Figure 2-1. Formation of the blastocyst
Embryonic Period
The embryonic period is the time when all tissue is formed and, particularly during the second month, when all organs are formed. The fetal period is a time of organ growth.2 Embryologic development begins with fertilization, the process by which the male and female gametes unite to give rise to a zygote. Approximately 3 days after fertilization cells of the compacted embryo divide to form a morula, which is composed of an inner and outer cell mass. The inner cell mass gives rise to the tissues of the embryoblast, and the outer cell mass forms the trophoblast, which later contributes to the placenta. After a period of cell division, during which time the morula enters the uterine cavity, the blastocoele forms, and the embryo is known as a blastocyst (Figure 2-1).
By the eighth day of development the blastocyst is partially embedded in the endometrium. At this time the trophoblast differentiates into an inner and an outer layer. Lacunae develop in the outer layer, maternal sinusoids are eroded, and by the end of the second week a primitive uteroplacental circulation begins to develop.
The inner cell mass, or embryoblast, differentiates into two layers, the epiblast and the hypoblast, which together form the bilaminar germ disk.
The most characteristic event occurring during the third week of gestation is gastrulation. This is the process that establishes all three germ layers: endoderm, ectoderm, and mesoderm in the embryo (Figure 2-2). Gastrulation begins with the formation of the primitive streak on the surface of the epiblast portion of the bilaminar germ disk. Cells migrate toward the primitive streak, detach from the epiblast, and slip beneath it. This inward movement is known as invagination. Once the cells have invaginated, some displace the hypoblast, creating the new embryonic endoderm. Other cells come to lie between the epiblast and the newly created endoderm to form the mesoderm. Cells remaining in the epiblast then form the ectoderm. Through the process of gastrulation, the epiblast therefore, becomes the source of all the germ layers in the embryo.1 Developments during the first 3 weeks of the embryonic period therefore produce an embryo with: one germ layer (week 1), two germ layers (week 2) and three germ layers with a recognizable three-dimensional body form (week 32 (Figure 2-3).
In general terms the ectoderm germ layer gives rise to organs and structures that allow us to maintain contact with the outside world.4 It gives rise to the central and peripheral nervous systems; the sensory epithelium of the eye, ear and nose; the epidermis and its appendages; hair and nails, mammary glands; the hypophysis, subcutaneous glands; and the enamel of the teeth.
The mesoderm gives rise to supporting structures of the body, such as cartilage, bone, and connective tissue; striated and smooth muscle; the heart, blood, lymph vessels, and cells; the kidneys, gonads, and serous membranes lining the body cavities, spleen, and the cortex of the adrenal gland.
The endoderm produces the epithelial lining of the gastrointestinal and respiratory tracts, as well as the epithelial lining of the bladder and urethra, tympanic cavity, antrum, and auditory tube. It also engenders the parenchyma of the tonsils, thyroid, parathyroid thymus, liver, and pancreas.
As the embryo forms, it rapidly develops along several axes,2 the first of which is the craniocaudal axis. It is established while the embryo is still a flat disk or sheet of cells. This axis runs from the future head to future tail of the body form. The dorsoventral axis is the next to be established. This occurs as the body folds and defines the future front and back sides of the body form.
Establishment of the body axes takes place prior to and during the period of gastrulation. Cells at the posterior margin of the embryonic disk signal the craniocaudal axis. The dorsoventral orientation of tissue is controlled by a complex interaction of proteins and growth factors.
This early orientation of cells in the body is a result of the expression of Hox genes. There are four Hox gene complexes in vertebrates: Hoxa, b, c, and d. Each consists of a group of between 9 and 11 genes arranged sequentially along a particular chromosome. A cascade of genes producing signaling factors also orchestrates left—right asymmetry, which is established early in development. As a result of complex interactions, for example, the heart and spleen lie on the left side of the body and the main lobe of the liver lies on the right.
Regions of the epiblast that migrate and ingress through the primitive streak have been mapped and their ultimate fates determined. Mesoderm cells that ingress through the cranial region of the primitive node become the notochord, those migrating at the lateral edges of the primitive node and from the cranial end of the primitive streak become the paraxial mesoderm. Cells migrating through the midstreak region become the intermediate mesoderm, and those migrating through the caudal part of the streak form the lateral plate mesoderm. This orientation of the mesoderm is important in understanding limb development.
The embryonic disc, initially flat and almost round, gradually becomes elongated, with a broad cephalic and a narrow caudal end. Expansion of the embryonic disk occurs mainly in the cephalic region. This growth in elongation is caused by continuous migration of cells from the primitive streak region in a cephalad direction. Invagination and migration forward and laterally of surface cells in the primitive streak continue until the end of the fourth week. Germ cell layers in the cephalic region begin their specific differentiation by the middle of the third week, whereas those in the caudal part differentiate beginning by the end of the fourth week. This causes the embryo to develop in a cephalocaudal direction (Figure 2-4).
At the beginning of the third week of development the ectodermal germ layer has the shape of the disk. The ectoderm gives rise to two subdivisions: neuroectoderm, which forms all neural tissue, and the epidermal covering of the body.2 Appearance of the notochord and prechordal mesoderm induces the overlying ectoderm to thicken and form the neural plate (Figure 2-5). Cells of the neural plate make up the neuroectoderm, and their induction represents the initial event in the process of neurulation. By the end of the third week the lateral edges of the neural plate become elevated to form neural folds, and the depressed midregion forms the neural groove. Gradually, the neural folds approach each other in the mid line where they fuse, resulting in formation of the neural tube. Neurulation is then complete, and the central nervous system is represented by a closed tubular structure with a narrow caudal portion, the spinal cord, and a much broader cephalic portion characterized by a number of dilations, the brain vesicles. As the neural folds elevate and fuse, cells at the lateral border begin to dissociate from their neighbors. This cell population, called the neural crest, will undergo a transition as they leave the neuroectoderm to enter the underlying mesoderm. portion of the somite proliferate and migrate down the ventral side of the remaining dorsal epithelium of the somite to form a new layer, the myotome. The remaining dorsal epithelium forms the dermatome, and together these layers constitute the dermomyotome. Each segmentally arranged myotome contributes to muscles of the back, whereas the dermatomes disperse to form the dermis and subcutaneous tissue of the skin. Each myotome and dermatome retains its innervation from the segment of origin, no matter where the cells migrate to. Therefore, each somite forms its own sclerotome, the cartilage and bone component; its own myotome, providing the segmental muscle component; and its own dermatome, the segmental skin component. Each myotome and dermatome also has its owns segmental nerve component (Figure 2-9).
Figure 2-2. Establishment of the three basic germ layers: endoderm, ectoderm, and mesoderm in the embryo. A: Trophoblast with the shell removed. B: Gastrulation viewed from the dorsal surface. Solid arrows show movement of cells in epiblast; dashed arrows show movement of gastrulating cells below the epiblast. C: Differentiation of the basic germ layers
Figure 2-3. Cross section of germ layers as they appear during embryonic folding. A: Cross section. B: Cross section of germ layers after folding is completed. C: Longitudinal section
Figure 2-4. Development of the embryo in a cephalocaudal direction. A: Lateral body folds, cross-sectional views. B: Craniocaudal body folds, longitudinal views
Figure 2-5. Formation of the neural plate. A: Formation of the neural plate. B: Formation of the neural folds and neural groove. C: Completion of neurulation, the creation of the neural tube
Figure 2-7. Formation of paraxial mesoderm (A), embryonic cavity (B), and embryonic folding (C)
Figure 2-8. Development of the supporting tissues of the body myotome (muscle tissue), sclerotome (cartilage and bone), and dermatome (subcutaneous tissue). A: Paraxial mesoderm condenses to form the somite. B: Somite forms three regions. C: Somitomeres develop into somites
During the second month, the external appearance of the embryo is changed greatly by the enormous size of the head and formation of the limbs, face, ears, nose, and eyes.1 By the beginning of the fifth week, fore- and hindlimbs appear as paddle-shaped buds (Figure 2-10). The forelimbs are located dorsal to the pericardial swelling at the level of the fourth cervical to first thoracic somites, which explains their innervation by the brachial plexus. Hindlimb buds appear slightly later just caudal to the attachment of the umbilical stock at the level of the lumbar and uppers sacral somites. With further growth the terminal portions of the buds flatten, and a circular constriction separates them from the proximal, more cylindrical segment. Soon four radial grooves separating five slightly thicker areas appear on the distal portion of the buds. This development foreshadows formation of the digits. These grooves, known as rays, appear in the hand region first, and shortly afterward, in the foot because the upper limb is slightly more advanced in development than the lower limb. While fingers and toes are being formed, a second constriction divides the proximal portion of the buds into two segments, and the three parts characteristic of the adult extremities can be recognized.
Fetal Period
The period from the beginning of the ninth week to the end of the intrauterine life is known as the fetal period.1 It is characterized by maturation of tissues and organs and rapid growth of the body growth in length. This is particularly striking during the third, fourth, and fifth months, and increasing weight are most striking during the last 2 months of gestation. During the third month the face becomes mqre human-looking, and the limbs reach their relative length in comparison with the rest of the body, although the lower /limbs are still a little shorter and less well developed than the upper extremities. Primary ossification centers are present in the long bones and the skull by the twelfth week.
Skeletal System: Limb Growth & Development
The skeletal system develops from paraxial and lateral plate mesoderm as well as neural crest tissue.1 The somites (as previously described) differentiate into a ventromedial component called the sclerotome and a dorsolateral component called the dermomyotome. This organization of cells forms a loosely woven tissue called the mesenchyme. The mesenchyme migrates and differentiates into fibroblasts, chondroblasts, and osteoblasts.
At the end of the fourth week of development limb buds become visible as out-pocketings of the ventrolateral body wall.1 Initially they consist of a mesenchymal core derived from the somatic layer of lateral plate mesoderm that will form the bones and connective tissue of the limb, covered by a layer of ectoderm. Ectoderm at the distal border of the limb thickens and forms a specialized inducing tissue known as the apical ectodermal ridge (AER). The AER exerts an inductive influence on the adjacent mesenchyme, causing it to remain as a population of undifferentiated, rapidly proliferating cells called the progress zone. As the limb grows, cells farther from the influence of the AER begin to differentiate into cartilage and muscle. In this manner of development of the limb proceeds proximodistally. In 6-week-old embryos, the terminal portion of the limb buds become flattened to form hand and footplates and are separated from the proximal segment by a circular constriction. Later a second constriction divides the proximal portions into two segments, and the main parts of the extremities can be recognized.1 Fingers and toes are formed when programmed cell death in the AER separates this ridge into five parts. Further formation of the digits depends on their continued outgrowth under the influence of the five remaining segments of ridge ectoderm. This results in condensation of the mesenchyme to form cartilaginous digital rays. Development of the upper and lower limbs is similar except that morphogenesis of the lower limb is approximately 1- 2 days behind that of the upper limb.
During the seventh week of gestation a key event occurs that is critical in understanding the final orientation and innervation of the limbs. The limbs rotate in opposite directions. The upper limb rotates 90 degrees laterally so that the extensor muscles lie on the lateral and posterior surface and the thumbs lie laterally. The lower limb rotates approximately 90 degrees medially, placing the extensor muscles on the anterior surface and the great toe medially.1 This explains why homologous joints of the upper and lower extremities (knees and elbows) point in opposite directions. This limb rotation results in:2
1. The final orientation of the limbs
2. The final location and orientation of muscle groups (because the muscles are connected to the limb bones prior to rotation)
3. The patterns of sensory innervation of the skin (also because nerve fibers are connected with the dermis layer of the skin prior to rotation and are pulled along)

Full access? Get Clinical Tree
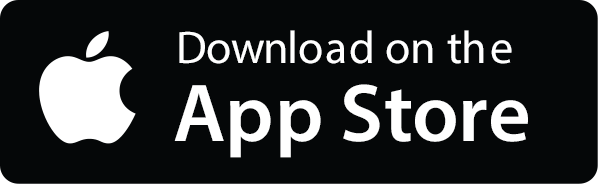
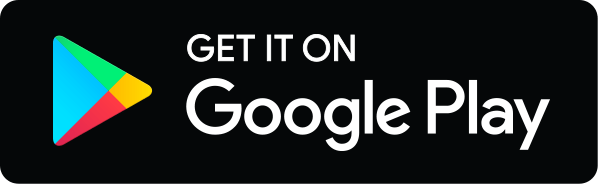