Procedure or positioning
Nerve(s) compressed
Symptoms
Arthroscopic surgery of the elbow [3]
Posterior interosseous nerve
Wrist drop
Abduction, dorsal extension, and external rotation of the arm [4]
Brachial plexus
Variable
Elbow abduction to 90*with a sagging armboard [4]
Brachial plexus
Variable
Trendelenburg positioning with shoulder braces [4]
Brachial plexus
Variable
Open heart surgery [5]
Lower brachial plexus
Ulnar nerve distribution numbness and weakness with finger extensor weakness
Arm abduction without elbow pad [4]
Ulnar
Numbness in digits III–V, weakness in ulnar innervated muscles
Lithotomy [6]
Peroneal nerve
Foot drop
Lithotomy [6]
Femoral nerve
Quadriceps weakness, loss of patellar reflex
Semilateral position [4]
Sciatic
Peroneal and tibial innervated nerves as well as hamstring muscles
Anesthetic Events
The details of anesthetic management should be thoroughly reviewed, especially if portions of the anesthetic care were delivered by other anesthetists. Drug choice, dose, and last time of administration are of obvious importance. Long duration of blockade and high concentration of agents probably increases the risk of neural complications. The development of hypotension or hypertension should be identified, since these can be a source of CNS injury. Examples include (1) malignant hypertension-induced posterior reversible encephalopathy syndrome [7], the lesions of which are typically occipital but can occur elsewhere in the brain; (2) hypotension-induced spinal cord infarct, especially in the setting of thoracoabdominal aortic interventions [8]; and (3) hypotension-associated cerebral watershed stroke leading to a “Man in the Barrel Syndrome ” characterized by bilateral arm weakness or paralysis with intact leg function [9].
Blood return through the needle at the time of performing the blockade, although sometimes intended, indicates the possibility of hematoma as mechanism of neural compromise. Undesired entry into the subarachnoid space may be evident only after doses suitable for epidural anesthesia have been injected, increasing the risk of local anesthetic toxicity. Attempted aspiration of cerebrospinal fluid (CSF) before each epidural injection should be a standard maneuver. Observation of a gradual development and expected sequence of blockade and hemodynamic changes offer some reassurance that the proper site of drug deposition has been achieved. Conversely, maldistribution will not only lead to possible toxic results but also fail to produce desired anesthetic effects. Examples include accumulation of hyperbaric subarachnoid lidocaine in the terminal dural sac or injection through a catheter intended for the epidural space but placed in or adjacent to a spinal nerve in the intervertebral foramen.
The presence of paresthesia or pain during needle placement may herald mechanical injury or injection within a nerve fascicle, increasing the likelihood of mechanical or chemical injury to individual nerve fibers. Because sedation or general anesthesia precludes the observation of pain and paresthesias, the exact timing of needle placement and injections relative to systemic medication may be critical, and the depth of sedation or presence of general anesthesia at the time of neural blockade should be noted. Injection into the spinal cord is unlikely to take place in a patient who is awake and can report the accompanying intense sensory event. However, injection into the cord, or even into a peripheral nerve with longitudinal passage of solution into the cord [10], may go unrecognized in an unresponsive patient, resulting in catastrophic myelopathy. Sudden hypotension may accompany the cord injury. Such events are most likely to occur during thoracic epidural injections or subarachnoid injections in obese patients in whom the surface landmarks mistakenly lead to high lumbar needle placement.
Development of Neurologic Dysfunction
The sequence and timing of the onset of symptoms related to the nerve injury should be determined to provide clues to the etiology and best treatment. The onset of pain, weakness, sensory deficit, and changes in sphincter control may be obtained from the patient, although sedation in the early postoperative period may compromise recollection of the details. Nurses are important sources of information, as are family members if the patient has already been discharged home. The ideal is frequent and complete postoperative visits by the anesthetist.
Physical Examination
General Examination
The general examination may yield clues to the etiology of a suspected postanesthetic nerve injury. For example, a local hematoma or ecchymosis will draw attention to the possibility of a focal compressive or traumatic nerve injury. Even in the absence of such superficial signs, focal nerve compression by a deeper limb or spinal hematoma should be considered if other manifestations of a coagulopathy are identified (e.g., bleeding at multiple sites). The presence of distal vascular insufficiency raises concern for a monomelic ischemic neuropathy. Abnormal function of the gastrointestinal or urologic system is a “red flag” for possible spinal cord injury or cauda equina syndrome. A postprocedural abscess should be excluded in the setting of fever or local erythema, tenderness, induration, fluctuance, or gross purulence. The presence of a fever, especially in the absence of another obvious source, should raise the question of a deep infection such as in the epidural space, although fevers are often absent. Tenderness and spasm of the muscles may result from bleeding, infection, or neural injury. A distended bladder may indicate a dysfunctional sphincter.
Neurologic Examination
A detailed evaluation is essential for identifying signs of dysfunction, monitoring for progression, and tracking recovery from regional anesthesia. In patients who have seen a neurologist in the past for an established diagnosis or evaluation of neurologic complaints, the previous neurologic examination should be employed as baseline against which the current examination is compared to objectively differentiate new from old findings. The neurologic examination should encompass all of its usual subcomponents, including cranial nerves and mental status, as abnormalities in these realms will redirect attention away from the site of regional anesthesia to more cephalad areas of the CNS. Additionally, examination of the muscles, skin, and hair in the extremities can help to determine if the patient has had a previous neurologic injury in the acutely symptomatic region. Muscle atrophy , cutaneous atrophy , loss of hair , anhidrosis , hyperhidrosis , scarring , or ulcers in a distribution similar to the current complaints may signify the presence of a preexisting, chronic neurogenic process. In patients with unilateral symptoms, examination of the contralateral side of the body should be done and may provide additional clues to the location and extent of injury.
When evaluating a potential neurologic injury, diagnosis and treatment hinge on first localizing the lesion in the neurologic system. The process of localization commences with the differentiation between central (brain and spinal cord ) and peripheral (peripheral nerves, muscles, and neuromuscular junctions) lesions. In the acute setting, both central and peripheral injuries tend to suppress stretch reflexes. Moreover, such lower motor neuron signs as muscle atrophy and fasciculations are generally delayed in appearance. Hence, shortly after the procedure and the onset of new neurological deficits, careful mapping of the patterns of motor and sensory abnormalities, combined with the presence versus absence of Babinski signs , is critical to the central versus peripheral differentiation. Once this distinction is made, further attention to the precise nature and distribution of the motor and sensory deficits permits the region of injury to be localized to specific areas of the central nervous system (CNS) , nerve roots , nerve plexuses , peripheral nerves , and/or muscles. After the site of injury is determined, a refined etiologic differential diagnosis can be designed and investigated with focused diagnostic testing. (With the advent of the smart phone, localization can now be aided by applications that provide a provisional localization based on input data comprising regions of affected versus intact strengths.) [11].
Mental Status/Cranial Nerves
Abnormal mentation (e.g., confusion or aphasia) or cranial nerves should prompt concern for an intracranial rather than peripheral process. Postoperative altered mental status has a broad differential diagnosis but most commonly results from metabolic, toxic, and systemic infectious causes. When focal neurologic deficits are accompanied by altered mentation, a cerebrovascular event is more likely than a peripheral regional injury. However, two (or more) processes may be concurrent.
Motor Examination
To evaluate any neurologic complaint, manual muscle testing should be performed. In manual muscle testing, the primary action of the tested muscle is identified and its action isolated, with the understanding that confounding by other muscles with similar actions is inevitable. As such, grading of strengths should be assigned to the action (e.g., elbow flexors) rather than the muscle (e.g., biceps brachii). Once the active joint and muscle are isolated, the joint is stabilized and the patient is instructed to perform a maximal isometric contraction against the examiner’s resistance. Strength is then graded on a standard 0–5 scale originally defined by the United Kingdom’s Medical Research Council (MRC) as shown in Table 7.2. While other scales exist, this is the most commonly used method of grading muscle strengths [12]. For regional injuries that appear to involve a single extremity, it is important to test not only ipsilateral but also contralateral muscles. The sensitivity of individual muscle strength assignments is enhanced by interside comparisons. Moreover, a bilateral examination may reveal unexpected deficits in the “unaffected” side, thereby altering the neurological localization .
Table 7.2
UK medical research council manual muscle grading scale
Grade | Exam finding |
---|---|
5 | Unable to overcome a patient’s antigravity positioning with an examiner’s muscle of similar strength |
4+ | Able to overcome with much effort a patient’s antigravity positioning with an examiner’s muscle of similar strength |
4 | Able to overcome with moderate effort a patient’s antigravity positioning with an examiner’s muscle of similar strength |
4− | Able to overcome with minimal effort a patient’s antigravity positioning with an examiner’s muscle of similar strength |
3 | Patient has full range of motion against gravity but cannot exert any power against the examiner |
2 | Full range of motion when gravity eliminated (action of muscle must be observed in plane perpendicular to gravity) |
1 | Contraction of muscle felt or seen without movement of joint |
0 | No movement of muscle |
In patients who complain of weakness despite intact manual muscle testing , other examination techniques may detect more subtle weakness. Examples include assessment of fine finger movements, such as those required with repetitive tapping or buttoning tasks, observation for a pronator drift of one arm with both upper arms forward flexed and eyes closed, and rolling of the forearms around each other (also known as “disco dancing ”). Of note, asymmetries in arm rolling and rapid finger tapping amplitude and rate can ensue not only from subtle weakness but also handedness, bradykinesia, or ataxia. Moreover, findings in these tasks are more difficult to localize than those revealed in manual muscle testing because multiple muscles contribute to these complex movements .
Once the distribution of motor deficits has been determined by muscle strength testing, the resulting pattern should be analyzed for lesion localization. If true injury has occurred, the pattern of weakness should conform to an upper motor neuron, spinal nerve root, plexus, or peripheral nerve process. Typical motor deficits observed in patients with cervical or lumbosacral nerve root injuries are itemized in Table 7.3.
Table 7.3
Clinical findings in nerve root lesions
Root | Sensory loss | Reflex arc | Motor deficits |
---|---|---|---|
C5 | Lateral shoulder | Biceps | Shoulder abduction/external rotation, elbow flexion |
C6 | Lateral forearm, thumb | Biceps, brachioradialis | Supination, elbow flexion, pronation, wrist extension |
C7 | Dorsal arm and forearm, middle finger | Triceps | Elbow extension, wrist flexion/extension |
C8 | Medial hand, small finger | Finger flexors | Finger extension, abduction, and flexion |
T1 | Anteromedial forearm | – | Thumb abduction |
L2–3 | Anterolateral thigh (L2), distal medial thigh/knee (L3) | Adductor, patellar | Hip flexion/adduction |
L4 | Anteromedial lower leg | Patellar | Knee extension/adduction, hip flexion |
L5 | Lateral lower leg, dorsal foot, great toe | Medial hamstrings | Ankle dorsiflexion, eversion, and inversion; great toe extension; hip abduction |
S1 | Posterior thigh and lower leg, lateral foot, sole | Achilles | Ankle plantar flexion, hip extension |
Sensory Examination
The sensory examination involves evaluation of multiple sensory modalities (pain, cold, heat, light touch, vibration, and proprioception), but not all modalities need to be tested in every patient. Testing of nociception with a truly sharp object (i.e., pin) and light touch with a finger or cotton wisp should be routinely performed. Similarly sized small myelinated and unmyelinated fiber types convey both temperature and pain sensation to the CNS. Within the spinal cord, primary afferents subserving pain and temperature cross over at their level of entry into the spine and then jointly ascend in the contralateral spinothalamic tract. Therefore, evaluation of cold and hot sensation need not be undertaken in every patient already evaluated for pain sensation, but thermal sensation testing may help to confirm a region of abnormal sensation suggested by the pinprick examination.
Vibration and proprioception sensation should also be examined. Unlike pain and temperature, these sensory modalities are carried by larger myelinated fibers, which ascend ipsilaterally (no cross-over) in the posterior part of the spinal cord (posterior columns). Loss of dorsal column function may lead to an abnormal “sensory ataxic” gait , as the patient loses their ability to sense the placement of their lower extremities in space. Spinal cord infarcts are usually caused by occlusion of the anterior spinal artery, which supplies the spinothalamic tracts but not the dorsal columns. Hence, vibration and proprioception are usually spared in ischemic spinal cord injuries.
In all modalities, testing should not only be distal but also delineate possible peripheral nerve, brachial or lumbosacral plexus, nerve root, and spinal sensory tract lesions. Key dermatomes (areas of sensory loss resulting from nerve root lesions) are detailed in Table 7.3.
Reflexes
Muscle stretch reflexes should be tested in all extremities in all patients. Normal reflexes range from 1+ (hypoactive) to 2+ (normal) to 3+ (brisk) depending on age, level of consciousness, medications, and past medical history. Reflexes are abnormal if they are asymmetric, absent (“0”), clonic (“4+”), or incongruent with reflexes in other extremities. Crossed adductor reflexes are also abnormal after a few months of age. Localization can be assisted by understanding the pathway of a reflex arc. Table 7.3 includes commonly tested reflexes and the nerve roots through which they are mediated.
Coordination/Gait
Coordination is dependent not only on CNS control but also intact peripheral motor and sensory function. Patients with peripheral weakness or large fiber sensory loss can be as clumsy and “ataxic” as those with cerebellar pathway lesions. Similarly, tremors are centrally generated but modified by peripheral sensorimotor function . As another confounding factor, objective weakness of the extremities can produce “tremulous,” impersistent movements mimicking tremors and cerebellar or sensory ataxia. Retesting with the involved joint isolated from gravity (e.g., testing finger–nose–finger with the elbow supported) sometimes clarifies the source of the impairment. If the ataxia and tremor then resolve, the impersistent, clumsy movements are probably due to weakness rather than an intracranial process.
In patients with complaints of leg weakness or disturbed ambulation, evaluation of gait is mandatory. The patient should be observed walking down a long hallway. Gait is assessed with respect to multiple parameters including rate, fluidity, stability, symmetry, step height, stride length, base, arm swing, truncal posture, and extraneous movements. There are characteristic features of abnormal gait due to peripheral weakness (e.g., foot drop leading to steppage), sensory ataxia, cerebellar ataxia, spasticity, Parkinsonism, truncal weakness, hyperkinetic movement disorders, and higher level cerebral dysfunction that are beyond the scope of this chapter [13]. Toe and heel walking may reveal mild posterior and anterior distal leg weakness occult to manual muscle testing. Finally, an ataxic or Parkinsonian gait may be misconstrued by some patients and providers as resulting from leg weakness.
Electrodiagnostic Evaluation
Injuries to peripheral nerves are routinely evaluated by electrodiagnostic studies that comprise electromyography (EMG) and nerve conduction studies (NCS) . These investigations assess the electrophysiological function of the larger diameter motor and sensory fibers, which is generally sufficient for regional anesthesia-related nerve injuries, almost all of which involve larger nerves to a greater or lesser extent. For evaluation of those rare anesthesia-induced injuries restricted to small cutaneous nerve fibers alone, autonomic nervous system studies such as the quantitative sudomotor axon reflex test (QSART) and thermoregulatory sweat test (TST) can be performed [14].
The goals of electrodiagnostic testing in the setting of a regional anesthesia-related nerve injury are to (1) confirm the presence of a neurogenic lesion; (2) anatomically localize the lesion; (3) characterize the lesion with respect to attributes of diagnostic and prognostic significance, such as the primarily axonal versus demyelinating nature of the injury; and (4) monitor the evolution and progression of the lesion, with the understanding that some NCS and EMG markers of nerve injury are delayed in onset. Specific pathogenic processes tend to exhibit preferential types of injury. For example, chronic nerve compression usually produces demyelination/remyelination whereas direct trauma (e.g., intrafascicular injections), most neurotoxic drugs, and ischemic insults cause predominantly axonal damage. Autoimmune inflammatory nerve diseases can be either axonal or demyelinating depending on the target of the immune attack.
Nerve Conductions
Motor Conductions
Motor nerve conductions are performed by stimulating a motor nerve at two separate locations along its course and recording from a surface electrode placed over the belly of a muscle supplied by that nerve. The amplitude of the evoked compound muscle action potential (CMAP) , distal latency (time from the distal stimulation to the onset of the corresponding motor response), and conduction velocity between the two stimulation sites are then calculated (Fig. 7.1). Based on comparisons to normative data, these values are then used to determine if the motor NCS is normal or shows evidence of a demyelinating or axonal lesion. As a general rule, motor NCS in axonal neuropathies (with intact myelination) exhibit reduced CMAP amplitudes with preserved conduction velocities and distal latencies, whereas demyelinating neuropathies feature slow nerve conductions, prolonged distal latencies, and normal or relatively normal CMAP amplitudes. Focal demyelination can also block conduction in some (partial) or all (complete) axons at the affected site despite the presence of structurally preserved axons. Similar to axon loss, motor conduction block produces clinical weakness. In a motor NCS performed on a nerve affected by a partial demyelinative conduction block, the CMAP evoked by stimulation above the site of block has significantly smaller amplitude than that evoked by stimulation below the block (Fig. 7.2). If the block is complete, no CMAP is evoked with proximal stimulation. A partial motor conduction block is the hallmark of an acquired demyelinating neuropathy.
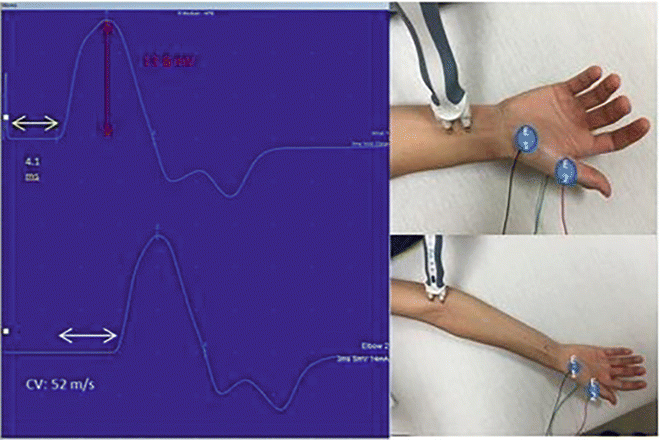
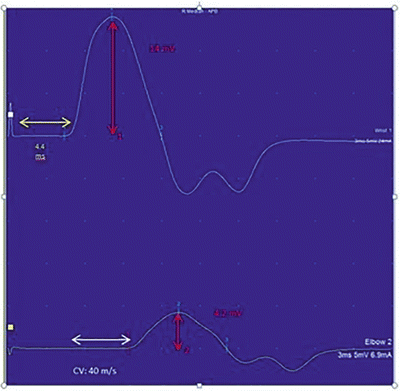
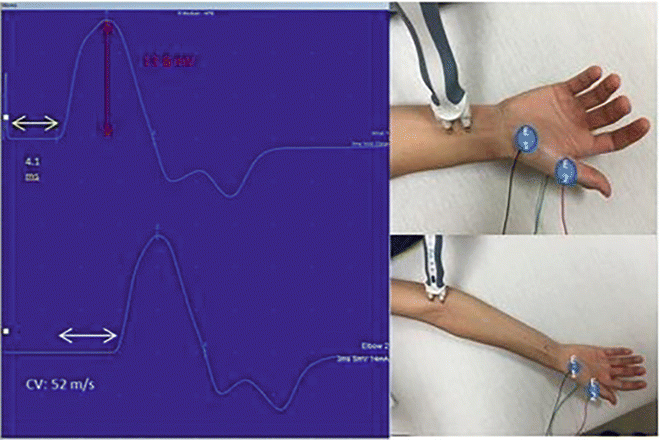
Fig. 7.1
Median nerve motor conductions . The CMAPs are on the left with placements of the distal stimulator (above right) and proximal (below right) shown. The yellow arrow highlights the onset latency, the white arrow highlights the conduction velocity (CV), and the red highlights the amplitude. E1 is the recording electrode and E2 is the reference electrode. The ground electrode is not visible on the posterior hand
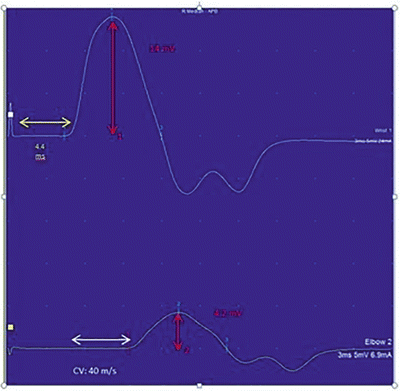
Fig. 7.2
Conduction block of the median nerve . In this patient, there is demyelination between the distal and proximal stimulation sites resulting in a drop in amplitude and conduction velocity. The superior waveform is the distal recording and inferior recording is from a proximal site. The yellow arrow highlights the onset latency, the white arrow highlights the conduction velocity (CV), and the red highlights the amplitude. Please note the differences between amplitude 1 (distal stimulation) and amplitude 2 (proximal stimulation)
Late Responses
A suprathreshold motor nerve stimulation evokes both an orthodromically (proximal to distal) and antidromically (distal to proximal) conducted neural impulse. The routine motor NCS depends on the orthodromic response. The antidromic impulse depolarizes the anterior horn cells of the constituent motor axons. If one or more of these cells is depolarized to or above its threshold, a recurrent discharge is generated that travels back down the nerve orthodromically resulting in “late” reactivation of the muscle, the F-wave response . In a single stimulation, only a small subset of motor neurons generates an F-wave. With multiple stimulations, consecutive F-waves are usually generated by different motor neurons, resulting in F-waves of varying latency and morphology (Fig. 7.3). If, on the other hand, the large Ia afferent sensory fibers are preferentially activated, typically by a lower intensity, longer duration stimulus, the evoked antidromically conducted impulse will synapse with and depolarize a pool of anterior horn cells in the spinal cord. These monosynaptic connections result in reflex activation of an orthodromically conducted motor impulse, which is recorded from the innervated muscle as the H-reflex. Unlike an F-wave , the latency of an H-reflex does not vary between stimulations, but its amplitude increases with progressively higher stimulus intensities until reaching a maximum. Then, with still higher stimulus intensities, the H-reflex decreases in amplitude and eventually disappears (Fig. 7.4). Whereas F-waves can be generated from all motor nerves and recorded from all muscles, H-reflexes are only consistently recorded from the soleus muscle after stimulation of the tibial nerve and more inconsistently recorded from the flexor carpi radialis muscle after stimulation of the median nerve.
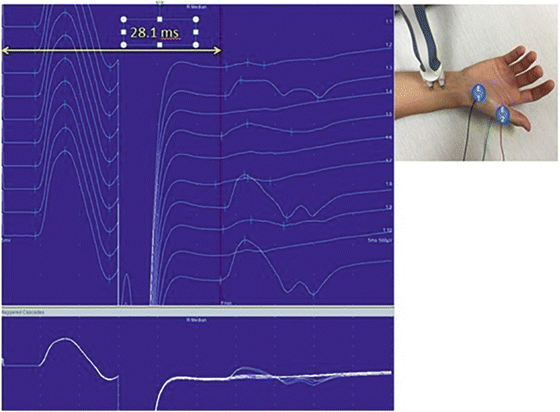
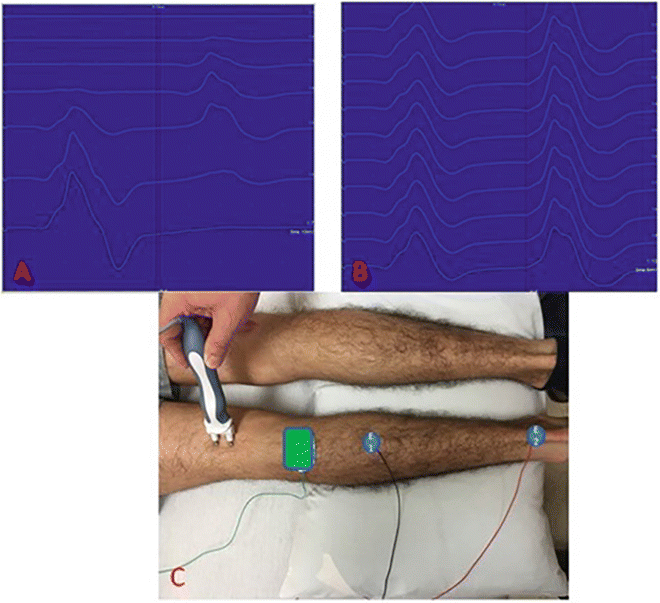
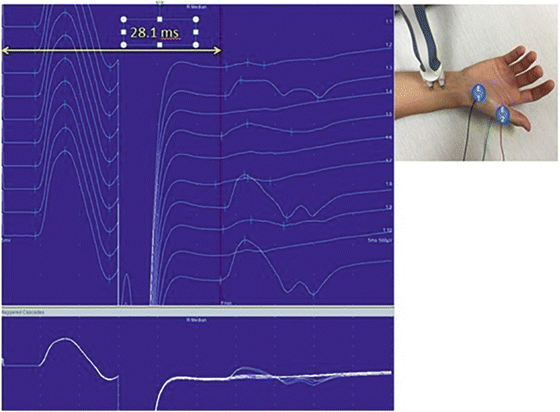
Fig. 7.3
F-Waves . The F-waves are located to the right of the red line. Please note the variable morphologies and latencies of these waveforms which indicate different motor neurons involved in each stimulation. The most common parameter measured is the minimum F-wave latency (yellow line)
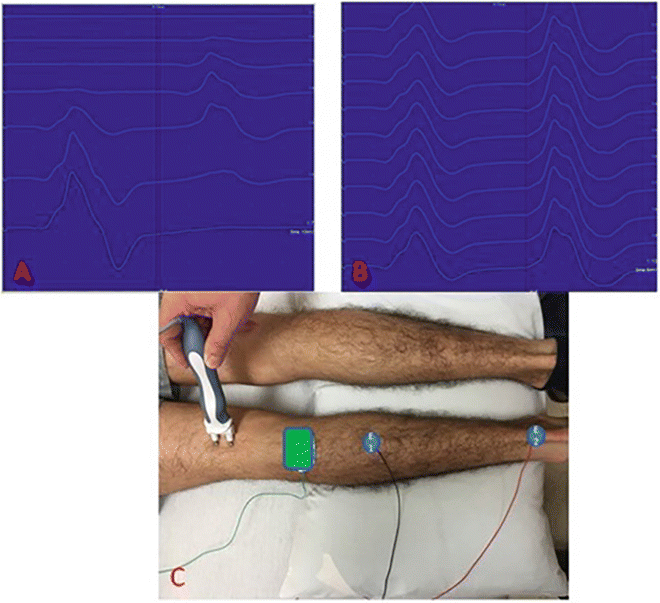
Fig. 7.4
Tibial H-reflex . The H-reflex is seen to the right of the red line in (a, b); the CMAP is to the left. From top to bottom, (a) shows sequential increasing levels of stimulation; the H-reflex gradually increases in amplitude and then decreases in amplitude as stimulation increases. (b) Shows a series of reflexes at unchanged levels of stimulus intensity; notice the uniform morphology and latency morphology (different from F-waves). (c) Shows montage with stimulator, ground (green), recording electrode (E1) and reference electrode (E2)
Clinically, F-waves and H-reflexes are used to evaluate the proximal portions of peripheral nerves not amenable to standard motor NCS. Whereas, prolonged F-wave and H-reflex latencies generally occur in demyelinating lesions, absent F-waves and H-reflexes can result from both axonal and severe demyelinating insults. F-waves that “repeat,” i.e., recur with the same latency and waveform morphology suggest a dropout of the original number of anterior horn cells or their motor axons. Focal or multifocal demyelinating lesions frequently produce variable effects on different nerve fibers in the same motor nerve, resulting in an increased spectrum of conduction velocities within individual motor fibers and hence an increased range of F-wave latencies.
Sensory Conductions
A sensory nerve conduction study is performed by stimulating a sensory nerve at one or more sites along its course and recording from a surface electrode placed over the same nerve distal or proximal to the stimulation site. Sensory conductions can be performed either antidromically or orthodromically. The evoked sensory nerve action potential (SNAP) is then evaluated with respect to amplitude, onset latency (time from stimulus to onset of the evoked potential), peak latency (time from stimulus to peak amplitude of the evoked potential), and conduction velocity (Fig. 7.5).
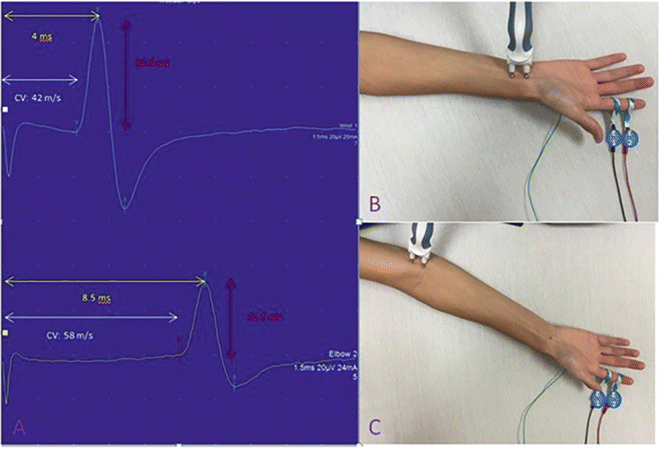
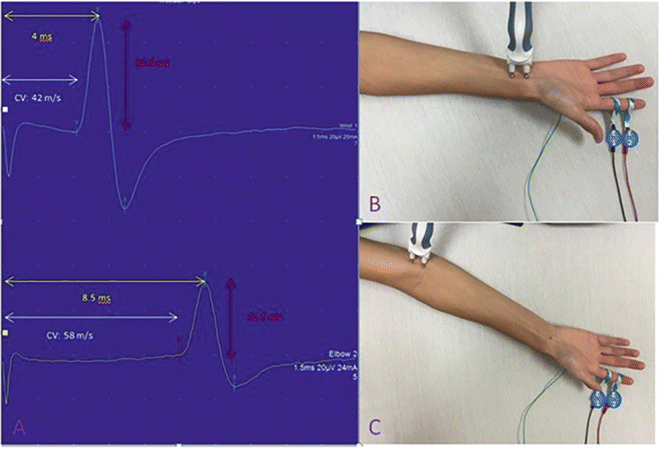
Fig. 7.5
Median sensory nerve conduction . (a) Shows the wave forms of distal (upper) and proximal (lower) waveforms. The yellow arrow highlights the peak latency, the white arrow highlights the conduction velocity (CV), and the red highlights the amplitude. The peak latency is measured from the stimulus to the peak of the wave whereas the time for the conduction velocity is measured from the stimulus to the onset of the wave (onset latency). This is an example of a normal median sensory conduction study. In (b, c) E1 is the recording electrode, E2 is the reference electrode and the ground electrode is on the posterior hand not visible in these photographs
Sensory responses are generally much smaller than motor responses. As such, sensory NCS are more technically challenging and produce more variable results than motor NCS. Similar to motor studies, SNAPs in axonal neuropathies are reduced in amplitude or absent with normal or only mildly abnormal latencies and conduction velocities. Sensory responses can be slowed in demyelinating neuropathies, but they more commonly lose amplitude because of the increased range of individual nerve fiber conduction velocities. This widened spectrum of velocities results in decreased synchrony and increased phase cancellation of the waves generated by single fibers at the site of the recording electrode. Peripherally directed sensory nerve axons originate from cell bodies of primary sensory neurons located in the dorsal root ganglia. They only degenerate with corresponding loss of SNAP amplitude when lesions damage the dorsal root ganglion or the sensory axons themselves, i.e., neuropathies or plexopathies. On the other hand, peripheral sensory nerves and thus SNAPs are preserved in most radiculopathies, in which centrally directed sensory axons in the dorsal root proximal to the dorsal root ganglion are primarily affected.
Limitations of Nerve Conduction Studies
There are some limitations to NCS. First, the nerve must be readily accessible to superficial stimulation with an equally accessible recording zone. This precludes NCS of most proximal nerves and plexus structures. Spinal nerve roots can be stimulated percutaneously with a needle electrode, but root stimulation is painful, technically difficult, and generally unreliable. Second, NCS only evaluate large sensory and motor fibers, so any process that is restricted to small-diameter nerve fibers may remain undetected with standard NCS. Third, sensory conductions can be technically difficult because of the inherently low amplitude of the evoked responses, especially in patients with extra tissue between the recording site and nerve (obesity and peripheral edema). Their performance requires utmost attention to proper technique to ensure the presence of a reproducible response. Undesired motor responses are not infrequently mistakenly identified as sensory responses when the SNAP is truly absent. Fourth, small cutaneous nerves—whether proximal or distal—cannot be reliably studied due to their inherently small surface recorded potentials (e.g., medial femoral cutaneous nerve). Fifth, the electrodiagnostic consultant needs to be attuned to the possibility of anomalous innervation pathways, e.g., Martin–Gruber (median-to-ulnar) anastomosis in the forearm, accessory peroneal branch in the lower leg, and aberrant course of the superficial peroneal sensory nerve. Sixth, CMAP amplitudes are exquisitely dependent on proper placement of the recording electrode over the “motor point” of the muscle, since the amplitude can change significantly with even minor relocations of this electrode. Seventh, preexisting conditions always need to be considered, since preexisting polyneuropathies and nerve entrapments are common and may confound interpretation of a postinjury NCS.
Another salient limitation is the delayed onset of NCS changes in response to acute axon-loss lesions. In traumatic nerve lesions resulting in Wallerian degeneration of the transected axons, which includes most needle injuries, the portion of the axon distal to the injury remains structurally intact and capable of transmitting a distally directed nerve impulse for the next 2–3 days prior to undergoing Wallerian degeneration (which will last up to 1 week). As such, CMAP and SNAP amplitudes do not begin to decrease until 2–3 days after the injury. CMAP amplitudes do not reach their nadir until 9 days after the insult. Sensory nerves degenerate at a slightly slower rate than motor nerves; accordingly, SNAP amplitudes do not achieve their nadir until 10–11 days postinjury [15]. Hence, NCS performed immediately after the injury serve as a preinjury baseline against which a follow-up study performed after 12 days can be compared to capture the true injury-related effects on the NCS.
There are also several practical limitations to the clinical utility of late responses. F-waves and H-reflexes are often normal in incomplete proximal axon-loss lesions due to the presence of many unaffected motor and sensory fibers that are capable of generating a normal response. These late responses are also often normal in proximal demyelinating lesions wherein focal slowing is limited to a small segment of the entire nerve pathway or conduction is preserved through the affected region by nondemyelinated nerve fibers. Moreover, F-waves are typically mediated by two or more nerve roots. As such, they tend to be normal in axonal or demyelinating mono-radiculopathies in which adjacent roots are spared. Unlike F-waves, H-reflexes are restricted in distribution and can only be recorded from the soleus (and occasionally the flexor carpi radialis) muscle in adults. H-reflexes tend to disappear in elderly individuals without known focal neurologic injuries.
Electromyography
For EMG , a needle electrode is inserted into a muscle for the purpose of directly recording the electrical activity (voltage changes) of the muscle fibers. In current EMG practice, two types of recording needle electrode are used, the concentric and the monopolar . A concentric needle consists of a cannula (reference electrode) and a core (active electrode), which are generally made of different materials. A monopolar needle consists of the active electrode alone covered with an insulating material except for its sharpened tip, which is the conductive area of the needle. When employing a monopolar recording electrode, the reference electrode is a surface electrode placed on the skin in close proximity to the active monopolar needle.
There are three separate phases of the evaluation. First, the electrical activity is evaluated during insertion of the needle electrode (i.e., “insertional activity”). In the second phase, the consultant searches for abnormal “spontaneous activity ” within the muscle which occurs when the electrode is stationary in the resting muscle. Multiple sites are assessed in each examined muscle. The type of abnormal spontaneous activity of greatest relevance to regional anesthesia-related nerve injury is the so-called fibrillation potential . A fibrillation potential is an EMG waveform generated by spontaneous firing of a single muscle fiber. Most fibrillation potentials discharge at a regular rate (Fig. 7.6). Positive sharp waves have the same origin and significance as fibrillation potentials. Fibrillations develop in acutely denervated muscle fibers, which become supersensitive after a short delay following the injury. They also occur in many muscle diseases associated with segmental necrosis of muscle fibers or primary electrical instability of the muscle fiber membrane.
