatment
Severe hyponatremia is associated with significant morbidity and mortality. A decrease in the serum sodium concentration would be expected to cause a shift of water from the ECF to the ICF compartment, resulting in generalized cellular edema. When this swelling occurs in the brain, which is limited by the presence of meninges and the cranium, neurologic symptoms are expected. Cerebral edema and transtentorial herniation may result. Hyponatremia developing over a more prolonged period is unlikely to cause herniation. Brain cells prevent massive swelling when gradual hyponatremia is produced by the extrusion of electrolytes and other osmolytes. Total brain amino acid content, particularly taurine, is strikingly decreased in hyponatremic rats with prolonged deficits. Brain water content is also lower in these animals. These data suggest that brain edema develops when the plasma/brain osmotic gradient reaches a critical level before these adaptive processes have been fully developed. Hyponatremia that has been present less than 4 hours can be corrected promptly. However, when adaptation of brain cells has already occurred, a rapid rise in serum sodium concentration may induce a shift of water from the ICF to the ECF compartment, resulting in brain dehydration, brain injury, and the osmotic demyelination syndrome.35 Originally noted in the pons, both pontine and extrapontine myelinolysis has been reported in children.36–38 Extrapontine sites have included the cerebellum, thalamus, lateral geniculate body, and hippocampus. Osmotic demyelination can occur without hyponatremia as a starting point.38,39 Large bolus doses of hypertonic saline solution may place the patient at risk regardless of starting sodium concentration. Electrolyte fluctuations around the time of liver transplantation may account for the risk of myelinolysis noted in these patients.40 Even rapid correction of hypernatremia is a possible cause of myelinolysis and suggests that pressure effects may be capable of causing damage to myelinated structures. Symptoms of osmotic demyelination may include obtundation, quadriplegia, pseudobulbar palsy, tremor, amnesia, seizures, and coma.41,42 Approaches to patients with hyponatremia may vary depending on whether the hyponatremia has developed suddenly, that is, in fewer than 4 hours, in which case rapid reversal may be safe. If asymptomatic hyponatremia has developed over many hours, or has developed over a longer period (i.e., chronic hyponatremia), gradual, conservative reversal is likely to be uncomplicated. In planning treatment it is critical to determine the presence or absence of CNS symptoms or imaging suggestive of cerebral edema. Development of CNS cellular swelling and symptoms is more likely with acute hyponatremia or with severe chronic hyponatremia.43,44 In these patients, an initial small but rapid correction of serum sodium, about 5 mEq/L, should stabilize or begin to reverse cerebral swelling and avoid impending herniation. Subsequently, further correction of the hyponatremia should occur more slowly.
A dose of 3% saline solution at 5 to 6 mL/kg would be expected to initially raise the serum sodium approximately 5 mEq/L. The subsequent correction rate for patients with acute hyponatremia with CNS symptoms or for any patient with chronic hyponatremia should raise the serum sodium no more than 0.5 mEq/L/h. For patients with acute hyponatremia but no CNS symptoms, rates of 0.7 to 1 mEq/L/h have been reported without patient morbidity or mortality. In many patients, a regimen of hypertonic 3% saline solution at 1 to 2 mL/kg/h plus periodic administration of a loop diuretic results in an appropriate correction for those patients for whom “rapid” correction is safe. Further correction may require isotonic fluids or a mixture of isotonic and hypertonic fluids, particularly in patients with CRSW. In resistant, severe CRSW, mineralocorticoid treatment has been helpful in several reports.45,46 Other protocol approaches are available.47 CNS symptoms that develop during correction suggest osmotic demyelination and have occurred most frequently in patients or experimental animals that are euvolemic rather than hypovolemic. At least three patients have been reported who had symptoms of osmotic demyelination and whose course was subsequently reversed; their serum sodium level was decreased to its nadir followed by a slower rate of correction resulting in recovery without neurologic sequelae.48–50 Conivaptan, an ADH V1 and V2 receptor antagonist, is in use as an IV agent in adults for treatment of hyponatremia, particularly in fluid-retaining states.51,52 This receptor blocker group53 increases urine volume and reduces urine osmolality. Pediatric usage has been reported,54 but further study of kinetics, safety, and efficacy will be needed to clarify the clinical role in pediatrics. In the patient in whom less severe hyponatremia occurs (i.e., serum sodium 125 to 130 mEq/L), slow correction through water restriction, occasionally with the use of oral sodium supplements, is all that is required for normalization to occur. In the patient with hypovolemia, volume status clearly must be corrected in addition to the hyponatremia.
Hypernatremia
Low Total Body Sodium Level
Patients with a low total body sodium level have a loss of water in relative excess of sodium losses. Because the ECF space is hyperosmolar, water movement from the ICF occurs with resulting ICF dehydration. Therefore the ECF space is somewhat preserved until extreme degree of hypovolemia has occurred. Losses of sodium and water may be extrarenal or renal (Box 67-3).
In the pediatric patient, extrarenal causes are commonly seen from vomiting and diarrhea, although hospital-acquired hypernatremia from insufficient free water administration is a major concern.55,56 Excessive sweating with inadequate replacement also may occur. Renal causes of hypernatremia include osmotic diuresis from mannitol, hyperglycemia, or increased urea excretion. Urine is either hypotonic or isotonic, and urine sodium is more than 20 mEq/L. Infants are particularly susceptible to hypernatremic dehydration because their surface area/weight ratio is high and their relative renal immaturity results in greater water losses for excretion of a solute load compared with older children and adults.57 Insufficient maternal lactation places small infants at risk of hypernatremic dehydration. Infants with prolonged hospitalization because of bronchopulmonary dysplasia seem particularly susceptible to hypernatremic dehydration with intercurrent gastrointestinal illnesses.
Normal Total Body Sodium Level
Loss of water occurs without excessive sodium losses in some conditions. Extrarenal causes include increased respiratory losses as may occur with tachypnea, hyperventilation, or mechanical ventilation with inadequate humidification and increased skin losses associated with fever, burns, extreme prematurity, or use of phototherapy or radiant warmers in the neonate without adequate water replacement. Renal losses result from acquired or congenital diabetes insipidus (DI), either central or nephrogenic. Acquired forms of DI are more commonly seen by the intensivist. Major insults resulting in central DI include head trauma, tumors, infections, hypoxic injury, neurosurgical procedures, and nontraumatic brain death. Often, in experimental animals and in humans, three stages occur: (1) an initial polyuric phase (hours to several days); (2) a period of antidiuresis probably due to ADH release from injured axons (hours to days); and (3) a second period of DI that may or may not resolve.58,59 Sudden onset of polyuria is characteristic, as is polydipsia (in the conscious patient). In the critically ill patient, lack of access to increased water may result in life-threatening hypernatremia.60 Patients with the rare congenital nephrogenic DI may have repeated bouts of hypernatremic dehydration, resulting from x-linked alteration of the vasopressin receptor V2 or the autosomal recessive changes in the aquaporin II water channel physiologically regulated by ADH.61 Acquired forms are much more common, and manifestations are less severe. Causes of DI are shown in Box 67-4.
Increased Total Body Sodium Level
Hypernatremia with an increased total body sodium level is most often an iatrogenic problem. In the critical care unit, administration of hypertonic solutions of sodium bicarbonate during resuscitation efforts or as therapy for intractable metabolic acidosis is often responsible for this condition. Particularly at risk is the patient after cardiac arrest, when renal failure from hypoxic injury may ensue as well. Other causes include excessive hypertonic NaCl administration for treatment of hyponatremia, near drowning in salt water, ingestion by infants of improperly diluted formula, inadvertent intravascular infusion of hypertonic saline solution during a therapeutic abortion, and dialysis against a high sodium concentration. Normonatremic patients with massive edema who undergo a forced diuresis frequently become mildly hypernatremic because the induced urine may be hypotonic and water loss exceeds sodium loss.
Hypernatremia is purposely induced in patients with head trauma as a form of osmotherapy for control of intracranial hypertension (also see Chapter 59).62,63 Such patients have tolerated a serum sodium of 175 mEq/L when carefully managed. When the ECF osmolality of these patients is manipulated, the risks involved with rapid changes in either direction must be kept in mind.
Signs and Symptoms
Clinical manifestations of hypernatremia relate predominantly to the CNS. Marked irritability, a high-pitched cry, a decrease in sensorium varying from lethargy to coma, normal or increased muscle tone, and frank seizure activity may occur in children with development of severe hypernatremia over 48 hours or more. Hyperglycemia and hypocalcemia also may occur. In infants with acute hypernatremia, vomiting, fever, respiratory distress, spasticity, tonic-clonic seizure activity, and coma are common.64 Death from respiratory failure occurred in experimental animals when serum osmolality reached about 430 mOsm/kg.64 Mortality in children has ranged from 10% to about 45% with chronic and acute hypernatremia, respectively. Morbidity in survivors also may be high.
Anatomic changes seen with the hyperosmolar state include loss of volume of brain cells with resultant tearing of cerebral vessels, capillary and venous congestion, subcortical or subarachnoid bleeding, and venous sinus thrombosis. During the first 4 hours of experimental acute hypernatremia, brain water significantly decreases while the concentration of solutes (electrolytes and glucose) increases. A small portion of increased osmolality is not accounted for by the increase in electrolytes and glucose, termed idiogenic osmoles. By 48 hours, however, brain water has returned to normal with idiogenic osmoles (amino acids, polyhydric alcohols, and urea and trimethylamines) accounting for about 60% of the increased osmoles.65 The central demyelination syndrome has been seen in patients with hypernatremia. It remains unclear as to whether it results from a rapid rise in serum osmolality or from some other aspect of the clinical course.
Treatment
Whenever possible, therapy of hypernatremia should address correction of the underlying disease process as a primary goal. Correction of dehydration with slow hypernatremia correction is the target. When sodium exceeds 165 mEq/L, isotonic fluid and colloid may be used for correction of shock or circulatory collapse and initial reversal of hypernatremia. Some data are available regarding the ideal rate of correction. Numerous fatal cases of cerebral edema have occurred with correction over a 24-hour period, leading to recommendations for correction over no less than a 48-hour period66,67 and general agreement that plasma osmolality should not be decreased more rapidly than 2 mOsm/hr (1 mEq/h sodium). Patients with serum sodium levels greater than 165 mEq/L for more than 48 hours deserve particular care, and a correction rate of no more than 1 mOsm/h may be appropriate. Thus corrections from extreme hypernatremia may take several days. This slower rate of correction appears to allow time for dissipation of idiogenic osmoles without development of cerebral edema. Estimated deficits, ongoing maintenance requirements, and additional excessive losses must be accounted for in calculations of amount of fluid replacement. Although it is not frequently required, calculation of tonicity balance may give an accurate analysis of the disturbance.
Potassium
The transcellular distribution of potassium establishes the resting membrane potential of cells, including the myocardium. Factors involved in total body distribution include acid-base status, insulin, catecholamines, magnesium, and aldosterone.68,69 Acidemia tends to increase the serum potassium, and alkalemia lowers it. The type of acid-base disturbance (metabolic or respiratory), the duration of the disturbance, and the nature of the anion accompanying the hydrogen ion in metabolic acidosis, however, are important in determining what effect a particular acid-base disorder may have on potassium concentration.70 The greatest effect occurs with mineral acids, where cellular permeability to accompanying anions is low so that hydrogen ions enter cells alone. In this case, potassium must move out of the cells to maintain electrical neutrality. In metabolic acidosis caused by organic acids such as lactate and β-hydroxybutyrate, however, hydrogen ions presumably enter cells with their accompanying anions so that cation exchange with potassium is not required.
Hyperkalemia associated with DKA may reflect the effects of hyperosmolality and decreased circulating insulin rather than the acidemia itself.71,72 Epinephrine initially causes serum potassium to rise, reflecting release from the liver, and then fall, as potassium moves into cells.73 This effect is abolished by β-adrenergic blocking drugs. In rats with experimentally induced insulin deficiency, both epinephrine and the peripheral sympathetic nervous system have been shown to regulate cell potassium uptake.74 Change in intracellular magnesium may affect the sodium-potassium adenosine triphosphatase (ATPase) pump and alter the transcellular distribution. All of these mechanisms, however, are generally representative of fine-tuning in potassium homeostasis. Ultimately, potassium balance is regulated through excretion by the kidney and to a lesser extent the gastrointestinal (GI) tract. Conditions associated with massive cell lysis require management of the sudden shift of intracellular potassium into the ECF, which occurs frequently in the presence of compromised renal function.
Most of the filtered potassium is absorbed before the distal nephron in normal kidneys.75 The potassium excreted in the urine then is mainly due to secretion in the distal convoluted tubule and cortical collecting duct. As with sodium, the kidney’s capacity to vary potassium excretion is profound, ranging from a low of approximately 5 mEq/L to amounts exceeding 100 mEq/L of urine. Factors influencing renal potassium excretion include mineralocorticoid and glucocorticoid hormones, acid-base balance, anion effects, tubular fluid flow rate, sodium intake, potassium intake, ICF and plasma potassium concentrations, and diuretics.75 Aldosterone is a major kaliuretic hormone. Metabolic acidosis decreases and metabolic alkalosis increases intracellular potassium activity in cells of the distal tubule, causing enhanced potassium secretion during alkalosis and reabsorption during acidosis. Fluid delivery to the distal tubule probably enhances potassium secretion by two mechanisms: (1) the faster fluid moves past the secretory site, and a greater amount of potassium can be secreted; and (2) because tubular fluid potassium concentration decreases as flow rate increases, a favorable gradient for potassium movement is maintained at high flow rates.76
Hypokalemia
Causes of Hypokalemia
Hypokalemia Without Potassium Deficit
The detection of a low serum potassium level may reflect a true deficit in total body stores or an apparent deficit from the shift of this ion from the ECF to the ICF pool. A shift to the ICF pool may occur in patients with alkalemia, exogenous administration and likely endogenous release of β-adrenergic agonists, familial hypokalemic periodic paralysis,77 barium poisoning,78 and excess insulin. In the case of alkalemia, potassium moves into the cell in exchange for H+ in an attempt to maintain extracellular pH. The pediatric patient with alkalemia also may have a true potassium deficit because of decreased potassium intake or increased losses. Numerous studies have confirmed an acute decrease in serum potassium after administration of β2 agonists, including epinephrine and albuterol, presumably from cellular influx of potassium into skeletal muscle cells. Endogenous levels of epinephrine equal to or greater than levels obtained by exogenous administration have been reported in adults with acute myocardial infarction and in children after near drowning.79
Periodic paralysis is a rare autosomal dominant disorder presenting with intermittent episodes of profound muscle weakness associated with a sudden fall in serum potassium concentration precipitated by a high-carbohydrate/low-potassium diet, exercise, infection, stress, or alcohol ingestion.77,80 Barium poisoning can produce hypokalemic paralysis and weakness probably by competitive blockade of inward rectifying potassium channels. Insulin produces potassium shifts into the liver in association with glycogen formation and into muscle cells.
Hypokalemia with Potassium Deficit
A deficit in total body potassium may occur from decreased intake, from renal losses, or from GI losses. Poor intake coupled with increased GI or renal losses is common. Any history of geophagia in the toddler should be sought because ingested clay binds potassium in the GI tract.81
Renal Losses
Major categories that may be seen in the ICU setting include diuretic use, osmotic diuresis, use of various other drugs, renal tubular acidosis, hyperaldosteronism, magnesium deficiency, and recovery from acute renal failure (ARF). Osmotic diuresis from glucosuria is the primary cause of renal potassium wasting in patients with prolonged DKA that can present with ventricular arrhythmia. Primary aldosteronism, congenital adrenal hyperplasia, adrenal adenoma, and familial idiopathic hyperaldosteronism are rare in children and even rarer in the pediatric ICU setting. Secondary hyperaldosteronism is common, however, either from volume depletion or from CHF, cirrhosis, or nephrotic syndrome. Patients with the latter conditions, however, rarely have severe hypokalemia unless they are additionally treated with diuretics. The infant with Bartter’s syndrome may initially come to the ICU because of multiple metabolic derangements including hypokalemia, metabolic alkalosis, and often hypomagnesemia and hyperuricemia. Other findings include weakness, polyuria, and failure to thrive, with elevated renin and aldosterone levels in the absence of hypertension.82 Other conditions associated with elevated renin secretion, secondary hyperaldosteronism, and hypokalemia include renal artery stenosis, malignant hypertension, renin-producing tumor, or Wilms’ tumor. Additional mechanisms include secondary hyperaldosteronism and increased distal tubular fluid delivery. The severity of hypokalemia is often masked by the shift of potassium from the ICF to ECF space related to insulin deficiency, metabolic acidosis, and hypertonicity.
If a brisk diuresis is induced by loop, thiazide, or osmotic diuretics, kaliuresis may result, and this may lead to acute hypokalemia. Use of combinations of these diuretics, in particular loop diuretics and metolazone, may accentuate potassium losses. Each agent leads to increased distal delivery of tubular fluid. Other drugs that induce excessive renal losses include amphotericin B (kaliuresis with reduced renal function and tubular injury); aminoglycosides, particularly gentamicin; and high-dose penicillin and carbenicillin, which produce an osmotic load in addition to acting as nonreabsorbable anions.83 Renal tubular acidosis or hypomagnesemia may cause renal potassium wasting.84

Full access? Get Clinical Tree
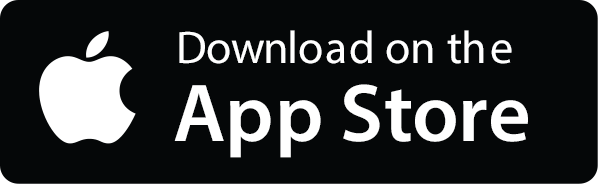
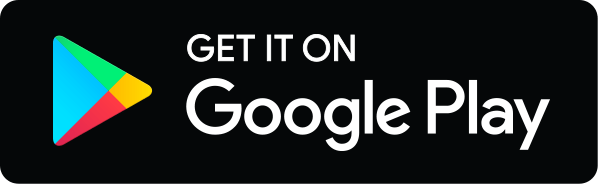