Peter Monteleone1, Peter Pollak2, David Carlberg3, and William J. Brady4 1 Department of Internal Medicine, University of Texas at Austin Dell School of Medicine, Austin, TX, USA 2 Mayo Clinic Specialist, Jacksonville, FL, USA 3 Georgetown University School of Medicine, Washington, DC, USA 4 Departments of Emergency Medicine and Medicine, University of Virginia School of Medicine, Charlottesville, VA, USA Ischemic and infarcted myocardium presents electrocardiographically in many different ways. Acute coronary syndrome (ACS) may present with an array of findings, ranging from the normal electrocardiogram (ECG) to obvious ST segment elevation myocardial infarction (STEMI) to a malignant dysrhythmia. In fact, ECG changes in almost any portion of the ECGs tracing can be seen with ACS and, in the appropriate setting, suggest the diagnosis. Unfortunately, these changes do not always manifest themselves in a standard, “textbook” manner. The clinician must remember that each 12‐lead ECG or cardiac rhythm strip is quite literally an “electrical snapshot in time,” a few seconds in the progression of the heart’s ischemic state. The use of serial ECGs with or without continuous cardiac monitoring is suggested in situations where a clinical suspicion of ACS is present. This chapter reviews and discusses the 12‐lead ECG findings encountered in patients with a range of coronary syndromes, ranging from stable angina to STEMI, with or without malignant dysrhythmia. Of the different types of ACS, STEMI is the most likely to demonstrate a predictable evolution of its ECG findings. The earliest ECG abnormality associated with STEMI, occurring within minutes of an interruption to myocardial blood flow, is the development of hyperacute, or prominent, T wave. In a normal ECG, the orientation of the T wave typically corresponds to the dominant direction of the associated QRS complex; in other words, if the QRS complex is largely positive, then the T wave will also be positive or upright and vice versa. The T wave is typically upright in leads I, II, and V3 through V6; inverted in leads aVR and V1; and variable in leads III, V2, aVL, and aVF. In the early stages of coronary artery occlusion, the T waves maintain their vector (i.e. do not invert) but become tall and peaked – thus the hyperacute T wave. Refer to Figure 13.1 for examples of hyperacute T waves in early STEMI. Classically, hyperacute T waves progress directly to ST segment elevation (STE). STE itself is both an alarming and demanding ECG finding, particularly in a patient with chest pain or chest pain equivalent presentation. It is both alarming and demanding in that it can be the manifestation of STEMI, a time‐sensitive myocardial infarction with significant risk for morbidity and mortality if therapy is not delivered appropriately and expeditiously. As per the American Heart Association (AHA), STE must reach at least 1 mm of magnitude in the limb leads and 2 mm in the precordial leads to meet criteria for the diagnosis of STEMI; these findings must also occur in at least two leads in the same anatomic distribution to suggest STEMI – assuming the clinical correlation is present. While the ST segment in a normal ECG is typically at the same level as the TP segment (i.e. not elevated above the electrocardiographic baseline defined by adjacent TP segments), during STEMI the ST segment changes from this flat and/or concave morphology to one which is elevated with either a convex or an obliquely straight morphology (Figures 13.2a, b and 13.3a, b). As the infarction progresses, the QRS complex, ST segment, and T wave fuse, leading to a single monophasic deflection on the ECG. Given its characteristic appearance and grave prognosis if left untreated, this finding has been named “the tombstone” form of STE. Refer to Figure 13.3a–e for an example of the evolution of the ECG in STEMI. Figure 13.1 (a) Hyperacute, or prominent, T waves of early STEMI. (b) Hyperacute T wave with early ST segment elevation in the anterior region, consistent with early anterior STEMI. Several features of STE are important to consider. The magnitude of STE is important. As noted, expert consensus groups such as the AHA suggest that 1–2 mm of elevation is required to establish the diagnosis of STEMI. The clinician is cautioned, however, in that minimal degrees of STE, that is, subtle STE, can be encountered in certain STEMI presentations, including both inferior and lateral infarctions as well as early in the patient’s course of acute myocardial infarction (AMI) (Figure 13.4). The morphology, or contour, of the elevated ST segment is also important to consider (Figure 13.2a–d). Typically, the elevated ST segment is either obliquely straight or convex in shape. Concave‐shaped, elevated ST segments are less often associated with STEMI. Of course, there is an exception to every such statement – early in the course of STEMI, the elevated ST segment can be concave in appearance with evolution ultimately to either obliquely straight or convex morphologies as the infarction progresses. Regarding these electrocardiographic features of STEMI, common pitfalls in the ECG interpretation include the missed diagnosis of STEMI when the magnitude of the elevation is either minimal (i.e. less than 1 mm) or atypical in contour (i.e. concave in shape). The clinician is encouraged to consider the ECG within the context of the overall patient presentation. Figure 13.2 ST segment elevation subtypes in STEMI and non‐STEMI presentations. (a) STEMI with obliquely straight ST segment elevation. (b) STEMI with convex ST segment elevation. (c) non‐STEMI (BER) with concave ST segment elevation. (d) non‐STEMI (acute pericarditis) with concave ST segment elevation. While pathologic Q waves, defined as the initial downsloping, or negative portion, of the QRS complex, may develop within one to two hours of symptom onset, they usually require as long as 12–24 hours after the event to fully develop (Figure 13.5a). Since nonviable cells do not conduct an electrical signal, Q waves are the ECG indication of myocardial cell death, representing an electrically inert myocardium. Clinicians must be aware, however, that the appearance of Q waves does not exclude ongoing infarction. A subset of patients with Q waves present on their ECGs in the presence of a STEMI pattern may still benefit from fibrinolytic therapy or percutaneous coronary intervention (PCI) (Figure 13.5b). Within 48 hours after an untreated STEMI, the ST segments will usually return to their baseline and the T waves should flatten and then symmetrically invert. Within days to months, the T waves will often revert to their normal upright conformation. The Q waves may disappear over months, though they will often become a permanent feature of the patient’s ECG. Refer to Figure 13.3a–e for the evolution of ECG changes with STEMI. Patients with STEMI frequently develop concomitant ST depression on ECG. Reciprocal change, also called reciprocal ST segment depression, is defined as at least 1 mm of ST segment depression occurring on an ECG which also demonstrates STE (Figure 13.6a, b). Further, the concept of reciprocal change cannot be used in ECG syndromes in which ST segment depression is encountered as part of that entity’s normal or defined appearance. Thus, with left bundle branch block (LBBB), left ventricular hypertrophy (LVH), and ventricular paced patterns, ST segment depression is, in fact, anticipated; therefore, both the term and concept of reciprocal change cannot be used. Reciprocal change most commonly occur in leads I and aVL, although they can occur in any lead. Reciprocal change is most commonly detected with inferior STEMI, associated with 70% of these events. Reciprocal change is only detected in 30% of anterior STEMI presentations. While not always present, reciprocal change has an important confirmatory value in STEMI diagnosis. The presence of reciprocal changes portends a 90% positive predictive value for STEMI, that is, 90% of patient with reciprocal changes are, in fact, having a STEMI. Also, the presence of reciprocal change is associated with greater cardiovascular risk as compared to STEMI patients without such abnormalities. The conducting system of the heart is divided into two parts, the left bundle and the right bundle, just distal to the atrioventricular (AV) node. The blood supply to the left bundle comes off the left anterior descending artery (LAD); therefore the left bundle may be damaged with the occlusion of the LAD. The presence of an LBBB complicates the ACS presentation in many ways. First, from a diagnostic perspective, the presence of an LBBB pattern confounds the ability of the ECG to detect changes associated with ACS. In other words, a patient may experience AMI and demonstrate the anticipated ECG findings of a “normal” LBBB. Second, the presence of LBBB is a marker of significant increased cardiovascular risk. Patients with LBBB have higher rates of malignant dysrhythmia, cardiogenic shock, and death. Finally, the presence of a new, or “not known to be old,” LBBB in the setting of acute chest pain or other anginal equivalent presentation is an indication of either fibrinolysis or PCI; of course, this last statement assumes that the clinical presentation is consistent with AMI. Patients have an LBBB when the QRS duration exceeds 120 ms (Figure 13.7); the QRS complex is largely negative in leads V1–V3 and positive in leads I, aVl, V5, and V6. The LBBB is associated with STE with upright T waves in leads V1 through V3 and ST segment depression with inverted T waves in the lateral leads I, aVl, V5, and V6. The relationship of the QRS complex to the ST segment position is described by the concept of appropriate discordance; this concept states that the major, terminal portion of the QRS complex and the ST segment are located on opposite sides of the electrocardiographic baseline. Thus, primarily positive QRS complexes are usually encountered with ST segment depression and termed discordant ST segment depression; primarily negative QRS complexes are seen with STE and termed discordant STE. Refer to Figures 13.7 and 13.8 for examples of appropriate ST segment changes in LBBB. Figure 13.3 Evolution of ECG abnormalities in STEMI. The ECG structures in (a) and (b) demonstrate the typical progression of changes encountered in a STEMI. The ECG structures seen in (c–e) describe the three possible progressions of STEMI, including an aborted STEMI without significant, lasting injury, a completed STEMI with significant, lasting injury and LV aneurysm, and a completed STEMI with significant, lasting damage to the heart, respectively. (a) Progression from normal ST segment and T wave configurations to the following ECG phases of STEMI evolution: hyperacute T wave, early ST segment elevation, progressive ST segment elevation, and the giant R wave (combination of hyperacute T wave and ST segment elevation). (b) Established ST segment elevation in STEMI. (c) An aborted STEMI, either due to endogenous fibrinolysis or medical intervention, with resolving ST segment elevation, eventual T wave inversion, and ultimate T wave flattening with continued presence of the normal QRS complex (i.e. no loss of the R wave), indicating the infarction likely did not produce lasting, significant damage. (d) Completed STEMI with established infarction (indicated by the Q wave) and LV aneurysm (indicated by the persistent ST segment elevation). (e) Completed STEMI with established infarction (indicated by the Q wave and T wave inversion); note that the ST segment elevation has resolved. More than 25 years ago, Sgarbossa and colleagues identified three independent electrocardiographic predictors of AMI in the presence of LBBB, including: (i) STE of at least 1 mm that is concordant with the QRS complex; (ii) ST segment depression of at least 1 mm in lead V1, V2, or V3; and (iii) STE of at least 5 mm that is discordant with the QRS; these findings were assigned weighted probability scores of 5, 3, and 2, respectively (Sgarbossa et al. 1996). To ensure the accuracy and using a specificity of 90% in AMI diagnosis, Sgarbossa and colleagues required a score of at least 3 to confirm the electrocardiographic presence of AMI. If an ECG features only discordant STE of 5 mm or more but neither of the other two criteria, further testing is recommended before one can conclude that the ECG is indicative of AMI. Stated another way, concordant ST segment changes (either elevation or depression) are strongly suggestive of electrocardiographic AMI; conversely, excessive discordant STE greater than 5 mm was weakly suggestive of electrocardiographic AMI. Thus, the third criterion, discordant STE greater than 5 mm, represented a significant limitation to the Sgarbossa decision rule when assessing the ECG for the electrocardiographic presence of AMI. This shortcoming was based primarily on the absolute nature of the third criterion, noting that greater than 5 mm of discordant STE was suggestive of AMI; it did not account for the proportional relationship of the ST segment to the QRS complex – basically, the magnitude of the discordant STE to the amplitude of the QRS complex. Figure 13.4 Subtle STEMI. (a) Subtle ST segment elevation in an inferior STEMI. Also note the reciprocal ST segment depression (reciprocal change) in lead aVl. (b) Subtle STEMI of the lateral wall with ST segment elevation in leads V5 and V6. Figure 13.5 (a) Q wave likely manifesting a completed myocardial infarction. (b) Q wave with significant ST segment elevation likely representing an acute (i.e. recent) STEMI. This patient is likely still a candidate for fibrinolysis or PCI. Correlation with the patient’s history is strongly encouraged in such cases to determine the onset of the STEMI.
13
Electrocardiographic Findings in Acute Coronary Syndrome
Introduction
The 12‐Lead Electrocardiogram in ST Segment Elevation Myocardial Infarction Evolution of Electrocardiogram Abnormalities
Reciprocal Change
Left Bundle Branch Block

Full access? Get Clinical Tree
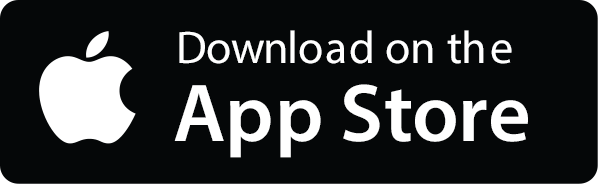
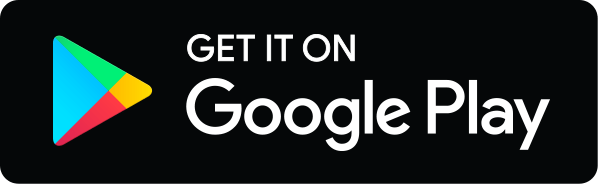