Fig. 45.1
Classic scalp recorded spike and waves in the right central-temporal head regions at arrows (south west arrow)
Currently, over 20 antiepileptic drugs (AEDs) are available but 20–40 % of patients cannot be successfully controlled on one or more drugs either due to ineffectiveness or unacceptable side effects [2, 3]. A lack of understanding of the generation of seizures inhibits the development of effective drugs . Mortality in drug-resistant patients is four times that in patients who are responsive to medication, prompting the desire for treatment to stop seizure activity to improve the quality of life and life expectancy. About 50 % of drug-resistant patients are candidates for surgery. Surgery to help the patient achieve a seizure-free state involves removing the neural tissue of the “epileptogenic zone” and is successful in 30–60 % of patients in terms of long-term freedom from seizures [2]. This region is often referred to as the epileptogenic zone : the region where ictal discharges originate is called the “irritative” zone and the region that produces symptoms is referred to as the “symptomatogenic” zone .
Whether seizures are focal or generalized determines the type of surgical procedure . Some seizures are partial (starting in one hemisphere and affecting only part of the central nervous system) while some are generalized (starting in both hemispheres). Partial seizures may be preceded by an aura. Seizures are thought to originate in one or more localized zones thought to be the result of central nervous system (CNS ) insults, which are usually never identified. Complete resection of the epileptogenic zone leads to the best results with freedom from seizures. In some patients the epileptogenic zone is impossible to resect because it cannot be identified, exists in multiple hemispheres, or because it overlaps with important eloquent cortex (e.g., motor or speech areas). These patients may be candidates for nonresective procedures such as disconnection of pathways that propagate the seizure (e.g., corpus callosotomy ) or stimulation/modulation techniques (deep brain stimulation, vagal nerve stimulation, trigeminal nerve stimulation, responsive neurostimulation) [4].
When being evaluated for surgery, patients undergo simultaneous video and standard scalp 24-h EEG monitoring from 32 or more channels to characterize their clinical manifestations and associated electrographic patterns. Termed Phase I, video EEG monitoring (vEEG ) can take days to a week depending on the successfulness of recording seizures. These patients are admitted into a dedicated epilepsy monitoring unit (EMU) generally with simultaneous withdrawal of AEDs. This facilitates the ability to record multiple seizures or nonepileptic events not requiring surgery within a short period of time. The patient or present family member participates in their seizure identification by actively pressing a portable alarm system. This alerts caregivers of the need to ensure patient safety during the ictal phase of the seizure, test the patient’s postictal clinical condition, and review the simultaneously captured vEEG .
During the admission, standard activation procedures such as hyperventilation and sleep deprivation are used to enhance seizure capture. There may be multiple triggers for seizures. Therefore, it is important to use provocative techniques based on patient history to maximize the effectiveness of the patient admission. Video EEG is considered the standard in distinguishing seizures from other nonseizures such as parasomnias , syncopal episodes , and events with a psychogenic origin [5]. It is important to characterize the clinical context of the semiology of the patient’s event to further help localize the region of seizure onset (epileptogenic zone ). The recent advances of EEG-triggered functional MRI (fMRI) allow vEEG to be recorded simultaneously while performing fMRI during ictal and interictal periods. The premise being that since seizure activity causes increased cerebral hemodynamic changes, these can be correlated with fMRI blood oxygen level-dependent technique alongside the vEEG to closer pinpoint the epileptogenic zone [6]. Due to its practical limitations (recording during ictal events) its practice, while compelling, is limited.
Functional MRI , however, can play a useful role preoperatively in the identification of critical anatomical locations and their functions. Using rapid echo planar imaging while the patient performs multiple motor tasks such as tongue movements , fist clenching , toe or finger taps and a multiple language task such as the verb generation task or the semantic decision-making task, detects small changes in signal intensity related to changes in cerebral blood flow. Computerized image processing can then define the areas of cortex activated by the specific task. Simultaneous three-dimensional rendering of cerebral topography gives a unique display of critical relational anatomy, creating both a structural and functional brain model. The ability to localize different body representations in the primary motor and somatosensory cortex, as well as for localizing and lateralizing language function prior to surgery, is a complementary presurgical evaluation with the Gold Standard Wada test (intracarotid amobarbital testing) for language hemispheric dominance and for guidance with intraoperative cortical stimulation [7].
Because scalp vEEG recording is a considerable distance away from where a seizure may originate, scalp electrodes are only capable of detecting a seizure discharge after it has spread considerably. While scalp vEEG is good at giving an overview of the electrical activity of the brain and suggests where the seizures are originating, if surgical ablation of an epileptogenic zone is being considered, further patient assessment is needed during Phase II and/or Phase III monitoring. Phase II monitoring involves a craniotomy , where placement of multiple-sized subdural grids and strip electrodes are placed bilaterally depending on the presumed epileptogenic zone (Fig. 45.2). If further hemispheric localization is needed, Phase III monitoring involves a unilateral craniotomy with electrode recordings from up to 128 contact points and may involve the addition of depth electrodes (stereoelectroencephalography ) in the hippocampal head regions. Surgical EEG recordings during these phases assure evidence of proper electrode contact with the cortical surface, creation of the preliminary maps identifying where the placement of the grids and electrode strips are located, and the ability to create the intracranial recording montage in the OR. Anesthesia management for this surgery is similar to others where electrocorticography is being recorded.
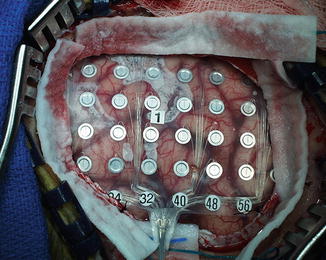
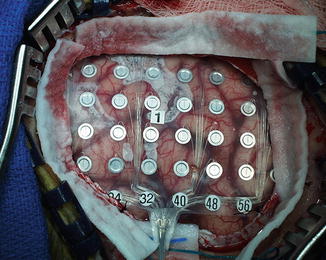
Fig. 45.2
Craniotomy showing 64-channel electrode grid placement on the cortical surface
Following surgical recovery, the patient is again monitored in an EMU where attempts are made to further lateralize the epileptogenic zone by recording from the implanted electrodes and additionally analyzing the seizures using computerized techniques such as three-dimensional imaging . Using cortical electrodes, recorded seizures from the epileptogenic zone usually have high-frequency oscillations or evolving “rapid discharges ” as compared to the standard spike and wave architecture seen from scalp leads [4] (Fig. 45.3). Phase II or III recording can last up to 2 weeks in the attempt to record multiple seizures with the same clinical manifestations (seizure signature ) and localize them to their epileptogenic zone.
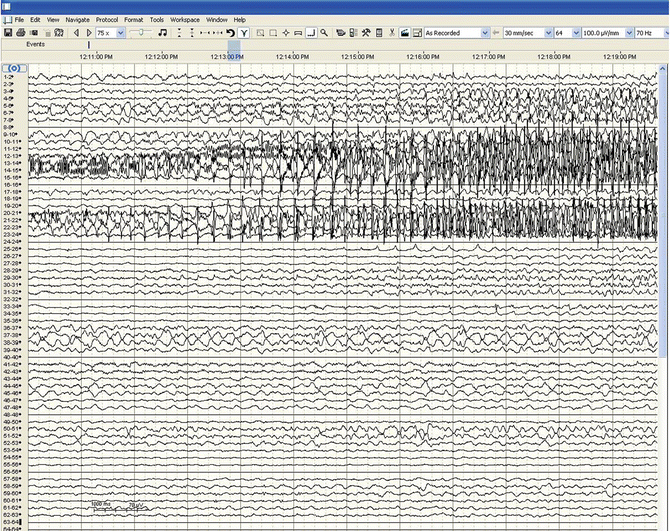
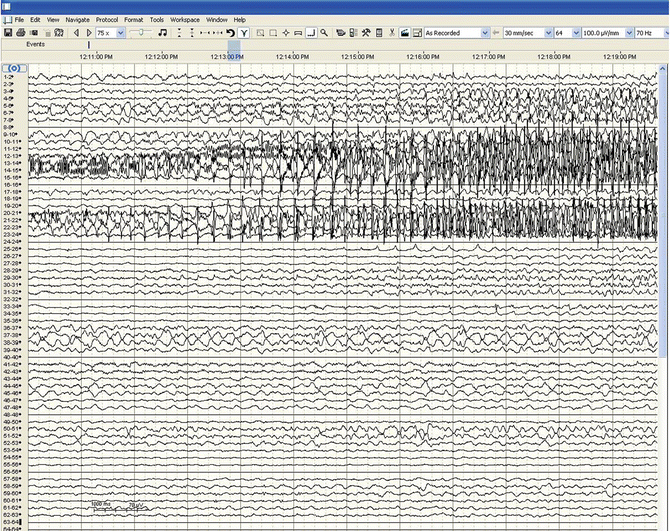
Fig. 45.3
Seizure activity consisting of high-frequency oscillations beginning in electrode contact point 14 followed by spread of the seizure to adjacent areas
The patient then returns for another craniotomy to remove the electrodes, determine the cortical tissue to be removed, map functional tissue to be avoided, and resect the epileptogenic zone. In the operating room, recording of EEG directly from the cortical surface (ECoG ) is valuable in the identification and localization of epileptic activity in preparation for pathological tissue resection as a means to control intractable epilepsy (Fig. 45.4a–c). Depending on the patient cooperativeness and the site of the epileptogenic zone, the approach to the surgery can either be done with the patient awake for the key portions (awake craniotomy ) or done under general anesthesia . The choice of anesthesia agents greatly affects the ECoG .
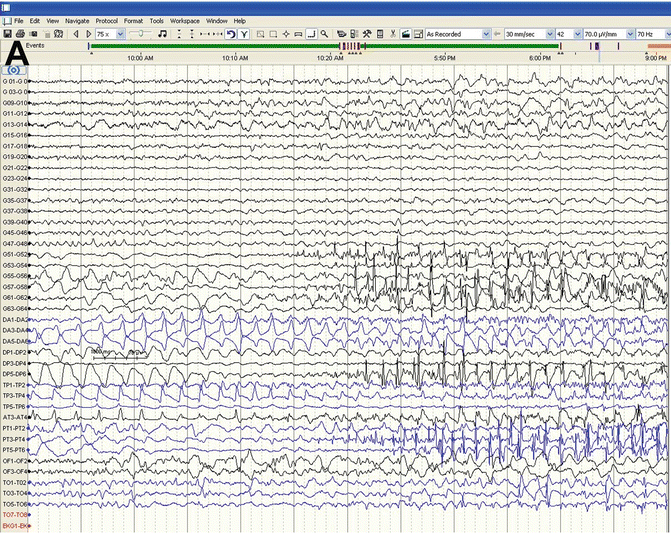
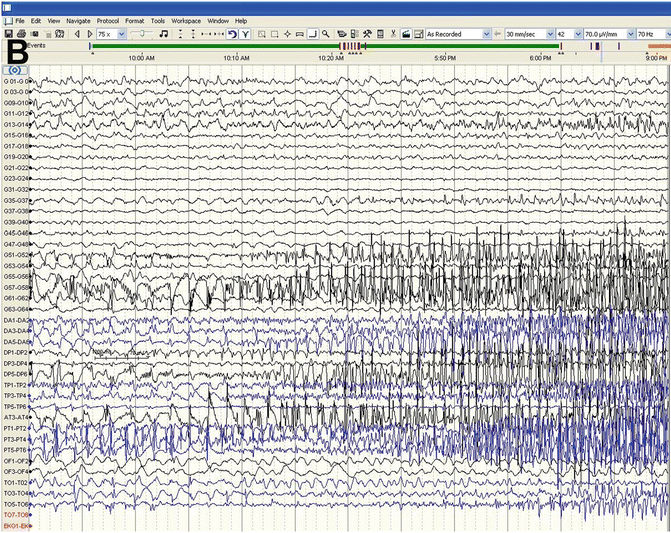
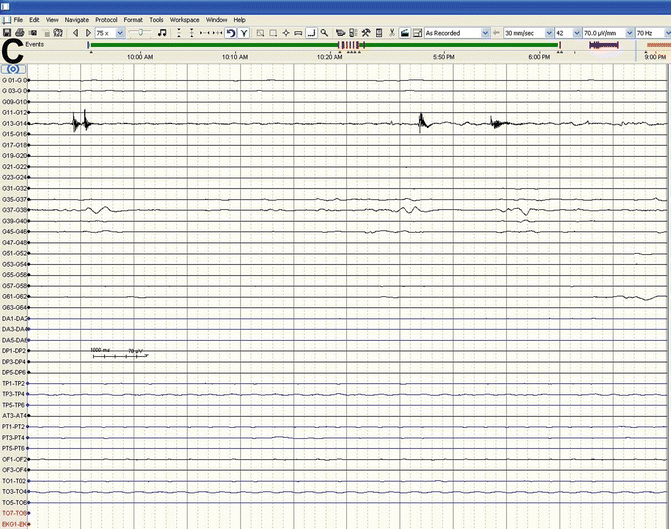
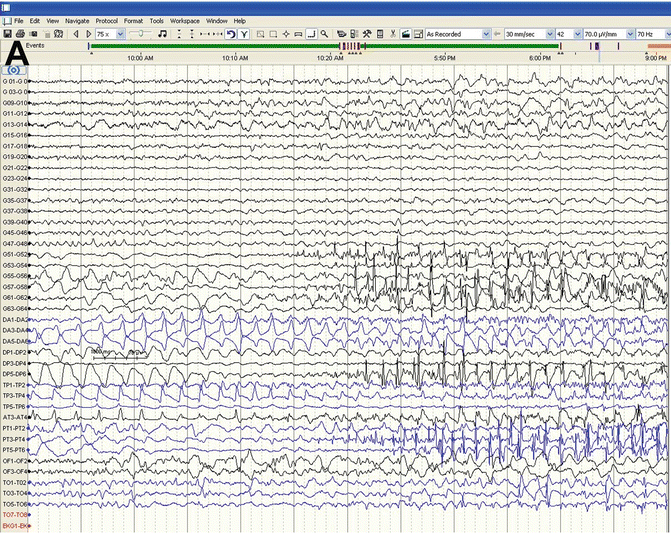
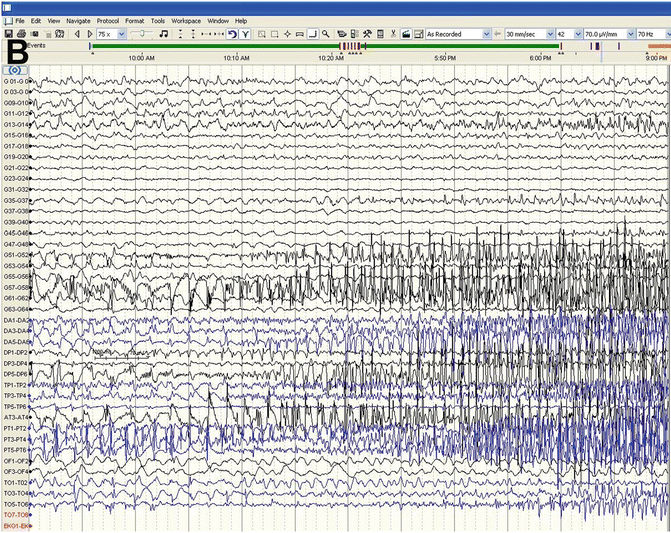
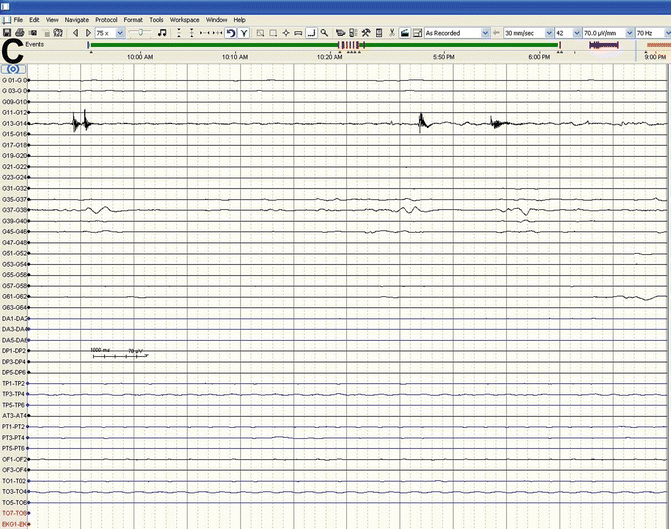
Fig. 45.4
(a) Start of Focal Seizure during ECoG beginning in the depth electrodes DA4/DA5 and anterior temporal grid strips. (b) Seizure spreads to adjacent cortical grids and strips. (c) Seizure stopped with saline applied directly to cortical surface—followed by cortical depression
Because of the patient’s ability to participate in identification of eloquent regions, awake craniotomy is the preferred technique when these must be identified in the dominant hemisphere. This also minimizes the impact of anesthesia agents. For anesthesia considerations for an awake craniotomy , see Chap. 18 (“Anesthesia for Awake Neurosurgery ”). In general, local anesthesia is used for the placement of stereotactic frames and head fixation devices (e.g., Mayfield) and topical anesthetics for discomfort of a urinary catheter. Infusions of propofol or dexmedetomidine with opioids (e.g., remifentanil) can assist during positioning and sedation until the craniotomy has been completed and the surgeons are ready for the procedure (at which time the infusions of the sedative and opioid are stopped).
However, general anesthesia will be needed in some patients who will be unable to cooperate or if an attempt at an awake procedure fails. If general anesthesia is used, it is important to maintain a stable light anesthetic during the ECoG recording using agents that minimize the depression of the ECoG signatures necessary for foci location. Opioids, with either propofol or dexmedetomidine, have been used. If halogenated agents are needed, very low doses of isoflurane or desflurane have been used [8]. Sevoflurane and nitrous oxide are not recommended.
During the craniotomy , electrophysiological monitoring is used for several components. First, ECoG is used to confirm or identify the epileptogenic zone to be ablated. If the epileptic activity is not sufficiently active, medications that have been used to activate it are shown in Table 45.1 [8]. Of note, some of these medications may activate seizure activity nonspecifically (i.e., from areas other than the epileptogenic zone, may induce seizures, and some have doses that may not be appropriate in the awake patient [notably opioids]). Ketamine is one of these nonspecific neurostimulants. Use of methohexital and alfentanil appears to have the largest experience. Activation may be particularly important if general anesthesia is used. Hyperventilation appears to increase the nonspecific activation [8].
Table 45.1
Intravenous medications which may activate epileptogenic foci
Drug | Dose |
---|---|
Methohexitala | 25–100 mg |
Etomidate | 0.2 mg/kg |
Propofol | 50–175 mg |
Fentanyla | 17–35 μg/kg |
Remifentanil | 1–2.5 μg/kg |
Alfentanil | 20–100 kg |
When the abnormal foci is located near the eloquent cortex (speech or motor cortex ), an awake craniotomy combined with ECoG allows for identification and localization of these regions near the epileptogenic zone. Defining the margin between abnormal and functional cortex allows normal brain preservation, complete epileptogenic zone resection, and a reasonable possibility of permanent seizure free state. Stimulation of the cortex is used to identify the functional tissue to be preserved.
Optimally, the identification of functional tissue to be spared is done during an awake craniotomy with preoperative identification of such areas using imaging techniques (e.g., single-positron emission tomography [SPECT ], PET for metabolically active areas with tasks, Wada testing to determine the dominant hemisphere, fMRI to create structural and functional brain models) or by use of the implanted electrodes at the bedside. In the operating room, mapping of these regions is done using different means of stimulation (handheld stimulators versus electrode grid strips) (Penfield technique versus direct cortical stimulation with motor evoked potentials; dcMEPs) and recording techniques are used to locate the eloquent cortex from the abnormal tissue [9] (see Chap. 9, “Brain and Spinal Cord Mapping”).
For motor cortex mapping (see Chap. 9), focal electrical stimulation of the cortex combined with a clinical response in the awake patient locates the motor cortex by causing nonvoluntary motor activity. When awake, the patient is asked to identify movement (often a “tug”) in response to stimulation. This patient participation can be continued if desired until the resection is complete or when the resection is halted as encroachment on the region is identified clinically. Occasionally the anesthesia team may identify associated movement. In the awake patient, the Penfield/Ojemann technique is typically used for stimulation.
For mapping of the speech-related areas, the brain is stimulated during a language-related task. For example, the patient may be shown a series of pictures of common objects and asked to name them (Boston Naming Test ). The eloquent cortex is identified when the task is interrupted (speech arrest/pause or word errors) [10].
Second, ECoG is used to identify undesirable after discharges or elicited seizure activity caused by the stimulation. The Penfield/Ojemann stimulation technique is associated with a moderate incidence of seizures so ECoG monitoring is important for seizure identification for this technique [11]. This activity may confound the identification of the initial epileptogenic foci by activating tissue adjacent to the epileptogenic zone and may pose a threat to the overall patient management (e.g., loss of airway in the wake patient). The EEG has characteristic waveforms after cortical stimulation and can be used to identify activity that is associated with preictal (precedes seizure) EEG, spreading seizure-like EEG, or an electrographic seizure. All of these EEG changes are usually stopped by cold saline applied directly on the exposed cortex. These can be followed by an appropriate (short acting) bolus of antiepileptic drugs (AED) such as propofol or methohexital should saline not terminate the seizure. Stimulation-induced seizures are frequent occurrences ranging from about 10 to 20 % of patients when a 50- to 60-Hz monopolar stimulator device is used (Penfield/Ojemann technique) [10, 12].
If general anesthesia is required or chosen, the motor cortex may be identified using direct stimulation of the cortex and recording of muscle responses using the motor evoked potential technique of direct cortex stimulation (dcMEP) and muscle recordings , as detailed in Chap. 2 (“Transcranial Motor Evoked Potentials”). For this stimulation, the high-frequency train technique for eliciting motor evoked potentials is more effective in overcoming the inhibitory effect of general anesthesia than the Penfield/Ojemann technique. As detailed in Chaps. 2 and 21 (“Intraoperative Neurophysiological Monitoring for Intracranial Aneurysm Surgery”), the stimulation parameters of the dcMEP technique are critical such that the motor cortex is stimulated rather than stimulation deeper in the subcortex, as would occur with a stronger stimulus.
If the direct cortical MEP stimulation technique is used, total intravenous anesthesia is required (see Chap. 19, “Anesthesia Management and Intraoperative Electrophysiological Monitoring ”). Muscle relaxants should also be avoided during this monitoring and train-of-four monitoring should be used to confirm resolution of any relaxants administered earlier in the procedure. As with the awake technique, monitoring can be continued during the resection. Fortunately, the incidence of stimulation-associated seizures is lower with the dcMEP technique (1–2 %) due to the reduction in voltage needed to produce the same outcome (see Chap. 9, “Brain and Spinal Cord Mapping ”) [10, 12, 13]. Identification of the speech areas under general anesthesia is not currently possible. Neuronavigation techniques may be helpful inferring the location of the eloquent cortex from preoperative imaging studies (as earlier). Some preliminary studies suggest direct cortical stimulation may be helpful as cortical stimulation of some speech-related regions may activate regions of the motor cortex related to speech [14, 15]. If during the Phase II or Phase III monitoring, vEEG in conjunction with high-resolution MRI identifies mesial temporal sclerosis in which seizures originate from focal areas of the hippocampus, a less invasive craniotomy is performed with the use of MRI-guided laser technology. Unlike traditional lobectomy, the use of a laser-tipped probe is directed through a burr hole in the back of the skull toward the hippocampus to ablate the epileptic foci. This minimally invasive surgery is performed under general anesthesia with hospital stays being reduced typically to overnight [16].

Full access? Get Clinical Tree
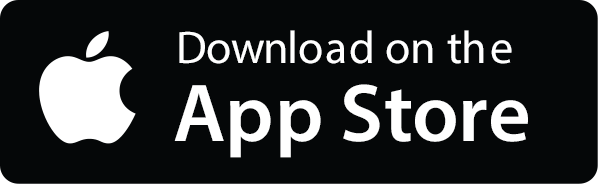
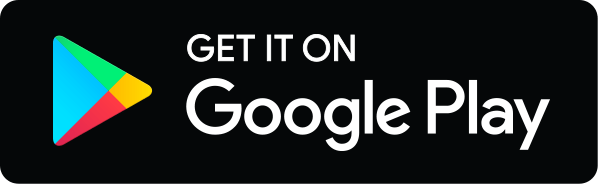