Systemic inflammatory response syndrome (SIRS) criteria
Two or more of the following criteria must be present:
•Body temperature less than 36°C or greater than 38°C
•Heart rate greater than 90 beats per minute
•Tachypnea, with greater than 20 breaths per minute; or, an arterial partial pressure of carbon dioxide less than 4.3 kPa (32 mmHg)
•White blood cell count less than 4,000 cells/mm3 (4 × 109 cells/L) or greater than 12,000 cells/mm3 (12 × 109 cells/L); or the presence of greater than 10% immature neutrophils (band forms)
To better define the categories of sepsis, severe sepsis, and septic shock with regard to the surgical patient, we have modified the American College of Chest Physician/Society of Critical Care Medicine Consensus Conference definitions. We have defined surgical sepsis as systemic inflammatory response syndrome (SIRS) plus an infection requiring surgical intervention for source control or SIRS plus an infection within 14 days of a major surgical procedure. Major surgical procedure is defined as any procedure requiring general anesthesia for >1 h.
Severe sepsis is defined as SIRS plus infection plus acute organ dysfunction. Qualifications of acute organ dysfunction are defined as follows:
1.
Neurologic: Glasgow Outcome Score (GCS) <13 upon recognition of sepsis or deteriorating GCS to <13 during first 24 h.
2.
Pulmonary: PaO2/FiO2 ratio <250 (<200 if lung is primary site of infection) and pulmonary capillary wedge pressure (PCWP) (if available) not suggestive of fluid overload.
3.
Renal (one of the following): urine output (UOP) <0.5 ml/kg for ≥1 h despite adequate volume resuscitation, increase in serum creatinine ≥0.5 mg/dl from baseline (measured within 24 h of starting sepsis resuscitation) despite adequate volume resuscitation or increase in serum creatinine ≥0.5 mg/dl during first 24 h of sepsis management despite adequate volume resuscitation. Adequate volume resuscitation is defined as a minimum intravenous fluid infusion of 20 ml/kg/ideal body weight (IBW) or central venous pressure (CVP) ≥8 mmHg or PCWP ≥12 mmHg.
4.
Coagulation (one of following): INR >1.5, platelet count <80,000 or ≥50% decrease platelet compared to 24 h before instituting sepsis resuscitation or in the 24 h after starting sepsis resuscitation in the absence of chronic liver disease.
5.
Hypoperfusion: lactate level >4 mmol/l. Septic shock is defined as SIRS plus infection plus acute cardiac dysfunction. Acute cardiac dysfunction is defined by the requirement of vasopressors to increase mean arterial pressure (MAP) ≥65 mmHg despite intravenous fluid (IVF) challenge ≥20 ml/kg/IBW of isotonic crystalloid infusion or CVP ≥8 mmHg or PCWP ≥12 mmHg.
Initial Assessment and Evaluation of the Septic Patient
Early Identification of Sepsis
Within our institution, we have identified sepsis to be a major cause of morbidity and mortality in our general surgery patients. Early signs of sepsis were often missed and interventions were delayed as bedside nurses and other team members focus on multiple priorities and tasks involved with patient care. Many of the early signs and symptoms of sepsis are often subtle and in the surgical population may be attributed to other problems. For example, oliguria is commonly seen in surgical patients and is often attributed to under resuscitation in the operating room or volume loss from the gastrointestinal tract. However, oliguria can also be an early finding in patients with sepsis. Alterations in mental status are often attributed to narcotic administration or ICU psychosis, but can also be an early warning sign off sepsis. Likewise, acute hypoxia on the surgical wards spurs a workup for pulmonary embolism but acute hypoxia may herald the onset of severe sepsis or septic shock.
Identifying patients in the early stages of sepsis is imperative. Progression to septic shock is associated with prohibitively high mortality (>30%) despite aggressive interventions. Considering the adverse outcomes associated with this progression, the benefit of routine, accurate screening of patients for sepsis quickly becomes apparent. In an attempt to increase the early identification of sepsis, we developed a sepsis screening tool for use in our SICU (see Fig. 6.1) [13]. Our initial experience with the implementation of this mandatory sepsis screening tool in our SICU showed promising results. The screening tool yielded a sensitivity of 96.5%, a specificity of 96.7%, a positive predictive value of 80.2%, and a negative predictive value of 99.5%. In addition, sepsis related mortality decreased from 35.1 to 23.3%. Subsequent expansion and statistical validation of the screening on the surgical floor yielded similar results [14]. Since implementing mandatory sepsis screening we have seen a significant decline in our severe sepsis and septic shock related mortality. Regardless of the method utilized to screen patients, all members of the patient care team must be aware and vigilant in the detection of the early signs and symptoms of sepsis.
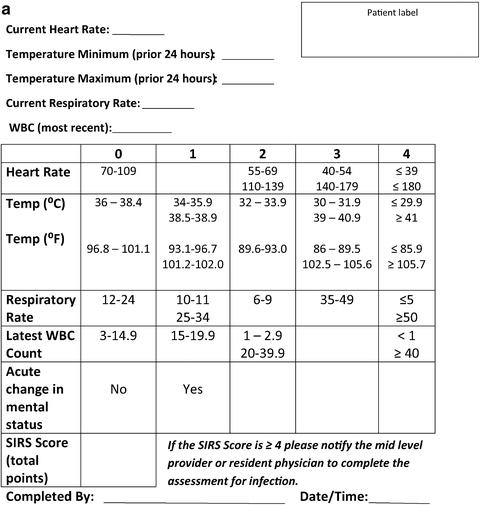
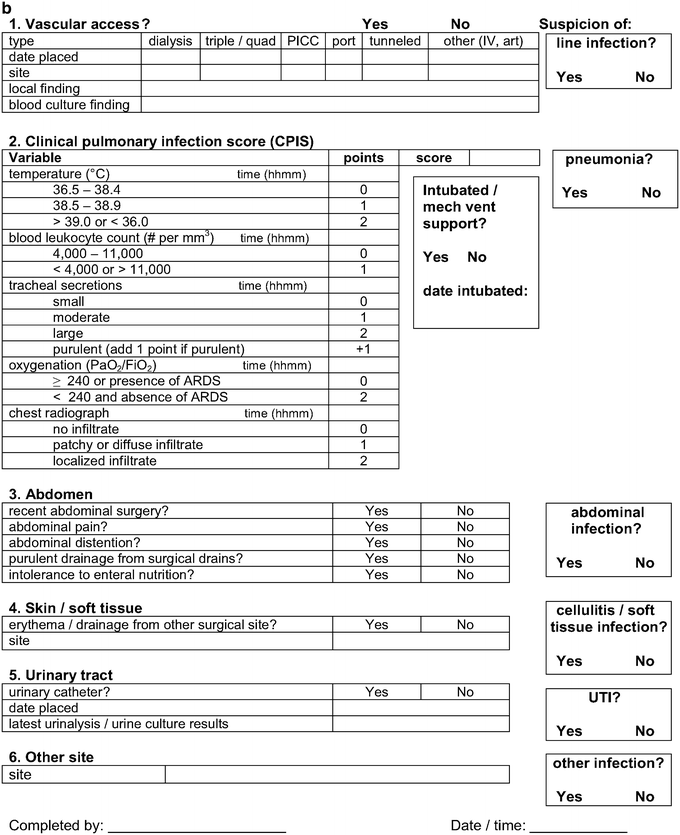
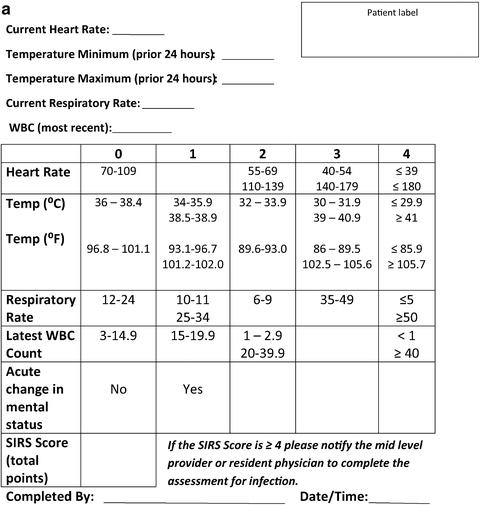
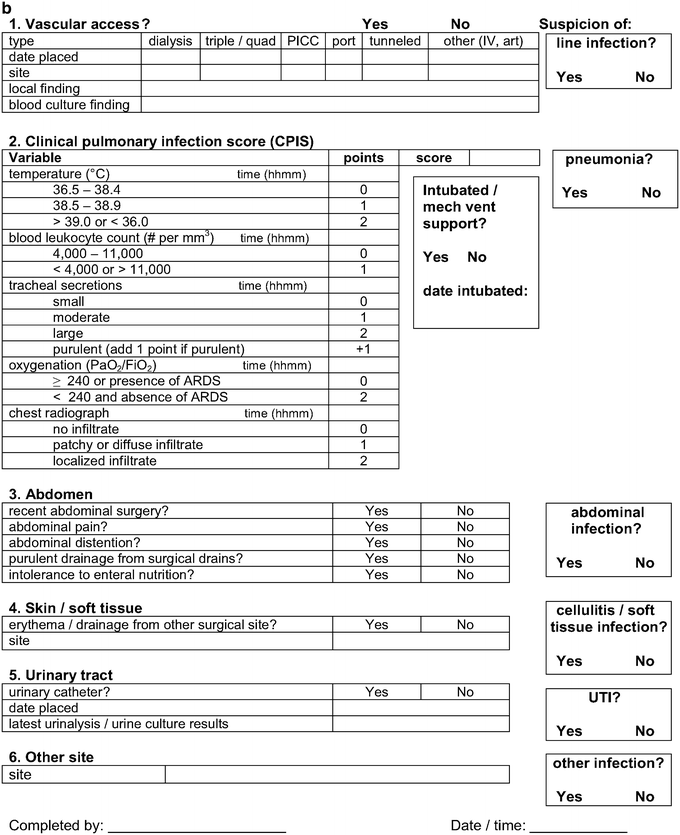
Fig. 6.1
Sepsis screening tool. (a) Sepsis screening score. (b) Midlevel/Physician sepsis screening assessment for source of infection
Initial Assessment
A clinical suspicion for the presence of sepsis should prompt further evaluation of the patient. This initial evaluation should focus on determining the degree of physiologic derangement exhibited by the patient. It is especially important to assess for the presence and degree of tissue malperfusion. There are several clinical and laboratory variables that can be used to evaluate the state of tissue perfusion. The following are indicators that the patient is experiencing tissue hypoperfusion: (1) urine output <0.5 ml/kg of ideal body weight, (2) mean arterial pressure <65 mmHg, (3) Glasgow Coma Score <12, and (4) serum lactate ≥4 mmol/l. The detection of tissue hypoperfusion should prompt aggressive resuscitative measures focused on restoring tissue perfusion. Based upon the definitions outlined previously those patients that do not have evidence of tissue hypoperfusion would fall into the category of sepsis. Those patients that do have evidence of tissue hypoperfusion would be categorized as having severe sepsis/septic shock. The initial resuscitation and management of these patients is discussed as follows.
Initial Resuscitation of Sepsis
The initial resuscitation phase of sepsis should begin immediately upon recognition of sepsis. Initiation of resuscitation should not wait until the patient is transferred to a higher level of care. The goals of the resuscitation include restoration of intravascular volume, diagnosis of the source of infection, initiation of broad spectrum antimicrobial therapy, and source control. Many institutions have developed order sets that specifically address each of these issues. The utilization of standardized protocols for the initial management of sepsis has been demonstrated to improve patient outcomes in multiple settings [15–20].
The major tenets of initial resuscitation can be initiated in any area of the hospital and should not be delayed pending transfer to the ICU. Establishing intravenous (IV) access is a critical first step as this allows for the administration of resuscitative intravenous fluid and antimicrobials. For those patients without evidence of tissue hypoperfusion, a large bore peripheral IV should be sufficient. In the event that peripheral IV access is not attainable, a central venous line should be inserted in a timely fashion.
Fluid resuscitation should be guided with the following goals in mind:
These endpoints of resuscitation should be achieved within 6 h of the recognition of sepsis. In addition a baseline serum lactate should be sent upon the identification of sepsis. A repeat serum lactate level should be sent 4 h later to monitor the progress of the initial resuscitation.
The initial resuscitation fluid of choice still remains extremely controversial. There are no prospective, randomized controlled trials evaluating crystalloid versus colloid resuscitation in surgical patients with sepsis. If colloids are given, the initial fluid bolus should be 300–500 ml of colloid over 30 min. If crystalloids are given, the initial fluid challenge should be 1,000 cm3 of crystalloid over 30 min. The patient’s response to fluid bolus will dictate the need for additional resuscitation. The Saline versus Albumin Fluid Evaluation (SAFE) study randomized nearly 7,000 critically ill patients requiring fluid resuscitation to receive albumin or normal saline, and no difference in mortality was identified. Interestingly, a subgroup analysis of the 1,218 patients with severe sepsis documented that albumin was associated with a trend toward reduced mortality (relative risk of death 0.87; 95% confidence interval 0.74–1.02) [24]. Currently two randomized trials are ongoing to further investigate this finding: (1) Volume Replacement with Albumin in Severe Sepsis (ALBIOS) [25] (Italy) and (2) Early Albumin Resuscitation during Septic Shock (France) [26].
Initial Resuscitation of Severe Sepsis and Septic Shock
For those patients presenting with severe sepsis and septic shock the timely correction of tissue hypoperfusion is critical. The concept of early goal directed therapy (EGDT) in severe sepsis and septic shock was initially developed and validated in the emergency department (ED) setting in a single-center trial [23]. The ED is frequently the point of entry for many septic patients into the hospital. Unfortunately, many of these patients may wait for prolonged periods of time in the ED. The end result is often a delay in the implementation of early sepsis resuscitation.
The implementation of EGDT has been shown to improve survival in patient presenting with severe sepsis and septic shock [18, 23, 27, 28]. The basic principles of EGDT therapy are to recognize tissue hypoperfusion and initiate therapies to reverse global tissue hypoxia by optimizing oxygen delivery. Tissue perfusion can be monitored by measuring mixed venous hemoglobin oxygen saturation (SvO2), central venous hemoglobin oxygen saturation (ScvO2), or peripheral muscle hemoglobin oxygen saturation (StO2). A SvO2 of ≤65%, a ScvO2 of ≤70%, or a StO2 of ≤75% are considered indicators of tissue hypoperfusion. Once tissue hypoperfusion is identified, specific therapies should be instituted to reverse tissue hypoxia by restoring adequate perfusion. The factors affecting oxygen delivery are cardiac output (CO), hemoglobin ([Hb]), and percent arterial hemoglobin oxygen saturation (SaO2). EGDT attempts to restore tissue perfusion by addressing these variables. The evidence-based Sepsis Resuscitation Bundle was established with a goal to accomplish all indicated tasks, 100% of the time, within 6 h of the diagnosis of sepsis was established, and is used to assist with the administration of prompt resuscitation efforts in the treatment of sepsis (Table 6.2).
Table 6.2
Sepsis Bundles: The goal is to perform all indicated tasks 100% of the time within the first 6 h (Sepsis Resuscitation Bundle) or first 24 h (Sepsis Management Bundle) of the diagnosis of severe sepsis
Sepsis resuscitation bundle—(to be started immediately and completed within 6 h) |
---|
•Serum lactate measured |
•Blood cultures obtained prior to antibiotic administration •Broad-spectrum antibiotics administered within 3 h for ED admissions and 1 h for non-ED ICU admissions |
•In the event of hypotension and/or lactate >4 mmol/L: |
–Deliver a minimum of 20 ml/kg of crystalloid (or colloid equivalent) |
–Apply vasopressors for hypotension not responding to initial fluid resuscitation to maintain mean arterial pressure (MAP) ≥65 mmHg |
•In the event of persistent arterial hypotension despite volume resuscitation (septic shock) and/or initial lactate >4 mmol/L (36 mg/dl): |
–Achieve central venous pressure (CVP) of ≥8 mmHg |
–Achieve central venous oxygen saturation (ScvO2) of ≥70%a |
Sepsis management bundle—(to be started immediately and completed within 24 h) |
---|
•Low-dose steroids administered for septic shock in accordance with a standardized ICU policy •Glucose control maintained ≥lower limit of normal, but <150 mg/dl (8.3 mmol/L) |
•For mechanically ventilated patients inspiratory plateau pressures maintained <30 cmH2O |
To restore intravascular volume and enhance cardiac output, an initial crystalloid fluid bolus of 20 ml/kg of ideal body weight is recommended. This fluid bolus can be administered initially through existing peripheral IVs, however, placement of a central venous line for monitoring of CVP is recommended. An arterial line should be placed in patients with hypotension that do not rapidly respond to volume challenge. The use of noninvasive blood pressure monitoring for patients in septic shock often produces inaccurate measurements and should be avoided for titration of vasoactive medications. A Foley catheter should also be inserted to allow for close monitoring of urine output. Bladder pressures should be monitored in patients requiring aggressive volume loading.
The goals of resuscitation remain the same as those listed previously:
In the event that a ScvO2 of ≥70% or SvO2 ≥65% cannot be achieved with restoration of intravascular volume and mean arterial pressure of 65–90 mmHg, red blood cells should be transfused to achieve of hematocrit of ≥30%.
Multiple international randomized controlled trials of early goal-directed therapy for patients with severe sepsis are underway to validate the findings of the single-center Rivers trial. These include ProCESS (Protocolized Care for Early Septic Shock), ARISE (Australian Resuscitation in Sepsis Evaluation), and ProMISe (Protocolized Management in Sepsis). The ProCESS trial will randomize 1950 patients who present to the emergency department in septic shock to three arms: (1) the EGDT Rivers protocol described previously, (2) a less complicated, less invasive protocol using esophageal Doppler monitor and no blood transfusion, and (3) usual care [29]. The ARISE trial will randomize 1,600 patients to EGDT versus standard care and assess 90-day mortality in patients presenting to the emergency department with severe sepsis [30]. The ProMISe trial will randomize 1,260 patients to EGDT versus standard care and assess 90-day mortality in patients presenting to the emergency department with septic shock [31]. Furthermore, an individual, patient data meta-analysis will be performed across the three trials.
Having achieved the goal CVP, the goal MAP, and the goal hematocrit, if there is still evidence of tissue hypoperfusion, inotropic agents should be administered to improve cardiac output. In patients presenting with septic shock, the initial fluid bolus may not restore their MAP to ≥65 mmHg. A repeat fluid bolus of 20 ml/kg of ideal body weight can be given to correct hypovolemia. However, transient vasopressors therapy may need to be initiated, even if volume resuscitation is still ongoing.
Vasopressor Therapy
Septic shock is primarily a vasodilatory shock, associated with a high cardiac output and a low systemic vascular resistance. Therefore, initial vasopressors therapy should be targeted at restoring vascular tone. Both norepinephrine and dopamine are acceptable first line agents for treatment of septic shock, and should be administered through a central venous catheter. Norepinephrine is primarily an α(alpha)-receptor agonist that promotes widespread vasoconstriction and has little effect on heart rate or stroke volume. Dopamine has dose dependent effects on α(alpha), β(beta), and dopaminergic receptors. The initial increase in blood pressure seen with dopamine is related to increasing cardiac output. At higher doses (>7.5 μ[mu]g/kg/min), dopamine does activate α(alpha)-receptors with resultant vasoconstriction.
In patients with septic shock that is refractory to first line vasopressors, the addition of vasopressin may be beneficial. Vasopressin is a stress hormone that has vasoactive effects. The use of vasopressin is supported by recent work by Landry et al. who suggest that in states of septic shock there is a relative deficiency of vasopressin [32]. The administration of vasopressin in this patient population has been shown to improve responsiveness to catecholamines and potentially reduce the amount of catecholamine needed to maintain blood pressure [33].
The Vasopressin and Septic Shock Trial (VASST) randomized 779 patients in septic shock requiring norepinephrine (5 μg/min) for at least 6 h and at least one organ system dysfunction present for <24 h to vasopressin (0.01–0.03 U/min) versus higher dose norepinephrine (5–15 μg/min) [34]. No difference in 28-day or 90-day mortality was identified. In the prospectively defined stratum of less severe septic shock, the mortality rate was lower in the vasopressin group than in the norepinephrine group at 28 days (26.5% versus 35.7%, p = 0.05), which persisted to 90-day mortality (35.8% versus 46.1%, p = 0.04). In a post hoc analysis of the VASST study, it was identified that the combination of low-dose vasopressin and corticosteroids was associated with decreased mortality and organ dysfunction as compared with norepinephrine and corticosteroids [35]. Based on the results of studies to date, clinicians should consider the addition of low-dose continuous infusion vasopressin (up to 0.03 U/min) in individual septic shock patients who despite adequate resuscitation are still requiring high doses of vasopressors.
It is our current practice to initiate a vasopressin drip at a rate of 0.04 U/min in patients requiring norepinephrine infusion at ≥15 μg/min. The dose of vasopressin should not exceed 0.04 U/min because of the possibility of decreased cardiac output and myocardial ischemia at higher doses [36].
While most patients with sepsis initially present with increased cardiac output, a subset of patients will develop myocardial depression from sepsis. The exact mechanism for this reversible myocardial dysfunction is still under investigation. B-type natriuretic peptide (BNP) is secreted in response to stretching of myocardium and is used clinically to assess volume overload and predict death in acute congestive heart failure. More recently, BNP has been demonstrated to be elevated in early septic shock and likewise predict death. We have recently shown that BNP increases with initial sepsis severity and is associated with early left ventricular (LV) dysfunction that in itself is associated with later death. Monitoring BNP in early sepsis to identify occult LV dysfunction may prompt earlier use of inotropes which are not commonly used in early sepsis resuscitation.
For those patients with suspected or known cardiac dysfunction the addition of inotropic therapy is recommended. Dobutamine is the first line agent for treatment of cardiac dysfunction in patients with sepsis. The management of patients with a cardiac component to their shock state presents a unique challenge to the clinician since they require the titration of vasopressors and inotropic agents. In this subset of patients, the utilization of a pulmonary artery catheter can be extremely useful. This allows for the specific titration of vasopressors based upon systemic vascular resistance and inotropic agents based upon cardiac output. There is no evidence to support increasing cardiac index to predetermined supranormal levels.
Steroids in Septic Shock
The use of steroids for the management of septic shock has been debated for several decades. In recent years, the concept of relative adrenal insufficiency in septic shock has received renewed interest. Despite several large clinical trials addressing the issue of steroid use in patients with septic shock, the topic remains controversial. The ongoing debate is primarily surrounding the definition of relative adrenal insufficiency in critically ill patients and the “gold standard” for diagnosing adrenal insufficiency in this population.
It had previously been a common practice to perform a low dose ACTH (cosyntropin) stimulation test on all patients with septic shock as a means to identify those patients with relative adrenal insufficiency. To perform the cosyntropin stimulation test, a baseline serum cortisol is drawn which represents time zero (T 0). The patient is then given 250 μg of intravenous cosyntropin. Subsequent serum cortisol levels are measured at 30 (T 30) and 60 (T 60) minutes after the cosyntropin. If the delta cortisol is ≤9 μg/dl then the patient is considered to have relative adrenal insufficiency and steroids are initiated. Based on the current evidence to date, we now recognize that the ACTH stimulation test is not recommended to identify the subset of adults with septic shock who should receive hydrocortisone. A number of factors interfere with the ACTH stimulation test, and our current diagnostic tests are not accurate. The administration of etomidate causes a temporary suppression of the hypothalamic–pituitary–adrenal axis, resulting in transient adrenal insufficiency. In addition, patients that have received steroids at any time during the previous 6 months should not undergo testing of their adrenal function. Rather, these patients should be empirically initiated on steroid therapy. The current edition (2008) of the Surviving Sepsis Campaign Guidelines recommends that intravenous hydrocortisone should be considered for adult septic shock when hypotension responds poorly to adequate fluid resuscitation and vasopressors. The literature indicates that low dose corticosteroids decrease the time to cessation of vasopressors [37], increase the systemic vascular resistance and MAP [38], and decrease the risk of death [39]. The dose of hydrocortisone should be ≤300 mg/day. We currently give hydrocortisone 50 mg IV every 6 h. The duration of steroid administration also remains controversial. The current recommendation is that steroids be discontinued once vasopressors are no longer required.
Identifying the Source of Infection
Identifying the source of infection is essential to the initial management of sepsis. Whenever possible, cultures should be obtained prior to initiation of empiric antimicrobial therapy. Current recommendations include obtaining a minimum of two blood cultures, including one blood culture from each vascular access device and one blood culture from a peripheral puncture. Additional cultures from other sites (respiratory, urinary tract) and radiographic imaging should be dictated by clinical suspicion. In the surgical population this may include obtaining cultures from surgical drains and performing pertinent imaging to identify an undrained abscess. Despite the importance of source identification, difficulty in the collection of cultures should not generate a significant delay in the administration of antimicrobial therapy.
In order to improve the chances of detecting bacteremia it is crucial to obtain the appropriate volume of blood for the culture medium. Several studies have demonstrated that the volume of blood cultured is the single most important factor in the detection of bacteremia [40–42]. The recommend volume of blood per culture tube is ≥10 ml. Obtaining blood cultures from all vascular access devices along with simultaneous collection of blood cultures from a peripheral site is beneficial in diagnosing catheter related infections. The concept of differential time to positivity has been well described in the literature [43, 44]. Differential time to positivity is defined as the difference in time necessary for blood cultured drawn simultaneously from a peripheral site and a central venous catheter to become positive. The differential time to positivity is considered to be positive if the blood culture that is drawn through the vascular access device becomes positive at least 120 min before the peripheral culture. If a patient has an indwelling vascular access device and the cultures drawn from that device become positive at least 120 min before the peripheral cultures become positive, it is recommended that the device be removed as it is likely infected [43].
Initiation of Empiric Antimicrobial Therapy
Another key component of the initial resuscitation of the septic patient is the administration of intravenous antimicrobial therapy. Antimicrobials should be administered after appropriate cultures have been collected but within 1 h of sepsis recognition. Difficulty with specimen collection should not delay the initiation of antibiotic therapy beyond the 1 h mark. The time to antimicrobial administration has been identified as a critical factor in survival of patients presenting with sepsis. A recent study by Kumar et al. found that each hour in delay of antimicrobials was associated with an average decrease in survival of 7.6% [45]. Delayed administration of antifungal therapy in patients with Candida bloodstream infections was an independent predictor of hospital mortality [46]. Maintaining a supply of commonly used antimicrobials in the ED and ICU can assist in the timely administration of these agents. The Surviving Sepsis guidelines recommend initiation of intravenous broad-spectrum antibiotics within the first hour of recognizing severe sepsis and septic shock.
The selection of antimicrobial therapy should take into account the patient’s history (including drug allergies and recent antimicrobial exposure), suspected source of infection, and hospital specific antibiograms. Within our surgical ICU, our multidisciplinary sepsis team has developed antimicrobial regimens based upon suspected source of infection and the current institution specific antibiogram (see Table 6.3). When choosing empiric antimicrobial therapy, a few general rules should be applied. Chiefly, the initial antimicrobial coverage should be broad enough to cover all potential pathogens. There is substantial evidence that administering inadequate initial antimicrobial coverage is associated with increased morbidity and mortality [47–50]. Any antimicrobial that the patient has recently received should be avoided. Vigilant monitoring of culture data and de-escalation of the antimicrobial regimen based upon culture results and sensitivities will reduce the risk of superinfection and the emergence of resistant organisms.
Table 6.3
Recommendations for source-specific Empiric Antibiotic Selection
Pneumonia | Antibiotic | Regimen |
Community acquired (CAP) | 1. Ceftriaxone + Levofloxacin 2. Aztreonam + Levofloxacin | 1g IV q24h 750mg IV q24h 2gm IV q8h 750mg IV q24h |
Aspiration (not chemical pneumonitis) | Piperacillin / Tazobactam | 4.5g IV q6h |
Ventilator associated (VAP) | ||
Early VAP (<5 day) | 1. Cefepime 2. Ciprofloxacin | 2g IV q12h 400mg IV q12h |
Late VAP (≥5dy; pseudomonas risk: previous hosp or broad spectrum antibiotic exposure; +pseudomonas culture) | 1. Cefepime + Vancomycin + Tobramycin 2. Ciprofloxacin + Vancomycin + Tobramycin | 2g IV q8h 15mg/kg IV q12h 7mg/kg IV 400mg IV q8h 15mg/kg IV q12h 7mg/kg IV |
Catheter related Infections | ||
Catheter-associated urinary tract infection (CAUTI) | 1. Cefepime 2. Ciprofloxacin | 1gm IV q12h 400mg IV q12h |
IV, art cath; bloodstream | Vancomycin | 1gm IV q12h |
Candidemia high risk (TPN, steroid Tx, diabetes, hepatic failure) | Fluconazole | 800mg IV q24h |
Wound/Soft tissue Infections | ||
Necrotizing soft tissue infection (NSTI) | 1. Piperacillin / Tazobactam + Vancomycin + Clindamycin 2. Ciprofloxacin + Vancomycin + Clindamycin | 4.5g IV q6h 15mg/kg IV q12h 900mg IV q8h 400mg IV q8h 15mg/kg IV q12h 900mg IV q8h |
Surgical Site Infection (SSI) | 1. Ertapenem + Vancomycin 2. Ciprofloxacin + Vancomycin | 1gm IV q24h 15mg/kg IV q12h 400mg IV q12h 15mg/kg IV q12h |
Intra abdominal Infections | ||
Pseudomonas -low risk | 1. Ertapenem + Vancomycin 2. Ciprofloxacin + Metronidazole + Vancomycin | 1gm IV q24h 15mg/kg IV q12h 400mg IV q8h 500mg IV q8h 15mg/kg IV q12h |
Pseudomonas- high risk (previous hospitalization or broad spectrum antibiotic exposure; positive pseudomonas culture) | 1. Imipenem / Cilastatin + Vancomycin 2. Ciprofloxacin + Metronidazole + Vancomycin | 500mg IV q6h 15mg/kg IV q12h 400mg IV q8h 500mg IV q8h 15mg/kg IV q12h |
Candidiasis – high risk (TPN, steroid treatment, diabetes, hepatic failure, upper GI perforation + H2 blocker, age ≥ 75, prolonged antibiotic, long term care) | consider Fluconazole | 800mg IV q24h |
Special Considerations | ||
1. indicates preferred therapy 2. alternative for severe β lactam allergy * dosing adjustments should be made if evidence of renal dysfunction * if Vancomycin allergy (not intolerance), then use Linezolid 600mg IV q12hr |
Obtaining Source Control
The final component of the initial resuscitation bundle is identification and control of the source of infection. This can be as simple as removing an infected vascular access device. However, in our experience, in surgical patients the abdomen is the site of infection in ≥50% of cases. These patients often require diagnostic imaging to identify the source and an operative procedure to attain source control. This includes, but is not limited to, emergent debridement of necrotic tissues, abscess drainage, removal of infected vascular access devices, and exploratory laparotomy. In the setting of septic shock, these procedures, although necessary, can present a unique challenge to the surgical team.
The concept of damage control laparotomy (DCL) was first recognized for the care of critically injured trauma patients [51–53]. Damage control is defined as rapid, initial control of hemorrhage and contamination followed by intraperitoneal packing as needed, and temporary abdominal closure. This concept was utilized on those patients that presented with severe physiologic derangements such as coagulopathy, acidosis, and hypothermia. Rather than persisting for hours performing the definitive operation, these patients have their critical surgical issues addressed in an abbreviated manner so they may be taken to the ICU for continued resuscitation. Once the physiologic derangements have been corrected the patient is taken back to the operating room for a definitive surgical procedure. The decision to utilize DCL should not be viewed as a bailout. Instead, it is a deliberate decision to truncate the surgical procedure in order to minimize the time away from the ICU. The decision to perform DCL is often made prior to arriving in the operating room and is based on the severity of the patient’s physiologic derangements at the time of presentation.
The concept of DCL has now evolved to include critically ill patients with surgical sepsis. Like the trauma patient with the lethal triad of acidosis, hypothermia, and coagulopathy, many patients with septic shock present in a similar fashion. For those patients presenting with septic shock and an identified source of infection requiring surgical intervention, the utilization of DCL can be life saving.
The first priority is to initiate resuscitation. The patient needs to undergo preoperative optimization during which time the airway is secured, central venous and arterial lines are placed, volume resuscitation and broad spectrum antimicrobial agents are administered, and if needed, vasopressors are titrated to the appropriate endpoints. Within 6 h the patient is taken to the operating room for emergent laparotomy and potential damage control procedures. The surgeon needs to assess the degree of physiologic derangement early in the operation and if the severe physiologic derangements exist, then the operative interventions need to be abbreviated. The primary aim is to control the source of infection, e.g., resect dead bowel, manage bowel perforations (resection versus primary closure), drain abscesses, and wash out the abdomen. We do not create ostomies and bowel often is left in discontinuity at this first operation. The abdomen is then managed with a temporary abdominal closure device (via a variety of techniques) and the patient is rapidly returned to the ICU where they undergo continued physiologic optimization. This includes optimizing volume resuscitation and mechanical ventilation, correction of coagulopathy and hypothermia, and monitoring for abdominal compartment syndrome. Over the next 24–48 h abnormal physiology is corrected so that the patient can safely return to the OR for a definitive operation and abdominal closure. Septic shock is a formidable metabolic insult and it is very important to provide optimal nutritional support (this often requires combined enteral and parenteral nutrition) and early mobilization to prevent the loss of lean body mass and resultant impaired recovery.
One of the problems with this “damage control” strategy is that in some of the patients the midline abdominal fascia cannot be closed at the second operation because of bowel distention and edema and they require multiple additional laparotomies for definitive abdominal wall closure. The midline fascia is progressively closed with the use of a vacuum-assisted closure (VAC) device. For this technique to work it is important that the bowel not become adherent to peritoneum of the anterior abdominal wall out to the lateral paracolic gutters otherwise the abdomen becomes “frozen” and the fascia cannot be brought to midline. The VAC device actively removes fluid and decreases edema, provides medial tension which helps to minimize fascial retraction and loss of domain, and protects the abdominal contents by providing separation between abdominal wall and viscera, with no fascial damage since it does not require fascial suture placement. Traditionally, abdominal wall defects in these “frozen” abdomens were closed by mobilizing skin/subcutaneous tissue flaps to cover the defect (i.e., accepting a large hernia defect and need for delayed reconstruction) or by bridging the defect with mesh with later split thickness skin grafting once granulation tissue has developed. This is associated with a 20% gastrointestinal fistula rate, which is an extremely morbid complication. Additionally, many of these patients required delayed complex abdominal wall reconstructions. Recently, there has been significant enthusiasm for acute reconstruction with biological mesh. Unfortunately the long term follow up studies show that many of these patients still require delayed hernia repairs of large defects [54]. In our published experience of treating the open abdomen with the VAC device, we achieved primary fascia closure in 87% at a mean 7 days with a 2% fistula rate and no intra-abdominal abscesses [55, 56]. These results are nearly identical to the results reported by Miller et al. from Wake Forest University who taught us how to do this type of closure [57]. More recently, Cothren et al. have reported 100% primary fascial closure rate using a modified VAC device technique [58]. The long term outcomes are not known but in short term follow-up (mean 180 days) ventral hernia was 2.3%. However, as is true with all emergency laparotomies, this rate will without a doubt increase with time but the hernia defects will be small and more easily repaired.

Full access? Get Clinical Tree
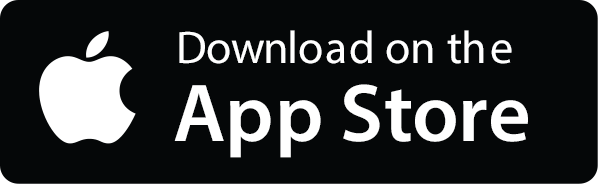
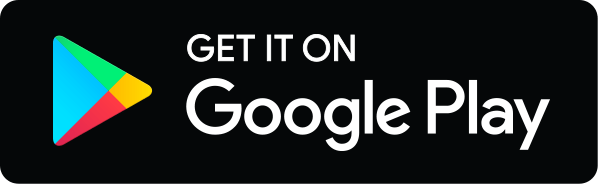