1. Fetal surgery is a rapidly evolving specialty with multiple fetal interventions being performed at different stages of pregnancy.
2. Pregnancy results in various anatomic and physiologic changes that impact anesthetic management.
3. Maternal safety is paramount and maternal risks should be minimized.
4. A multidisciplinary approach with effective teamwork and communication is vital.
5. Maintenance of uteroplacental blood flow is crucial during fetal interventions.
6. Advances in surgical techniques and instrumentation for minimally invasive fetoscopic interventions have altered anesthetic management.
7. EXIT procedures allow successful transition to extrauterine life and their indications continue to evolve.
8. Open fetal surgery has limited indications and managing preterm labor continues to be a vexing problem.
I. Introduction. Fetal surgery is a rapidly evolving specialty. Advances in the field of prenatal imaging, including three-dimensional ultrasonography and fetal magnetic resonance imaging (MRI), have allowed a better understanding of the natural history and pathophysiology of fetal malformations. In addition, advances in minimally invasive surgical techniques and instrumentation have led to a continued refinement of the approach to fetal interventions. A wide range of fetal interventions are being performed at different stages of pregnancy in order to save the life of the fetus or prevent permanent organ damage. The mother has been described as an innocent bystander without any direct physical benefit (1,2). The risks to the mother and the fetus must be balanced against the benefit to the fetus. Fetal interventions pose several anesthetic challenges and a thorough understanding of maternal physiologic changes during pregnancy, fetal physiology, and the pathophysiology of fetal malformations is critical.
II. Maternal considerations
A. Physiologic changes of pregnancy. Pregnancy results in various anatomic and physiologic changes that impact anesthetic management.
1. Airway/respiratory
a. Capillary engorgement of the respiratory mucosa during pregnancy predisposes the upper airway to trauma and bleeding from airway manipulation (3,4). Nasotracheal intubation is likely to cause epistaxis and is discouraged. Pregnant women are potentially more difficult to intubate secondary to edema and increased friability of the upper airway mucosa (5,6). A smaller endotracheal tube (6.0 to 6.5 mm) should be readily available.
b. Pregnancy results in a progressive increase in oxygen consumption to meet the metabolic demands of the enlarged uterus, placenta, and fetus. Resting minute ventilation also rises during pregnancy, primarily with an increase in tidal volume with a constant breathing rate and pattern (7). The increased minute ventilation occurs secondary to increased CO2 production and progesterone-induced increase in chemosensitivity.
c. The gravid uterus displaces the diaphragm upward decreasing the parturient’s functional residual capacity (FRC) up to 20% at term (3,4,7). However, the closing capacity (CC) does not change. The resulting decrease in FRC/CC ratio causes faster small-airway closure, V/Q mismatch, and hypoxemia, especially in the supine position. During periods of apnea, pregnant women desaturate faster compared to nonpregnant women (8). Preoxygenation with 100% O2 for 3 to 5 minutes is recommended before induction of general anesthesia.
CLINICAL PEARL Pregnant women rapidly become hypoxic during apnea secondary to increased oxygen consumption and decreased FRC.
2. Cardiovascular
a. Pregnancy is a high cardiac output state with a 35% to 40% increase in the cardiac output seen by the end of the first trimester and plateauing to a level 50% greater than nonpregnant women in the third trimester (3,4). Cardiac output increases even more during labor and immediately postpartum. The increase in cardiac output is secondary to an increase in stroke volume (25%) and heart rate (15% to 25%) (9,10). Systemic vascular resistance decreases by 20% secondary to addition of the low-resistance placental circulation as well as the vasodilatory effects of prostacyclin, estrogen, and progesterone, resulting in an overall slight decrease in maternal blood pressure (3,4).
b. When the parturient is positioned supine, compression of the inferior vena cava and aorta by the gravid uterus can cause a significant decrease in cardiac output and uteroplacental perfusion (11). Aortocaval compression occurs as early as 20 weeks of gestation. Supine hypotension syndrome is characterized by severe hypotension and bradycardia secondary to a profound decrease in venous return in the supine position (12). When supine, pregnant women should be positioned with either left or right uterine displacement (15 to 20 degrees) using a small pillow or wedge under the contralateral hip.
CLINICAL PEARL Aortocaval compression in the supine position must be avoided in all parturients by using either left or right uterine displacement.
c. Pregnant women are more prone to pulmonary edema secondary to the normal decrease in colloid oncotic pressure that occurs during pregnancy and immediately postpartum (9). The use of tocolytic agents during fetal surgery further predisposes them to pulmonary edema.
3. Neurologic
a. The minimum alveolar concentration (MAC) of inhalational anesthetics is decreased by approximately 30% in the parturient, secondary to increased levels of progesterone and plasma endorphins (13).
b. Pregnant women are more sensitive to the effects of local anesthetics secondary to a combination of hormonal changes, decrease in CSF volume from engorgement of epidural veins and pH changes in the CSF (14–16). Increased dermatomal spread of epidurally administered local anesthetics is also frequently seen.
4. Gastrointestinal
a. Pregnant women are at higher risk of aspiration of gastric contents secondary to the effects of progesterone decreasing the tone of the lower esophageal sphincter, altered position of the stomach, and decreased gastric pH (17,18). Gastric emptying does not decrease during pregnancy, except during labor and with the administration of opioids (19). All pregnant women should receive aspiration prophylaxis, including a H2 receptor antagonist, promotility agent, and nonparticulate antacid before induction of general anesthesia. A rapid-sequence induction must be performed prior to endotracheal intubation.
CLINICAL PEARL Pregnant women are at higher risk of aspiration of gastric contents and should receive aspiration prophylaxis medications before a rapid-sequence induction of general anesthesia.
5. Renal
a. During pregnancy, renal blood flow increases by 75% and glomerular filtration rate (GFR) increases by 50% resulting in a 50% decrease in blood urea nitrogen (BUN) and creatinine levels (20,21). Hence, a normal or slightly increased BUN and creatinine level in a pregnant patient indicates poor renal function. Urea, creatinine, and uric acid clearance, all increase during pregnancy.
b. Total protein and glucose excretion in urine is increased several-fold during pregnancy secondary to changes in resorptive capacity of the proximal tubules (21).
6. Hematologic
a. Maternal plasma volume increases by 55% during pregnancy secondary to the effects of estrogen, progesterone, and placental lactogen on the renin angiotensin–aldosterone system (22,23). The red blood cell volume increases by 30% during pregnancy due to increased erythropoietin production. The plasma volume expansion exceeds the red blood cell volume increase resulting in the so-called “physiological anemia of pregnancy,” with a hematocrit in the 30% to 35% range at sea level. The physiologic hypervolemia of pregnancy allows the parturient to tolerate the blood loss during delivery with minimal hemodynamic changes.
b. Pregnancy represents a state of accelerated but compensated intravascular coagulation as evidenced by enhanced platelet activation and consumption, increase in coagulation factors (I, VII, VIII, IX, X, XII) and increased fibrinolytic activity (24).
CLINICAL PEARL Pregnancy affects every organ system with various anatomic and physiologic changes. Fetal interventions performed in early and mid-gestation might not be associated with the same degree of pregnancy changes compared to procedures in the third trimester or closer to term gestation.
B. Uteroplacental blood flow
1. Uterine blood flow increases significantly during pregnancy, rising from 50 to 100 mL per minute before pregnancy to approximately 700 to 900 mL per minute at term, constituting approximately 12% of maternal cardiac output (25). The uteroplacental circulation is a low resistance vascular bed with limited ability for autoregulation. Uterine blood flow is directly proportional to uterine perfusion pressure (difference between uterine arterial pressure and uterine venous pressure) and inversely proportional to uterine vascular resistance. Therefore, adequate uteroplacental blood flow depends on the maintenance of maternal blood pressure and normal uterine perfusion pressure (Table 33.1).
CLINICAL PEARL During fetal interventions, it is critical to maintain maternal blood pressure and uteroplacental blood flow by avoiding aortocaval compression, hypotension, and uterine contractions.
2. The effects of neuraxial anesthesia on uterine blood flow depend on multiple factors. While effective pain relief and decreased stress increase uterine blood flow, associated hypotension if present, decreases uterine blood flow (26,27).
3. Both phenylephrine and ephedrine are used clinically for the treatment of hypotension. Ephedrine crosses the placental to a greater extent than phenylephrine and stimulates metabolic processes in the fetus, resulting in lower fetal pH and base excess than phenylephrine (28). Studies comparing the two drugs in humans have not demonstrated any clinical difference in neonatal outcomes (29).
TABLE 33.1 Etiology of decreased uterine blood flow
Decreased uterine arterial pressure
• Aortocaval compression
• Hemorrhage/hypovolemic shock
• Sympathetic blockade from neuraxial anesthesia
• Hypotension from deep general anesthesia
Increased uterine venous pressure
• Venacaval compression
• Uterine contractions
• Uterotonic drugs
Increased uterine vascular resistance
• Endogenous catecholamines (pain, anxiety, stress)
• Vasopressors (phenylephrine > ephedrine)
Adapted from Ngan Kee WD. Uteroplacental blood flow. In: Chestnut DH, Wong CA, Tsen LC, et al, eds. Obstetric Anesthesia: Principles and Practice. 5th ed. Philadelphia, PA: Elsevier-Mosby; 2009:41.
4. Intravenous induction agents such as propofol and thiopental have minimal effects on uterine blood flow unless associated with hypotension (30). Animal studies in pregnant sheep have shown that the use of inhalational agents in the usual clinical doses has minimal effects on uterine blood flow, although the use of higher doses is associated with maternal hypotension and decreased uterine blood flow (31,32).
C. Placental transport
1. The placenta is a dynamic organ that brings the maternal and fetal circulation in close opposition for the transfer of gases, nutrients and wastes. Factors affecting the transport of drugs across the placenta include lipid solubility, molecular weight, protein binding, pKa, pH of fetal blood, and uterine blood flow (33). High lipid solubility and molecular size <500 daltons allow rapid transport of drugs across the placenta. Highly protein-bound drugs are also affected by the concentration of maternal and fetal proteins with only the free, unbound fraction of the drug transferring across the placenta. If the fetal pH is lower than the drug’s pKa (i.e., fetal acidemia), basic drugs such as local anesthetics and opioids become more ionized when they reach the fetal circulation. Since ionized drugs cannot easily cross the placenta, they are trapped in the fetal circulation (i.e., “ion trapping”) (33).
2. The high lipid solubility and low molecular weight of inhalational anesthetics allow rapid transport across the placenta. However, studies in fetal lamb have showed delayed equilibration, with fetal levels of isoflurane and halothane significantly lagging behind maternal levels (34,35). Fetal anesthesia during open fetal surgeries is ensured by intramuscular administration of muscle relaxant and narcotic to the fetus. Nitrous oxide also readily crosses the placenta (36).
CLINICAL PEARL Despite transplacental passage of inhalational anesthetics during open fetal surgery, delayed equilibration necessitates intramuscular administration of narcotic and muscle relaxant to the fetus.
3. Intravenous induction agents also readily cross the placenta. Despite rapid transport of thiopental across the placenta, there is wide intersubject variability in the umbilical cord blood concentrations of thiopental at delivery, secondary to large differences in volume of distribution and plasma clearance of thiopental among pregnant patients (37). Propofol also readily crosses the placenta and may have sedative effects on the neonate. Maternal administration of propofol for induction of general anesthesia during elective cesarean delivery results in lower 1- and 5-minute APGAR scores when compared to thiopental (38). The effects on the newborn depend on the induction dose and the time elapsed between induction and delivery of the fetus (39).
4. Nondepolarizing muscle relaxants and anticholinesterase agents are large, highly ionized molecules that do not readily cross the placenta (33,40). Following maternal administration of a standard intubating dose, an insignificant amount of succinylcholine crosses the placenta. At higher doses (>300 mg), a detectable amount of succinylcholine may be noted in umbilical cord blood which is not clinically significant (40,41). Even though the serum concentration of plasma cholinesterase is reduced in pregnancy, the duration of action of succinylcholine is not affected due to increased volume of distribution of the drug.
5. Opioids readily cross the placenta. Maternal administration of morphine has been shown to cause a reduction in the fetal biophysical profile with absent fetal breathing movements and nonreactive nonstress tests (42). Epidural administration of fentanyl and sufentanil for labor analgesia results in significant transplacental transfer of both these lipophilic opioids (43).
III. Fetal considerations
A. The fetus has no effective oxygen reservoir and depends on ongoing transplacental diffusion of oxygen from the maternal circulation (44). At baseline, fetal PO2 is much lower than postnatal circulation with umbilical venous PO2 reaching a maximum of 50 to 60 mm Hg. However, fetal tissue is not ischemic because of a higher fetal hemoglobin concentration (approximately 18 g/dL) and a relatively higher concentration of hemoglobin F in fetal blood (75% to 85% of total hemoglobin). Hemoglobin F has a higher affinity for oxygen than maternal blood, augmenting the transfer of oxygen from the mother to the fetus. The P50 (PO2 at which hemoglobin is 50% saturated) of fetal blood is approximately 20 mm Hg compared to 27 mm Hg in adult blood (44).
B. Fetal intravascular blood volume is approximately 100 to 110 mL per kg, with one third of it contained outside the fetal body, in the umbilical cord and placenta (44). Fetal intravascular blood volume is determined by the transport of fluids across capillaries and is primarily regulated by changes in capillary hydrostatic pressure.
C. The fetal circulatory pattern differs significantly from the adult circulation with the right and left ventricles functioning in a parallel system (44). Oxygenated blood from the placenta enters the fetus via the umbilical vein. Some of it bypasses the liver via the ductus venosus to enter the inferior vena cava. In the right atrium, most of the oxygenated blood preferentially passes across the foramen ovale, to enter the left atrium. It then flows into the left ventricle, from where it is distributed to the brain and preductal circulation, facilitating delivery of richly oxygenated blood to the organs with highest oxygen requirements, that is, brain and heart. Deoxygenated blood from the lower extremities and superior vena cava is preferentially directed into the right ventricle and pulmonary artery. The majority of this blood bypasses the high resistance pulmonary vasculature and passes through the ductus arteriosus into the descending aorta to supply the lower extremities. Blood returns to the placenta via the umbilical arteries for nutrients and gas uptake.
D. Due to the parallel flow of the fetal circulatory system, the fetal cardiac output is measured as a sum of the left and right ventricular output or combined cardiac output (CCO). Doppler echocardiographic studies estimate that the CCO ranges between 425 and 550 mL/kg/minute, with the right ventricle contributing 60% to 70% of the CCO (45).
E. The fetal myocardium has a greater proportion of noncontractile proteins, resulting in increased myocardial stiffness and impaired relaxation (46). It functions close to the upper limit of the Frank–Starling curve, with increases in preload resulting in only minimal increases in stroke volume. Fetal cardiac output is more sensitive to changes in heart rate, with bradycardia resulting in decreased ventricular output. Additionally, the constraint of the pericardium and chest wall–lung combination is a major factor limiting the filing of the left ventricle (47). At birth, aeration of the lungs and clearance of lung fluid relieves this constraint, improving left ventricular preload and stroke volume.
F. Fetal hypoxia causes aortic chemoreceptor stimulation resulting in bradycardia and decreased cardiac output. The decrease in cardiac output is exacerbated in the presence of fetal acidemia and increased after load from umbilical cord compression (48). Fetal hypoxia also redistributes blood flow from the peripheral circulation to the brain, heart and placenta (“central sparing effect”). Fetal heart rate (FHR) monitoring using Doppler ultrasonography is commonly utilized during fetal interventions. Fetal bradycardia is a reliable indicator of fetal distress and umbilical cord compression must be ruled out.
CLINICAL PEARL Fetal cardiac output is more sensitive to changes in fetal heart rate and fetal bradycardia is a sign of fetal distress.
G. Fetal hemostatic mechanisms are immature and gradually evolve throughout pregnancy. The fetus produces coagulation factors independently of the mother. Direct umbilical vein sampling of fetuses at different gestations has shown that even though fetuses at 30 to 38 weeks gestational age have higher levels of coagulation activators and inhibitors compared to younger fetuses, their levels are significantly lower than in term newborns (49).
H. Perception of a painful stimulus by the fetus is a highly controversial topic. Needling of the fetal intrahepatic vein during intrauterine blood transfusions has been shown to be associated with an increase in fetal plasma cortisol and β-endorphin levels and decreases in middle cerebral artery (MCA) pulsatility index (50). Administration of fentanyl has been shown to blunt this stress response (51). However, withdrawal reflexes, decreased fetal MCA pulsatility index, and increased neuroendocrine stress hormone levels do not constitute evidence of fetal pain as they can be elicited by non-noxious stimuli and can occur without conscious cortical processing (52). Fetal awareness of noxious stimulus requires functional thalamocortical connections which begin appearing between 23 and 30 weeks gestational age (52). Electroencephalography patterns representing wakefulness appear around 30 weeks’ gestational age.
I. The fetus is more sensitive to inhalational anesthetics; animal studies show the MAC of halothane and isoflurane in fetal lambs is reduced compared to pregnant ewes (53,54).
J. The fetal lungs actively secrete lung fluid, which distends the lungs and allows normal lung development. Excess lung fluid escapes through the trachea and enters the oropharynx, after which it is either swallowed or enters the amniotic cavity. Excessive and continuous drainage of lung liquid results in small hypoplastic lungs which are deficient in alveoli. In contrast, ligation of the trachea results in large hyperplastic lungs with well-defined future alveoli (55).
K. Fetal temperature is closely linked to maternal temperature. Secondary to increased oxygen consumption and metabolism, the fetus produces more heat that is dissipated to the mother via the placenta, until a relatively constant fetal-to-maternal temperature gradient of 0.5°C is established (44). After birth, loss of this “heat clamp” and new evaporative losses require the newborn to increase heat production via shivering and nonshivering thermogenesis. A fetus exposed during open fetal surgery is not capable of this increased heat production and is prone to hypothermia.
IV. Fetal malformations treated by fetal interventions. Advances in prenatal imaging and surgical techniques have resulted in a wide variety of fetal interventions being performed for different fetal malformations (Table 33.2).
A. Hydrops fetalis
1. Hydrops fetalis is a serious fetal condition characterized by abnormal fluid collection in at least two different fetal compartments including pericardial effusion, pleural effusion, ascites, subcutaneous edema, polyhydramnios and placentomegaly (56,57). There are two types of hydrops: immune and nonimmune, depending on the presence or absence of maternal antibodies against fetal red cell antigens.
2. Immune hydrops is most commonly the result of Rh isoimmunization, where maternal IgG antibodies against fetal red cell antigens cross the placenta, causing hemolysis of fetal red cells and fetal anemia (56). The incidence of immune hydrops secondary to Rh isoimmunization has drastically reduced after the widespread use of immunization with Rh immunoglobulin. Rarely, isoimmunization due to non-Rh and non-ABO incompatibility can occur secondary to blood transfusions.
3. Fetal interventions for immune hydrops include percutaneous umbilical blood sampling (PUBS) and intrauterine fetal blood transfusion (56). Typically, a 20-gauge spinal needle is used to cannulate the umbilical vein under direct ultrasound guidance and obtain the fetal blood sample. Freshly washed, irradiated, high hematocrit (approximately 80%), group O packed red cells are then transfused into the fetus to reach a target hematocrit, which should not be >25% or greater than four times the initial hematocrit to avoid fluid overload in the fetus (56).
4. Nonimmune hydrops fetalis (NIHF) is a heterogenous disorder caused by a variety of underlying pathologic processes including cardiovascular disorders, chromosomal abnormalities and hematologic disorders (57–59) (Table 33.3). Cardiovascular disorders and arrhythmias frequently cause hydrops secondary to congestive heart failure and are associated with a poor prognosis. In hematologic disorders, severe fetal anemia leads to the development of high-output cardiac failure. Thoracic masses such as congenital pulmonary airway malformations and bronchopulmonary sequestration cause hydrops by increasing intrathoracic pressure and decreasing venous return.
TABLE 33.3 Common causes of nonimmune hydrops fetalis
Cardiac malformations | Atrioventricular canal defect, hypoplastic left and right ventricle, atrial septal defect, ventricular septal defect, tetralogy of Fallot, Ebstein anomaly, truncus arteriosus, transposition of great vessels, aortic stenosis, pulmonary stenosis, premature closure of ductus arteriosus, and foramen ovale. |
Cardiac arrhythmias | Supraventricular tachycardia, atrial flutter, heart block, Wolff–Parkinson–White syndrome |
Chromosomal abnormalities | Trisomy 18 and 21, Turner syndrome, triploidy |
Thoracic abnormalities | Congenital pulmonary airway malformations, bronchopulmonary sequestration, diaphragmatic hernia, bronchogenic cyst |
Hematologic abnormalities | Alpha-thalasesemia, parvovirus B19 infection, twin-to-twin transfusion syndrome, G6PD deficiency |
Genetic syndromes | Arthrogryposis, Noonan syndrome, tuberous sclerosis |
Infectious disease | Cytomegalovirus, parvovirus B19, syphilis, toxoplasmosis, herpes simplex, varicella |
Vascular disorders | Arteriovenous malformations, sacrococcygeal teratoma, placental chorioangioma, vascular malformations |
Skeletal dysplasia | Achondroplasia, achondrogenesis, osteogenesis imperfecta |
Adapted from Bianchi DW, Crombleholme TM, D’Alton ME, et al, eds. Nonimmune hydrops. In: Fetology: Diagnosis and Management of the Fetal Patient. 2nd ed. New York, NY: The McGraw-Hill; 2010:895.
5. Following the diagnosis of hydrops, maternal blood tests should include indirect Coombs antibody screen, maternal blood type, complete blood cell count, hemoglobin electrophoresis, and evaluation for infectious diseases like syphilis, parvovirus B19, toxoplasmosis, cytomegalovirus, and herpes simplex (57). In addition, a detailed fetal sonography and echocardiography must be performed to rule out structural anomalies.
6. Mirror syndrome, also referred to as Ballantyne syndrome or pseudotoxemia, refers to a state of generalized maternal edema that mirrors the hydropic fetus and occurs only in the presence of placentomegaly (60). Clinical features include maternal weight gain, generalized edema, hypertension, anemia, hemodilution, and pulmonary edema. Treatment of the cause of hydrops, if it can be readily reversed, or delivery of the fetus usually results in resolution of maternal symptoms over a period of 48 to 72 hours.
B. Twin-to-twin transfusion syndrome (TTTS)
1. TTTS is a serious complication seen in 5% to 15% of monochorionic twin pregnancies and is associated with a poor prognosis (60% to 100% perinatal mortality of both twins) if left untreated (61,62). TTTS is a progressive disease characterized by placental intertwin vascular anastomoses and unequal placental sharing resulting in the “recipient” twin becoming hyperdynamic and hypervolemic, while the “donor” twin becomes hypodynamic and hypovolemic (Fig. 33.1). As the disease progresses, the “donor” twin develops oliguria, oligohydramnios, and growth restriction, while the “recipient” twin develops worsening hypertensive cardiomyopathy with unilateral or bilateral ventricular hypertrophy, ventricular dilation, increased cardiothoracic ratio, and atrioventricular valve regurgitation (61,62). There is mounting evidence to suggest that the pathophysiology of TTTS is more complex than mere volume shifts and is characterized by transport of vasoactive mediators such as renin and endothelin-1, resulting in hypertensive cardiomyopathy in the recipient twin.
2. TTTS is diagnosed based on ultrasound findings of (i) monochorionic twins (ii) same sex (iii) discrepancy in amniotic sacs with polyhydramnios of one twin and oligohydramnios of the other twin (iv) discrepancy in size of umbilical cords (v) cardiac dysfunction in the polyhydramniotic twin (vi) abnormal Doppler waveforms in umbilical artery, umbilical vein, ductus venosus or middle cerebral artery and (vii) significant growth discordance (>20%) (61). Several staging systems have been described based on ultrasound findings and fetal echocardiographic changes.
FIGURE 33.1 Twin-to-twin transfusion syndrome. (Reprinted with permission from Timothy M Crombleholme, MD, Colorado Institute for Maternal & Fetal Health, Aurora, CO.)

Full access? Get Clinical Tree
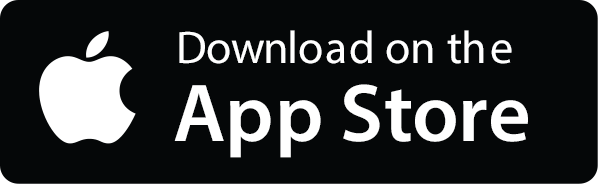
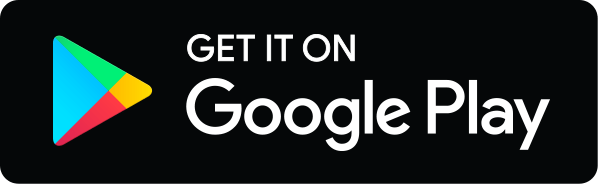