FIGURE 118.1 A: Nonlinear nature of the relationship between the volume of intracranial contents and intracranial pressure. B: Actual intracranial pressure (ICP) and jugular venous oxygen saturation (SjO2) tracings in a patient who developed a delayed intracranial hematoma. Initially the rise in ICP was minimal as the brain was able to compensate for the increasing volume from the hematoma. Later when these compensatory mechanisms were exhausted, there was a very rapid increase in ICP associated with compromise of cerebral blood flow and a decrease in SjO2.
These CBF thresholds were determined in normal brain, but seem to hold true for brain-injured patients, as well. As a result, a low CBF, particularly in the early postinjury period, is highly predictive of a poor outcome. Indeed, TBI patients with CBFs of less than 18 mL/100 g/min have a significantly worse outcome compared to patients with higher CBF (16) and within 12 hours of injury, every 10 mL/100 g/min increase in CBF was associated with a threefold increase in the probability of surviving the injury (17).
Brain oxygenation and cerebral perfusion can be monitored with both invasive and noninvasive techniques (7). For example, snapshots of oxygenation or perfusion can be acquired noninvasively using positron emission tomography (PET) or xenon computed tomography (CT) imaging, respectively. Similarly, qualitative assessments of CBF can be gathered from transcranial Doppler recordings, assuming that there is no appreciable change in the size of the measured vessel or the angle of measurement. On the other hand, invasive techniques can be used to continuously assess brain oxygenation and perfusion. For example, global assessment of both oxygenation and perfusion can be performed through combined monitoring of arterial oxygen saturation (SaO2) and the oxygen saturation in the jugular bulb (SjO2). This allows the calculation of the arteriojugular oxygen content difference (AJDO2), which is dependent on the amount of oxygen consumed by the brain (CMRO2) and on CBF by the formula CMRO2/CBF. Thus, an increased AJDO2 indicates a deficiency of flow relative to the metabolic needs of the brain. Brain oxygenation can also be monitored continuously in a local region of the brain by inserting an invasive probe to measure the brain tissue oxygen tension (PbO2). While continuous global monitoring provides an overall assessment of brain oxygenation, it can miss local changes (18). On the other hand, continuous local monitoring with an invasive probe provides information on a specific portion of the brain but is dependent on probe location and may not reflect overall brain perfusion (19).
DIAGNOSIS
Identification of Patients with Increased ICP
Symptoms of elevated ICP are progressive. For example, a patient with mildly increased ICP can present with complaints of only headache and blurred vision; further increases in ICP are associated with decreased level of consciousness. If the ICP continues to climb, this may be associated with signs of brain herniation. Herniation of the temporal lobe over the edge of the tentorium can compress cranial nerve III and cause ipsilateral pupillary dilation. It can also directly compress the brainstem and cause contralateral posturing or hemiparesis. However, if the brainstem is displaced and compressed against the opposite side of the tentorium, there may be ipsilateral symptoms. This is known as Kernohan notch phenomenon. Further symptoms of herniation are hypertension, bradycardia, and widening pulse pressure, which are classically known as the Cushing triad. Respiratory abnormalities may be also present, including Cheyne–Stokes respiration, hypoventilation, or central neurogenic hyperventilation. Any patient in whom elevated ICP is suspected should undergo an emergent noncontrast CT scan of the brain to evaluate for mass lesions, hydrocephalus, subarachnoid hemorrhage, or other treatable causes.
For TBI patients, a degree of suspicion can be made based on the initial Glasgow coma score (GCS). A patient with a GCS of 13 to 15, defined as a mild head injury, has minimal risk of deteriorating or developing elevated ICP. Patients with GCSs that range from 9 to 12, defined as moderate head injury, have a higher chance of deteriorating and developing elevated ICP. Finally patients with a GCS of 8 or less, defined as severe head injury, have the highest risk of developing elevated ICP, and should undergo ICP monitoring, particularly if they have an abnormal head CT or if they meet two of the three criteria of age older than 40 years, unilateral or bilateral motor posturing, or SBP below 90 mmHg (20).
TREATMENT
Initial Stabilization and Management of Patients with Elevated ICP
The initial steps in managing any patient with elevated ICP (Fig. 118.2) follow the ABCs of trauma resuscitation. First and foremost, airway protection is crucial and current guidelines recommend that an appropriate airway should be established in any patient with a GCS less than 9, the inability to maintain an airway, or hypoxemia not corrected with supplemental oxygen. While some studies have shown that intubation in field to accomplish this goal leads to lower mortality rates (21), others have suggested that the rapid sequence intubation (RSI) techniques used by paramedics result in higher mortality rates and poorer outcomes (22). In general, the routine use of neuromuscular blocking agents (NMBAs) for RSI is not recommended in spontaneously breathing patient maintaining saturations above 90%. It is also recommended that if a patient is intubated in the field, it should be performed by a well trained paramedic, hyperventilation should be avoided unless signs of herniation are evident (23), and end tidal CO2 monitors should be used to confirm placement of the tube in the airway when available (24,25).

FIGURE 118.2 Algorithm for initial management of patient with elevated intracranial pressure. ICU, intensive care unit; OR, operating room; SBP, systolic blood pressure.
Maintaining adequate blood oxygenation and pressure are also critical in patients with TBI. Episodes of hypoxemia (arterial PaO2 <60 mmHg) or hypotension (systolic blood pressure <90 mmHg) have been associated in numerous studies with significantly worse outcome in these patients (26–30) and rapid correction is associated with increased survival (31). While hypotension can be secondary to brain injury, it should not be thus attributed unless all other possible causes have been excluded. Hemodynamic resuscitation should begin in the emergency department with the placement of two large-bore IVs or a femoral line. A radial arterial blood pressure catheter is also useful for continuous blood pressure recording and volume resuscitation with 0.9% normal saline or blood is the first step for managing hypotension. If a vasopressor is required, norepinephrine is preferred as it produces the most consistent improvement in blood pressure and cerebral profusion.
Once resuscitation has been initiated, management of ICP should be addressed as soon as possible. Multiple studies have shown improved survival if patients with suspected elevated ICP are taken to a center with immediately available CT scanning, neurosurgical care, and the ability to monitor ICP in an ICU setting (32–34). Initiation of osmotic therapy with mannitol is effective at controlling raised ICP. However, ideally mannitol therapy is restricted to patients with ICP monitors or those who have signs of transtentorial herniation or progressive neurologic deterioration not attributable to other causes. Effective doses for mannitol range from 0.25 to 1 g/kg, with a 1 g/kg bolus being the standard dose. However, studies have shown improved outcome with preoperative doses as high as 1.4 g/kg in patients with signs of herniation secondary to subdural hematoma (35). Mannitol should only be given once fluid resuscitation has been completed as it does result in an osmotic diuresis and can cause hypotension in the incompletely resuscitated patient. Alternatively, hypertonic saline is another osmotic agent that can be used and has an advantage in a hypotensive patient since it does not cause diuresis.
Surgery
If a surgical lesion is identified, the patient should be taken immediately to the operating room for evacuation. Invasive monitoring devices can be inserted in the operating room if required; at a minimum, an ICP monitor should be inserted. Other monitoring devices that can be used if available include PbO2 and SjO2 catheters. An arterial line should also be placed and a central venous catheter may be needed, especially if hypotension is present or large doses of mannitol are required.
Commonly Monitored Parameters in Patients with Elevated ICP
Intracranial Pressure
Normal ICP in a healthy supine adult ranges between 7 and 15 mmHg (36). In a head-injured patient, several studies have demonstrated worse outcomes with ICP values greater than 20 mmHg (26,37–39) and values above 40 mmHg indicate severe life-threatening intracranial hypertension. Thus, the goal of treatment should be to maintain ICP values below 20 mmHg.
The gold standard for monitoring ICP is a ventriculostomy catheter inserted into one of the lateral ventricles and connected to an external strain gauge and drainage bag. This system provides the most accurate measurement of ICP and is stable over time (40). It can also be used to drain CSF and treat elevated ICP if needed. CSF should be intermittently drained to treat ICP except in cases that are associated with hydrocephalus, where continuous CSF drainage is optimal. In such cases, one of the secondary ICP monitors described below should be additionally used to continuously record ICP while the CSF is being drained. The main risk of ventriculostomy is infection, with rates ranging up to 20% (41). Antibiotic-impregnated ventriculostomy catheters, however, can reduce this risk significantly to around 1.5% (42) and thus should preferentially be used. Other problems often associated with ventriculostomy catheters, although to a much smaller degree, include obstruction, malposition, infection, and malfunction.
Other invasive monitors for measuring ICP include intraparenchymal, subarachnoid, and epidural monitors. The advantage of theses probes is they do not require insertion into the ventricle, which not only lowers the infection rate (41), but is also useful when the ventricle is collapsed or displaced by significant midline shift. However, these probes are less accurate and tend to drift over time (7). Of these monitors, intraparenchymal probes are arguably the most accurate with the least amount of drift, thus offering the best alternative to ventriculostomy (43).
Cerebral Perfusion Pressure
CPP is defined as the MAP minus the ICP and is a second major parameter that can be both monitored and treated in the patient with elevated ICP. CPPs below 50 mmHg should be avoided and can be supported by maintaining MAP and by lowering ICP, however, aggressive attempts to maintain CPPs above 70 mmHg using fluids and vasopressors should also be avoided secondary to the risk of acute respiratory distress syndrome (ARDS). Also, while patients with intact autoregulation can tolerate CPPs of 70 mmHg, patients with impaired autoregulation risk malignant hyperemia with CPPs above 60 mmHg (44). For most patients, a CPP of 60 mmHg is adequate (45) and guidelines recommend a range between 50 and 70 mmHg.
Brain Oxygenation
As describe above, brain tissue oxygenation can either be measured globally at a single time point using PET imaging techniques or continuously and locally by using probes to monitor PbO2 and/or SjO2. PET has provided important insights in TBI research, but is not widely available for ICU patients. In contrast, PbO2 and SjO2 monitoring can be used in any ICU setting.
Brain Tissue Oxygen Tension. PbO2 monitoring is accomplished by inserting an oxygen-sensing probe into brain tissue. The location of the probe is critical and determines the nature of the PbO2 information that will be obtained (Fig. 118.3). For example, if the probe is inserted near a focal lesion, oxygenation can be monitored in the tissue at greatest risk. In contrast, insertion of the probe in uninjured brain allows monitoring of a local area that should be representative of the overall less-injured oxygenation status of the brain (19). While normal values of brain PbO2 are not well established, multiple studies have shown associated time-dependent worse outcomes with PbO2 values lower than 10 to 15 mmHg (46–48) and values of less than 6 mmHg are also associated with poorer outcomes in a time-independent fashion (46). While some studies have shown reduced mortality when brain PbO2 values are kept around 25 mmHg (49) or 20 mmHg (50), in general the recommended treatment threshold is less than 15 mmHg.
Jugular Venous Oxygen Saturation. SjO2 is a measure of the oxygen saturation in the jugular bulb and can be monitored by inserting a catheter into the internal jugular vein and advancing toward the skull base. This allows measurement of the oxygen saturation of the blood exiting the brain, which provides information on the adequacy of CBF and oxygen delivery to the brain (18). Normal values for SjO2 are better studied than PbO2 values but are still not well established, with typical values from older series ranging from 55% to 71% (51). However, newer studies suggest that normal SjO2 values may be as low as 45% (52). Despite this, episodes of SjO2 desaturations to values less than 50% are associated with worse outcome (53) and should be treated. Increased SjO2 of greater than 75% may indicate decreased oxygen uptake in the brain (54) and is also an indicator of poor outcome. While it is a useful tool for global cerebral oxygenation assessment, the major limitation to SjO2 monitoring is that unlike brain PbO2, it cannot detect local ischemia within the brain.

FIGURE 118.3 Location of the brain tissue oxygen tension (PbO2) catheter relative to the injured brain determines the nature of the pO2 information that will be obtained. On the left is a patient where the PbO2 catheter was placed in relatively normal brain opposite a temporal contusion. The PbO2 reflected the global oxygenation of the brain measured with jugular venous oxygen saturation (SjO2). On the right is a patient where the PbO2 catheter was placed near a contusion. As this contusion evolved, the PbO2 decreased even though the global measures (SjO2 and cerebral perfusion pressure [CPP]) remained unchanged. MAP, mean arterial pressure.
Treatment of Elevated ICP: Principles of CNS Resuscitation
Principles of management of intracranial hypertension (Fig. 118.4) include general measures that are used in all patients to minimize factors that exacerbate ICP rise, first-line therapies that are applied to patients who subsequently have elevated ICP, and additional treatments that can be used if elevated ICP is refractory to these first-line measures.
General Measures
Prevention or treatment of factors that may aggravate or precipitate intracranial hypertension is the cornerstone of central nervous system (CNS) resuscitation. Specific factors that may aggravate intracranial hypertension include the obstruction of venous return, hypoxia, hypercapnia, fever, severe hypertension, hyponatremia, anemia, and seizures. Routine critical care management of the patient at risk for intracranial hypertension should include measures to prevent these factors as much as possible. Also important are general strategies for maintaining normal brain oxygenation and CPP.
Minimize Obstruction to Venous Return
Head of the bed elevation to 30 degrees and neutral head positioning maximize venous return from the brain and has been standard neurosurgery practice for management of ICP. While some studies initially suggested that this resulted in a decrease in CPP (55), other studies designed to specifically address this question found no significant reduction in CPP, CBF, or regional microcirculation in most patients (56,57). If head elevation is used, it is important to remember that both the ICP and blood pressure transducers should be zeroed at the same level (i.e., at the level of the foramen of Monro) to get accurate measurements of CPP. It is also important to look for other potential causes of elevated ICP in patients refractory to standard therapies. For example, in patients with abdominal injury, an increased intra-abdominal pressure may also impede venous return from the brain and increase ICP. In such cases, abdominal decompression can improve an otherwise refractory ICP (58).
Prevent Fever
Fever is common during the recovery from a head injury. Fever is a potent cerebral vasodilator and has been associated with increased intracranial temperature, metabolic requirements, and ICP (59,60). As a result, it is also associated with longer hospital stays and worse outcomes (61). Efficient external cooling systems as well as intravascular cooling devices are available and can maintain normal body temperature when needed. However, more importantly, when fever occurs, infectious causes should be investigated with appropriate cultures and treated with antibiotics.

FIGURE 118.4 Algorithm for treatment of elevated intracranial pressure (ICP). CT, computed tomography; CSF, cerebrospinal fluid.
Prevent Seizures
Posttraumatic seizures (PTS) are not uncommon after TBI. While upward of 11% of civilian patients with TBI can have a seizure within 5 years (62), the number who will have early PTS, characterized as seizures within the first week of injury, is closer to 3% (63). This incidence is strongly dependent upon the severity of the injury (64). Unfortunately, severe TBI patients, who have the highest risk of seizure—which approximates 22%—are often pharmacologically sedated and paralyzed to treat intracranial hypertension, rendering clinical monitoring ineffective. For these high-risk patients, continuous electroencephalogram (EEG) monitoring may be particularly useful (65,66) and is recommended for 48 to 72 hours. Continuous monitoring of EEG should also be done in patients who clinically deteriorate or develop worsened ICP or brain oxygenation without an obvious explanation.
Based on large randomized control trials, prophylaxis with phenytoin has been shown to be effective in reducing early, but not late, PTS (67). Similar reductions in PTS have been found with both valproic acid (68) and levetiracetam (69), though mortality was higher in those treated with valproic acid. However, while these seizures can precipitate adverse effects in the acute phase by increasing cerebral metabolic rates, studies have shown that early PTS did not significantly influence neurologic recovery at 6 months (63). Thus, routine use of anti-seizure prophylax for PTS is controversial and is recommended only for one week to prevent early seizures. If it is used, it should be discontinued after 7 days if there is no evidence of a seizure.
Maintain Brain Oxygenation
Oxygen delivery to the brain is dependent on CBF as well as the oxygen content of the blood. Thus, while there has been no strong clinical evidence directly linking hypoxemia with increased morbidity or mortality, clinical intuition would suggest that TBI patients who also have episodes of hypoxemia should have poorer outcomes. While some studies have shown worse outcomes with early hypoxemia (70,71), at least one study has shown no relationship (72). In general, however, current guidelines recommend avoiding hypoxemia, which is typically defined as an oxygen saturation of less than 90% or a PaO2 of less than 60 mmHg. As most oxygen content in the blood is bound to hemoglobin, avoiding anemia, common in TBI patients, should conceptually be beneficial in maintaining brain oxygenation. However, while there are data that transfusion improves brain oxygenation in some patients (73), a recent randomized control trial showed no significant benefit in outcome if hemoglobin values were kept greater than 10 g/dL versus 7 g/dL (74).
Maintain Cerebral Perfusion Pressure
Several studies have shown poorer outcomes in the TBI patient with episodes of early hypotension (26–28). As such, initial recommendations are to avoid hypotension, as characterized by systolic blood pressure of less than 90 mmHg. Once an ICP monitor is in place, further blood pressure management should be aimed at maintaining a CPP of at least 50 mmHg, and preferentially around 60 mmHg (45). Increasing CPP higher than 70 mmHg increases the risk of ARDS and does not improve outcome (45,75). Boluses of intravenous fluid can be used as initial treatment of low CPP. All intravenous fluids given should at least be isotonic to avoid worsening cerebral edema and there are data showing improved results with hypertonic solutions (31). If fluid resuscitation is not sufficient to maintain CPP, treatment with vasopressors should be initiated with norepinephrine, which has been shown to be more efficient in augmenting CPP than dopamine (76).
First-line Therapies to Treat Intracranial Hypertension
When ICP becomes elevated despite measures to remove exacerbating factors, the first-line therapies include sedation and neuromuscular blockade, osmotic therapy, CSF drainage, and hyperventilation.
Sedation and Paralysis. Minimizing agitation and/or stimuli that may contribute to elevations in ICP through coughing, controlled ventilation resistance, or posturing can be accomplished with sedative and/or NMBAs. However, the routine use of these medications in patients with severe head injury is known to increase the rate of complications and prolong the ICU stay (77). As a result, they should be selectively used in carefully chosen patients. For example, propofol is a good temporary option for sedation and, indeed, several studies have shown no significant changes in ICP with propofol infusion (78,79). However, propofol dosages of greater than 5 mg/kg/hr or any dose for longer than 48 hours should be avoided as it raises the risk of propofol infusion syndrome which is characterized by hyperkalemia, metabolic acidosis, rhabdomyolysis, renal failure and, possibly, death (80). Alternatively, infusions of morphine (2–10 mg/hr), fentanyl (100–200 μg/hr), or sufentanil can also be used for analgesia. A randomized control trial has shown similar outcome results to propofol with morphine infusion (81). While boluses of fentanyl or sufentanil have been shown to cause an elevation in ICP (82), (83), this can be avoided with slow titration (84). In patients in whom an NMBA is needed, cisatracurium has been shown to cause no significant changes in ICP when used in conjunction with sufentanil and midazolam, the latter agents for continuous sedation (85), and is the preferred neuromuscular blocker. Since the neurologic examination cannot be closely monitored while the patient is pharmacologically paralyzed, these medications should be withheld once a day, usually before morning rounds, to obtain a brief neurologic examination.
Osmotic Therapy to Reduce Intracranial Pressure. One of the mainstays of treatment of elevated ICP is osmotic therapy with either mannitol or hypertonic saline. Mannitol can be given in a single dose of 1 to 1.5 g/kg for short-term effect, particularly in patients without an ICP monitor who have clinical signs of active brain herniation (86). Once an ICP monitor has been inserted, it can also be used for prolonged therapy for patients with persistently elevated ICPs greater than 20 to 25 mmHg, either with intermittent boluses between 0.25 and 0.5 g/kg every 2 to 3 hours or with a continuous infusion, however there is little clinical evidence regarding this approach (87). The serum osmolality in either approach should be monitored and kept less than 320 mOsm/kg H2O. Dosing to a serum osmolality of greater than 320 has not been demonstrated to improve outcome and increases the risk of acute renal failure (88).
Although mannitol has been more widely studied, some studies have suggested that boluses of 7.5% hypertonic saline are more effective at lowering ICP (89). Other variations of hypertonic saline that have shown improvements over mannitol include hypertonic saline/hetastarch (90) and hypertonic saline/dextran (91). However, further large randomized controlled studies are necessary to define the role of hypertonic saline in the treatment of increased ICP.
Drainage of Cerebrospinal Fluid. Placement of a ventriculostomy catheter is a mainstay of therapy for hydrocephalus and allows for drainage of CSF during episodes of increased ICP. In cases where ICP remains refractory to both medical therapy and ventriculostomy, additional lumbar drainage is emerging as a potential secondary source for CSF drainage (92,93).
Hyperventilation. Hyperventilation is a temporizing measure to reduce ICP that works by inducing cerebral vasoconstriction. Clinically, it is also known to reduce CBF (94). Thus, in TBI patients who already have lowered levels of CBF (95), aggressive hyperventilation risks precipitating ischemia and, indeed, randomized control trials show poorer outcomes in patients who receive prophylactic hyperventilation (96). As a result, current guidelines do not recommend prophylactic hyperventilation, as defined by PaCO2 levels of 25 mmHg or less.
However, far less is known about the effects of moderate hyperventilation, as characterized by PaCO2 values between 30 and 35 mmHg, and in general this is considered a possible treatment strategy for patients with refractory ICP. It is important to withdraw chronic hyperventilation over several days as abruptly returning the PaCO2 to normal can result in a dramatic increase in ICP.
Additional Treatments for Refractory Intracranial Hypertension. All of the treatments outlined in this category have been shown in clinical studies to significantly reduce otherwise refractory ICP. However, none has demonstrated improved outcome. There are also no data to suggest which of these treatments is most effective or has the least morbidity. For these reasons, they are applied selectively to patients who are judged to have some potential for recovery if their ICP can be controlled. However, if ICP remains refractory, it is also important to consider whether a delayed intracranial hematoma may have developed. A follow-up CT scan may be indicated before advancing to these additional treatments and any surgical intracranial hematoma should be evacuated.
Pentobarbital Coma. The administration of pentobarbital coma has been shown in a randomized multicenter control trial to double the likelihood of ICP control in otherwise refractory patients (97). However, given its strong side effect profile, most notably for causing significant hypotension—which can significantly influence outcome (98)—its use should be restricted to those who are truly refractory to other methods. It has also been shown ineffective as a prophylactic treatment for all severe TBI patients with a poor examination (99), or as a first-line treatment for elevated ICP (100) and can be detrimental in these situations.
A simple paradigm for induction is a loading dose of 10 mg/kg intravenously (IV) over 30 minutes followed by 5 mg/kg/hr for three doses followed by a maintenance dose of 1 mg/kg/hr (97). The initial loading dose should be given by slow intravenous push with close monitoring of the blood pressure. While this dosing regimen is based upon titrating the maintenance dose to achieve a serum level of 3 to 4 mg/dL (30–40 μg/mL), there is poor correlation between serum levels of pentobarbital and benefit. Instead burst suppression on continuous electroencephalographic recording is a more accurate indicator of effect (101).
The goal of treatment is to reduce ICP to below 20 to 25 mmHg. After ICP has been controlled for 24 to 48 hours, pentobarbital can be weaned and stopped; if ICP increases during reduction of pentobarbital, stop the downtitration and increase the dose until ICP is controlled. It should be noted that in addition to risk of hypotension, which should be closely monitored and corrected with fluid boluses and vasopressors as needed, pentobarbital coma also increases the risk of hospital-acquired complications, including pneumonia, pressure ulcers, and paralytic ileus. Given this side effect profile, several studies have tried to identify the subgroup of TBI patients most likely to respond to pentobarbital coma. Some characteristics that have been identified include younger age, diffuse rather than focal brain injury, and a high pretreatment level of brain electrical activity (102). Other factors associated with a positive outcome include a lack of overwhelming injuries that would drastically reduce the cerebral metabolic rate and a lack of significant hypotension that would limit the amount of barbiturate that can be given (103). Preservation of the cerebrovascular reactivity to CO2 has also predicted a good ICP response to barbiturates (104).
Decompressive Craniectomy. This therapeutic modality has a long and controversial history since its initial description by Kocher in the early 20th century. Indeed, its efficacy in trauma has been questioned for several issues, including early studies debating its usefulness in the evacuation of subdural hematomata (105,106) and more recent studies debating the effectiveness in managing refractory ICP (107,108). A recent multicenter randomized control trial has demonstrated that early decompressive craniectomy does decrease ICP, but is associated with more unfavorable outcomes (109). However, the patients in this trial only needed elevations in ICP of greater than 20 mmHg for 15 nonconsecutive minutes in a 1-hour period to be included. The results from patients with longer elevations in ICP is currently being evaluated in another multicenter randomized trial (110). In general, craniectomy for refractory ICP management in patients without an underlying surgically addressable lesion is only considered when CSF drainage, osmotic therapy, hyperventilation, and sedation have failed and ICP remains elevated for an extended period of time.
When performing a decompressive craniectomy, multiple technical considerations must be considered (111). Most notably in these considerations is the location. For example, if the injury primarily involves a single hemisphere of the brain, a hemicraniectomy in which a large bone flap over one hemisphere is removed with particular focus paid to visualization of the middle fossa floor and anterior temporal lobe can provide adequate decompression. Diffuse injury involving both hemispheres may necessitate bifrontal craniectomy, with decompression of both temporal and frontal lobes. Similarly, the size of the craniectomy has also been shown to significantly affect the degree of ICP reduction (111,112), with studies showing very poor outcomes with sizes less than 10 cm (112).
Hypothermia. This therapy has, conceptually, several potential neuroprotective effects, including reducing cerebral metabolism and decreasing ICP. However, the results from randomized control trials have been mixed, with some studies showing a positive benefit on outcome (113–116) and at least one large study showing no benefit (117) despite a reduction in ICP. Part of this difference in results might be secondary to differing levels of hypothermia and differing lengths of treatment in each trial. As a result, while avoiding hyperthermia (>38°C) is recommended, routine induction of hypothermia is not recommended, but it may be an effective adjunctive treatment for increased ICP refractory to other treatments.
Contraindicated Therapies (Therapies with No Beneficial Effect)
There is no demonstrated benefit in treating TBI patients with steroids. A multicenter randomized control trial was halted early after an observed increased risk of death in patients receiving methylprednisolone for 48 hours after injury (118). As a result, steroids are contraindicated for treatment of head injury. However, they can be given for other indications in TBI patients, including hypothalamic/pituitary dysfunction or a prior outpatient condition requiring steroid use, such as asthma.
Completion of Treatment
Treatment should be titrated off once ICP has been controlled below 20 mmHg for 24 to 48 hours. ICP monitors can be removed if ICP is stable below 20 mmHg for 24 to 48 hours after therapy has been stopped. It is important to remember that patients may develop delayed increase in ICP secondary to blossoming of contusions, evolution of a stroke, or development of new mass lesions.
CONTROVERSIES
Benefit of ICP Monitoring?
No study clearly demonstrates that monitoring and treating ICP improves neurologic outcome. On the one hand, there are strong associations between intracranial hypertension and a poor outcome, and the development of refractory intracranial hypertension has a very high mortality rate (26,37–39,97,100). Historically, institution of treatment protocols aimed at controlling elevated ICP has significantly improved outcome in TBI. For example, in 1977 Jennett et al. (119) reported a mortality rate of 50% in comatose patients (GCS <8) in a cohort treated without ICP monitoring. By way of contrast, Becker et al. (120) reported significantly lower mortality of 30% in a similar patient cohort in whom ICP monitoring was used. Additionally, mortality rates at 30 days for patients with severe brain injury have been slowly declining with an increase in treatment strategies designed to control ICP. The mortality of patients in the Traumatic Coma Data Bank decreased from 39% in 1984 to 27% in 1996 (121). On the other hand, the recent multicenter randomized control BEST-TRIP trial comparing ICP monitored care to care based on clinical and imaging without ICP monitoring found no difference in survival, functional status at 6 months, or neuropsychological outcome at 6 months (122).
The availability of multiple monitoring modalities has provided more information on the status of patients in whom poor outcomes develop, and has provided treatment goals. Treatment strategies are aimed at maintaining ICP less than 20 to 25 mmHg, maintaining CPP greater than 60 mmHg, and maintaining brain tissue oxygenation at greater than 10 mmHg. Even with these advances in treatment, TBI is still associated with significant morbidity and mortality in both the short and long term.
What Is the Most Important Physiologic End Point: ICP, CPP, or Brain Oxygenation?
As it has become possible to measure additional brain-specific physiologic parameters in the ICU, different management strategies have evolved that place special emphasis on parameters other than ICP. For example, some have advocated that ICP is not important as long as CPP is maintained. This philosophy led to the use of CPP-directed therapy where induced hypertension was used to drive CPP to high levels even though ICP was also increased by the therapy (55). However, all of these physiologic parameters are related to outcome, and there is no clear evidence that one parameter is more important than the others. Table 118.1 presents normal values and treatment thresholds for these parameters.
TABLE 118.1 Normal Values and Treatment Thresholds for Physiologic Parameters | ||
![]() |

Full access? Get Clinical Tree
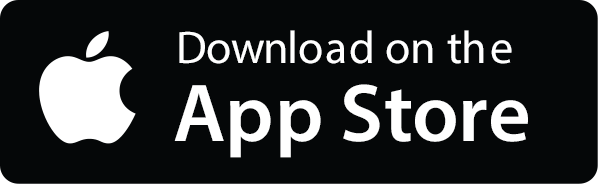
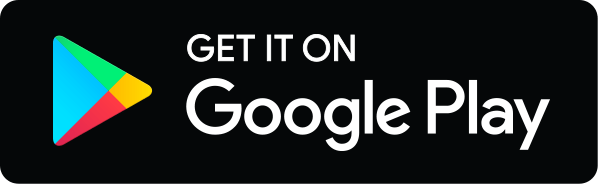