Evaluation and Management of Liver Failure
Gowri Kularatna
Mauricio Lisker-Melman
Introduction
Liver failure results from progressive deterioration of hepatic function in the setting of either acute or chronic liver disease. Acute or fulminant hepatic failure (FHF) is a devastating condition in which the liver fails within a short period of time, encompassing a range of clinical syndromes. FHF is an uncommon entity, estimated to affect approximately 2,000 patients annually in the United States. Prompt evaluation and aggressive management, including liver transplantation, play an integral part in successfully treating patients with FHF in the intensive care unit (ICU). In contrast, chronic liver failure is a more frequent medical condition that may evolve into end-stage liver disease, independent of its cause (e.g., viral, autoimmune, metabolic, toxic). It usually develops after years of hepatocyte insult. The definition, etiology, clinical features, complications, and management of fulminant and chronic liver failure are reviewed in this chapter. The role of liver transplantation and future therapies for fulminant and chronic liver disease are discussed briefly.
Fulminant Hepatic Failure
Definition
Fulminant hepatic failure (FHF) is a rare condition which includes evidence of coagulation abnormalities (international normalized ratio [INR] > 1.5) and any degree of mental alteration (encephalopathy) in a patient without preexisting cirrhosis and with an illness of no more than 24-week duration. This definition can be further classified by length of illness: hyperacute liver failure (7 days), acute liver failure (8 to 28 days), subacute liver failure (29 to 60 days), late-onset hepatic failure (8 to 24 weeks), and subfulminant hepatic failure (jaundice to encephalopathy in 2 to 12 weeks). These terms, however, are not helpful, as they do not provide prognostic or practical information separate from etiology. Terms such as late-onset hepatic failure (8 to 24 weeks from the beginning of symptoms) and subfulminant hepatic failure (jaundice and encephalopathy in 2 to 12 weeks) are not commonly used. Patients with acute liver failure superimposed on a chronic liver disease do not fit the classic definition of FHF. There are classifications [1,2], however, that allow for the inclusion of these patients with previously asymptomatic chronic conditions as having FHF.
Etiology
Numerous causes of FHF are recognized, and their relative importance differs around the world. Some authors classify them into four major categories: infectious (e.g., viral hepatitis), drugs/chemicals/toxins (e.g., acetaminophen, isoniazid, Amanita phalloides), vascular (e.g., Budd–Chiari syndrome, ischemic hepatitis, tamponade), and metabolic (e.g., Wilson’s disease, Reye’s syndrome, acute fatty liver of pregnancy). In the United States, acetaminophen accounts for nearly 50% of cases followed by idiosyncratic drug reactions (12%). Acute viral hepatitis (hepatitis A and hepatitis B) is the etiologic factor in 12% of cases and has become an infrequent cause of FHF over the last few decades due to effective immunization programs. In contrast, in the developing world, viral hepatitis is the dominant etiology of fulminant hepatitis. Hepatitis C is a rare source of FHF [3].
The identification of the cause of FHF is important because it can provide important prognostic information as well as dictate treatments and antidotes. In the case of viral hepatitis, patient contacts must be identified and informed of the potential need for prophylaxis as indicated. Approximately 20% of adult cases with acute liver failure have indeterminate causes, with women affected more often than men [4,5]. Initial laboratory
testing to delineate etiology and assess degree of injury is obligatory (Table 95.1).
testing to delineate etiology and assess degree of injury is obligatory (Table 95.1).
Table 95.1 Initial Laboratory Testing for FHF | |||
---|---|---|---|
|
Acetaminophen is the most common cause of FHF in the United States and the United Kingdom. This drug is a constituent in numerous over-the-counter preparations and is also commonly combined with prescription analgesics. Although the recommended doses of acetaminophen (up to 4 g per day) are safe in healthy individuals, dose-dependent hepatotoxicity can occur. Hepatotoxicity can occur through intentional (e.g., suicide attempt) or unintentional (e.g., combined with alcohol, barbiturates, or other inducers of the cytochrome P450 system) overdose. Approximately one third of cases can occur in association with efforts at pain relief; these “therapeutic misadventures” occur with lower cumulative doses of ingested acetaminophen [6]. These patients may seek late medical attention resulting in delayed physician recognition.
Acetaminophen is 95% eliminated by hepatic conjugation. Approximately 5% of acetaminophen is converted to N-acetyl-p-benzoquinone-imine (NAPQI), which is inactivated after reaction with cellular glutathione and excreted rapidly. If glutathione stores are depleted, NAPQI becomes highly toxic and produces massive liver necrosis. NAPQI accumulates at a higher rate in the setting of increased cytochrome P450–2E1 activity, as seen with chronic alcohol ingestion, and with medications that induce the P450 system. As a result, patients with chronic moderate alcohol intake can develop toxicity with ingestion of less than 10 g of acetaminophen. For more detail on this topic, please refer to chapter 120.
Patients with acetaminophen toxicity present in three phases. The first phase involves acute gastrointestinal (GI) symptoms of nausea, vomiting, and abdominal pain within the first few hours after ingestion. During the second phase (12 to 48 hours), asymptomatic liver test abnormalities occur, with marked elevation of liver enzymes and a high aspartate aminotransferase (AST)/alanine aminotransferase (ALT) ratio. The third phase presents with manifestations of hepatic failure, including jaundice and encephalopathy. The mortality of acetaminophen toxicity is higher when associated with severe acidosis, coagulopathy, renal failure, mental status changes, and cerebral edema (CE) [6] (Table 95.2).
The effective antidote, N-acetylcysteine (NAC), is potentially lifesaving when administered early, especially within 24 hours of acute ingestion. Thus, suspicion and early identification of acetaminophen toxicity are of vital importance in treating patients presenting with FHF. NAC replenishes glutathione stores, preventing depletion and subsequent tissue hypoxia and ischemic damage. Both, the oral and intravenous forms can be used with similar efficacy [7]. Oral NAC is given over 72 hours with a loading dose of 140 mg per kg and subsequent doses of 70 mg per kg every 4 hours for a total of 17 doses. Because of its sulfur moiety, NAC has a strong odor and taste, and it needs to be diluted (usually in cola) to be tolerated. It is not uncommon for oral NAC to induce nausea and vomiting. A nasogastric or nasoduodenal feeding tube
may be placed in this situation to reliably deliver the medication. If the patient develops significant nausea or vomiting, has a polysubstance overdose requiring gastric decontamination, GI bleeding, or intestinal obstruction, IV NAC is the preferable administration route. IV NAC is given as a 150 mg per kg loading dose, then 50 mg per kg over 4 hours, followed by 100 mg per kg over 16 hours. It has been our experience that dosing of both, oral and IV NAC, can be administered beyond the recommended doses until improvement in liver chemistry parameters is noted. Anaphylactoid reactions are seen in 5% to 14% of patients, typically occurring with the IV loading dose [8,9]. Because this reaction may be rate dependent, the loading dose has to be administered over 15 to 60 minutes.
may be placed in this situation to reliably deliver the medication. If the patient develops significant nausea or vomiting, has a polysubstance overdose requiring gastric decontamination, GI bleeding, or intestinal obstruction, IV NAC is the preferable administration route. IV NAC is given as a 150 mg per kg loading dose, then 50 mg per kg over 4 hours, followed by 100 mg per kg over 16 hours. It has been our experience that dosing of both, oral and IV NAC, can be administered beyond the recommended doses until improvement in liver chemistry parameters is noted. Anaphylactoid reactions are seen in 5% to 14% of patients, typically occurring with the IV loading dose [8,9]. Because this reaction may be rate dependent, the loading dose has to be administered over 15 to 60 minutes.
Table 95.2 Indicators of Poor Prognosis in Acute Liver Failure | ||||||||
---|---|---|---|---|---|---|---|---|
|
The decision to administer NAC is dependent on serum levels of acetaminophen and time after ingestion (consult nomogram in acetaminophen chapter 120). Although the therapeutic benefit of NAC is best when given within 10 hours, its effects can still be of value within 36 hours of ingestion. When administered early, NAC leads to greater than 95% survival. The administration of NAC to patients with nonacetaminophen FHF has shown possible benefit [10]. However, combined analyses of these data do not demonstrate a mortality benefit in nonacetaminophen hepatotoxicity cases [11]. Larger controlled trials are needed to better assess the use of NAC in these patients.
Clinical Manifestations and Management
Despite timely intervention, multisystem organ failure can develop in patients with FHF. Hepatic encephalopathy (HE) and CE affect patients with FHF in varying degrees. Coagulopathy and jaundice are often present before severe encephalopathy occurs. Cardiorespiratory failure, renal failure, and infectious complications can develop and further complicate the management of these patients. Unfortunately, multisystem organ failure is a cause of ineligibility for transplantation in 20% of patients with FHF [12].
Hepatic Encephalopathy
HE is a syndrome of disordered consciousness and altered neuromuscular activity. HE is present in all patients with FHF, with symptoms ranging from subclinical confusion (grade 0) to coma (grade 4) (Table 95.3). The mechanism of HE is complex with interplay of many hypothesized factors including CE; toxins such as ammonia, glutamate, and endogenous benzodiazepines; and cytokine-like mediators such as interleukin (IL-1), IL-6, and tumor necrosis factor [13,14,15]. Alterations in cerebral blood flow due to loss of intracranial autoregulation may be a minor contributor to HE [16]. The diagnosis of mild HE is made through interviewing the patient for signs of impaired cognition. This may manifest itself through mild agitation, inability to concentrate, or more subtly, as an inability to perform counting tasks. HE may progress rapidly to grade 3 or 4, and frequent monitoring is essential. Arterial ammonia level more than 200 μg per dL is associated with cerebral herniation, although levels do not correlate with the degree of HE [17]. Mechanical intubation is indicated in the setting of significant acidosis or grade 4 HE, to protect patient’s airway and decrease metabolic stress. Treatment is directed at the correction of precipitating factors such as sepsis, GI bleeding, medications, and fluid and electrolyte imbalance [18]. Standard therapy for HE in chronic liver disease, such as lactulose, neomycin, or rifaximin, have no proven benefits in FHF and can induce significant side effects such as bloating, abdominal pain, nausea, and electrolyte disturbances from diarrheal losses [19]. Flumazenil, a benzodiazepine antagonist, had limited success in one trial treating HE in children with FHF, and no definitive data are available for its use in adults [20].
Table 95.3 Stages of Hepatic Encephalopathy | |
---|---|
|
Cerebral Edema
CE is a devastating complication of FHF that accounts for about 25% of deaths. It is seen in up to 80% of patients with FHF and advanced encephalopathy [21]. The pathogenesis of CE is thought to be the result of progressive accumulation of water in the brain. Two main mechanisms have been proposed to explain CE. The first is water influx down an osmotic gradient into the gray matter, astrocytes in particular [22]. Osmotic and metabolic alterations in the astrocytes lead to cellular swelling and accumulation of glutamine. The second mechanism is reduced cerebral flow and loss of autoregulation, resulting in increased intracranial pressure (ICP) [23].
The early clinical manifestations of CE often overlap with advanced encephalopathy (grades 3 and 4) and include agitation, headache, nausea, or vomiting. The most feared complications are brain herniation and death. Frequent evaluation of mental status (avoiding sedation and neuromuscular blockade if possible), assessment for hyperreflexia, pupillary changes, and sudden systemic hypertension play an important role in monitoring these patients. The neurologic examination and computed tomography (CT) of the brain are often unreliable methods to follow CE or ICP [24]. However, head CT can be helpful if there is clinical suspicion for structural lesions or hemorrhage. Severe CE, cerebral herniation, or a large intracranial bleed from an underlying coagulopathy eliminate the possibility of transplantation.
ICP measurements obtained by placement of intraparenchymal, subdural or epidural pressure transducers, accurately determine ICP and help direct therapy. Monitoring of ICP has always provoked controversy. Some suggest that these patients can be monitored without the need of invasive placement of intracranial bolts, while others suggest that ICP monitors facilitate the care of patients with CE [25,26]. ICP monitoring should be considered in patients with progressive mental status impairment (HE grades 3 and 4) and under consideration for liver transplantation. Placement of ICP monitors may be complicated by intracranial bleeding (< 5%) in the setting of severe coagulopathy [27,28] and volume overload from the use of blood products in an effort to correct coagulation abnormalities. Activated recombinant factor VII (see “Coagulopathy” section in the chapter) may help ameliorate these complications. Infections from monitor placement are also potential life-threatening complications. As a result, the efficacy and indication for ICP monitoring in patients with encephalopathy has been questioned [29]. Once the decision to place an ICP monitor is made, epidural pressure transducers are preferred because of a lower rate of bleeding complications (approximately 5%) compared with the intraparenchymal and subdural types (approximately 20%). Measurement of ICP with these monitors requires experience and familiarity with the equipment [30]. Monitoring mean arterial pressures (MAPs) and maintaining cerebral perfusion pressures (CPPs) (MAP minus ICP) are important. Ideally, ICP should be maintained around 15 mm Hg, with CPP greater than 40 to 50 mm Hg. Recovery of neurologic
function is optimized by maintaining CPPs at greater than 40 mm Hg.
function is optimized by maintaining CPPs at greater than 40 mm Hg.
Simple recognized strategies to stabilize or decrease the ICP include elevation of the head of the bed to 30 degrees to improve venous drainage and minimization of endotracheal suction and external stimuli [31], control of fever, correction of hyponatremia and hypoosmolality, and short-term hyperventilation to a PCO2 of 30 to 35 mm Hg [32]. Intravenous administration of mannitol (100 mL of 20% solution given intravenously at 0.5 to 1.0 mg per kg) and intravenous hypertonic saline boluses (20 mL 30% saline) to obtain sodium levels of 145 to 155 mEq per L are considered first-line treatment strategy for CE. Mannitol draws water osmotically from brain tissue reducing the ICP and may be helpful to “bridge” patients to liver transplantation. It should be avoided in patients with renal failure. Hypertonic saline infusions have been used to decrease the incidence and severity of increased ICP in FHF [33]. Hyponatremia increases ammonia-induced CE and has been recognized as a cause of this complication in postoperative patients treated with excessive 5% glucose solutions [23].
No established role exists for the use of regular diuretics. Administration of corticosteroids such as dexamethasone has failed to show any benefit in treating elevated ICP [34]. Hypothermia (32°C to 34°C) reduces the production of inflammatory mediators, decreases arterial and cerebrospinal spinal fluid ammonia levels, and attenuates ICP pressure; however, no control studies are available, and its use as a therapeutic option remains uncertain [35,36,37,38]. Barbiturates have been investigated as a treatment option for CE in FHF; however, with the exception of patients with seizures and CE, barbiturates are not useful. Experimental use of NAC and prostaglandin I2 infusions increases cerebral microcirculation and blood flow. The role of these agents remains to be determined in human studies.
Definitive treatment of CE in FHF is liver transplantation. Prolonged low CPPs and increased ICP are contraindications to liver transplantation given the high risk of brain death and significant neurologic sequelae. Despite the most appropriate care and stringent inclusion criteria, residual neurologic deficits may persist after transplantation [39].
Coagulopathy
Because of the loss of hepatocyte function in FHF, there is reduced synthesis of coagulation and anticoagulation factors that results in marked elevation of the INR, prolongation of the prothrombin, and activated partial thromboplastin times. These parameters should be followed to monitor hepatic function recovery (in the absence of fresh frozen plasma). Factor V has the shortest half-life and is a sensitive marker of defective synthesis of coagulation factors, but it is rarely measured. Along with clotting factor deficiencies and consumption, there may be platelet dysfunction, thrombocytopenia, and fibrinolysis resulting in a clinical picture similar to disseminated intravascular coagulation [40].
In the setting of coagulopathy due to clotting factor synthesis deficiency, overt bleeding does not frequently occur. However, when severe coagulopathy develops along with platelet dysfunction, GI and oropharyngeal bleeding can result. Protection of the gastric mucosa with proton pump inhibitors, H2 blockers, or sucralfate is important. The skin, lungs, and urogenital tract are also potential sites of significant blood loss. Fresh frozen plasma and platelets are indicated in patients with active bleeding or before invasive procedures. Packed cell replacement is required for significant blood loss. However, due to risk of worsening CE and fluid overload, blood products use must be judicious.
Factor VII is a central figure in the clotting cascade. Its activation is in response to tissue factor release after endothelial injury [41]. Recombinant activated factor VII (rFVIIa) has emerged as a potential treatment and prophylaxis option for bleeding in patients with liver disease. Prophylactic use of rFVIIa is not standard of care. In a group of children with FHF, prophylactic rFVIIa allowed for decreased transfusion requirements and better maintenance of fluid balance [42]. Data also supports rFVIIa use in patients who are undergoing placement of ICP monitors. In seven patients receiving rFVIIa, complete normalization of the prothrombin time (PT) allowed ICP monitor placement in comparison with three out of eight historical controls managed by conventional means. The patients receiving rFVIIa also had a significant decrease in mortality and anasarca due to fluid overload. The high cost of rFVIIa limits its more frequent use (approximately $2,000 for a 60 kg patient). However, the cost savings from decreased transfusion requirements and hemofiltration for fluid overload may offset the additional costs of rFVIIa [43].
Cardiac Complications
Elevated cardiac output (hyperdynamic circulation), decreased peripheral oxygen extraction (tissue hypoxia), and low systemic vascular resistance are present in patients with FHF [44]. These hemodynamic parameters, possibly due to the release of vasoactive mediators from dying hepatocytes, are similar to sepsis. Splanchnic and peripheral vasodilatation leading to systemic hypotension should be treated with volume replacement. Pulmonary artery catheter, central venous pressure, or esophageal Doppler monitoring can be crucial in assessing and correcting fluid status in these patients. MAP should be maintained above 50 mm Hg. Norepinephrine is generally used as the primary vasopressor due to its consistent effects on CPP. Conflicting data exist regarding vasopressin and terlipressin and their effects on ICP and systemic hemodynamics. A progressive rise in systolic blood pressure, within minutes or hours, can be indicative of ICP elevation and should be treated as discussed earlier.
Respiratory Complications
Respiratory complications occur frequently in patients with FHF. Hypoxemia can result from acute lung injury, cardiogenic or noncardiogenic pulmonary edema, pneumonia, intra-alveolar hemorrhage, or intrapulmonary vascular shunting (hepatopulmonary syndrome). These patients should be treated with supplemental oxygen or intubation as clinically indicated. Intubation, however, is used more frequently for airway protection rather than for respiratory failure. Positive pressure ventilation should be used to optimize compliance with caution, as the resulting decreased vascular return can lead to increased ICP and cardiac output. The combination of rising ICP and metabolic acidosis in patients with FHF leads to a compensatory hyperventilation and hypocapnia.
Monitoring the development of CE becomes difficult in patients who are intubated, sedated, and paralyzed. As a result, intubation should be performed in those who are unresponsive in a grade 3 or 4 HE and require airway protection with ventilatory assistance.
Renal Failure
The development of renal failure in FHF is a poor prognostic indicator and is the result of a variety of factors. Up to 75% of patients with acetaminophen toxicity develop acute renal failure from direct renal toxicity of NAPQI. Renal failure also occurs in approximately 30% of patient with FHF from other causes [8]. The most frequent causes of renal failure are intravascular volume depletion (relative hypovolemia caused by vasodilatation), acute tubular necrosis, and hepatorenal syndrome (HRS). Type I HRS predominates in FHF. It is rapidly progressive and carries a dismal prognosis.
Oliguric renal failure in FHF is defined as urine output less than 300 mL per 24 hours [45] or serum creatinine 3.4 mg per dL (> 300 mmol per L). Renal failure leads to a variety of metabolic disorders including hyperkalemia, hypercalcemia, hyperphosphatemia, hypophosphatemia, and hypermagnesemia that can further complicate patient management.
Diagnosis requires close monitoring of urine output, volume status (see “Cardiac Complications” section in the chapter), and measurement of urinary sodium and creatinine. Urinary sodium and creatinine can help identify the presence of acute tubular necrosis (high or normal urine sodium) but cannot differentiate between prerenal azotemia and HRS (low urine sodium). Nephrotoxic agents and mannitol should be avoided in renal failure, and careful renal dosing is vital. Some patients will require hemodialysis or hemofiltration to control the volume status, especially in the setting of CE, correct acidosis, improve electrolyte imbalance, and azotemia. Patients with tenuous hemodynamics who require hemodialysis or hemofiltration are best served with continuous modes of renal replacement [46].
Metabolic Disorders
Lactic acidosis is a severe metabolic complication of FHF. Serum lactate accumulates as a result of tissue hypoxia from hypotension as well as impaired hepatic uptake and metabolism of lactate. Renal dysfunction and other metabolic abnormalities can easily contribute to increase the underlying acidosis. Severe, refractory lactic acidosis requires intravenous bicarbonate infusion or dialysis.
Hypoglycemia also frequently complicates FHF given the primary metabolic role of the liver in glycogen storage and gluconeogenesis. Massive hepatic damage is required before serum glucose drops to levels that impair neurologic and cellular function. Nonetheless, frequent glucose monitoring and infusion of concentrated dextrose solutions may be required [47].
Sepsis
Patients with FHF are at high risk for septic complications. Abnormal neutrophil and Kupffer cell function, decreased bacterial opsonization, bacterial gut translocation, and altered cytokines contribute to immunologic impairment [48]. Up to 80% of patients with FHF will develop a bacterial infection. The urinary tract and pulmonary system are the most frequent sources of infection. Skin wounds, indwelling vascular access catheters, and ICP monitors are also potential sources of infection. The most common organisms identified are Staphylococcus, Streptococcus, Gram-negative organisms, and Candida species. Fungal infections occur late in the course of illness and are associated with high mortality.
Because the hemodynamic, metabolic, and hematologic parameters of FHF are often indistinguishable, hospital staff must maintain a high level of suspicion for infectious complications. In fact, one third of septic patients maintain a normal white cell count and remained afebrile. Since sepsis may be easily overlooked, early surveillance cultures should be obtained with a low threshold to start broad-spectrum antibiotics. If possible, aminoglycosides should be avoided owing to their nephrotoxicity. The use of prophylactic antibiotics is controversial. The addition of enteral decontamination regimens has not been shown to decrease the incidence of infection [49]. Sepsis can be catastrophic, since it compromises the opportunity to proceed with transplantation and contribute to multisystem organ failure.
The systemic inflammatory response syndrome (SIRS) presents clinically with manifestations compatible with sepsis: fever or hypothermia, tachycardia, leucocytosis or leucopenia, and tachypnea or hyperventilation, and it can be triggered by a variety of infectious and noninfectious conditions [50]. In FHF, SIRS can be associated with infection or by the release of inflammatory cytokines from the necrotic hepatocytes; as such, high level of suspicion and early identification of infectious sources are needed for adequate treatment and ICU support.
Prognosis
Prognostic factors in patients with FHF have been investigated to determine patient survival and identify those who would be best treated by transplantation. The Kings College criteria [51] produced the first prognosis model and have been used most often worldwide (Table 95.2) for this purpose. These criteria have a high positive predictive value and remain useful today [52]. Recent papers suggest that elevated lactate levels on admission improves the sensitivity of the model to predict mortality in acetaminophen-induced FHF [53]. On admission to the ICU, the Acute Physiology and Chronic Health Evaluation (APACHE) II system has been found comparable with the Kings College criteria in those with acetaminophen-induced liver failure [54]. However, more recent studies have shown that the absence of these criteria may not be as reliable in predicting survival [55].
Age does not appear to play an important prognostic role in the situation of transplantation for FHF. Although the grade of encephalopathy may influence posttransplant outcome, its reliability has been debated. The role of serial Doppler ultrasounds has been investigated in predicting outcome by measuring the mean hepatic artery resistive index. Results have indicated that as the mean hepatic arterial resistive index increases, there is an associated poorer prognosis in those patients who meet transplant criteria [56]. Assessing patients with CT has also been investigated. A prior study indicates that patients with liver volumes of less than 1,000 mL have a poorer prognosis [57].

Full access? Get Clinical Tree
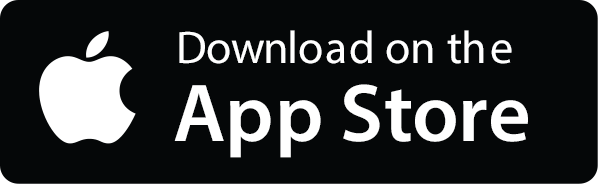
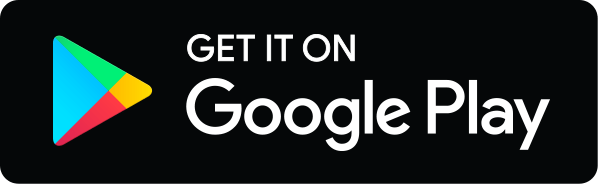