Drowning
Nicholas A. Smyrnios
Richard S. Irwin
Overview
Drowning is the seventh most common cause of unintentional injury death in the United States [1]. In 2005, 3,582 people died from drowning in the United States [2]. The incidence of fatal drowning declined from 2.7 per 100,000 in 1983 to 1.21 per 100,000 in 2005. Drowning is most common in men, children younger than 14 years, Native Americans, and African Americans [2]. The states with the highest drowning rates are Alaska and Mississippi [3]. Statistics on nonfatal drowning are less exact because many nonfatal drowning victims do not seek medical attention. Estimates on the incidence of nonfatal drowning vary widely enough that a definitive statement cannot be made at this time.
The official nomenclature for submersion injuries has changed. Based on the results of a consensus conference held as part of the World Conference on Drowning in 2002, appropriate references to submersion injuries use the terms fatal drowning to describe death by submersion in water and nonfatal drowning to describe at least temporary survival after respiratory impairment from submersion in water [4]. However, some reports continue to use the terms drowning and near-drowning as synonyms for fatal drowning and nonfatal drowning, respectively. In addition, the terms submersion and immersion continue to be used to describe both fatal and nonfatal drowning together.
Etiology and Pathogenesis
The following are considered risk factors for drowning.
Alcohol
Ethanol use is the major risk factor in submersion accidents. Thirty percent to 70% of drownings are associated with alcohol consumption [5,6,7]. Alcohol use seems to be an issue in drowned men in particular [8]. Alcoholic beverages reduce the ability to deal with emergency situations by depressing coordination, increasing response time, and decreasing awareness of stimuli. Furthermore, alcohol consumption by a potential rescuer or by the adult responsible for supervising a child in the water can destroy that person’s ability to function effectively, often resulting in a double tragedy [7]. In addition, alcohol is
frequently a factor in drownings that result from automobile accidents [9].
frequently a factor in drownings that result from automobile accidents [9].
Inadequate Adult Supervision
Children die in water because adults do not supervise them well enough. The backyard pool and family bathtub are common sites of pediatric drowning [10,11,12,13,14]. Lack of appropriate precautions and supervision play a major role in most of these cases. Studies have shown lower rates of drowning in areas where swimming pools are required by law to be surrounded by a fence [15,16]. The fence must completely isolate the pool from unsupervised access by children to be effective. Appropriate sign posting in hazardous areas, effective educational programs on the dangers of water recreation, and the presence of lifeguards also minimize risk and improve survival [10,17,18].
Inattentive guardians also contribute to bathtub-related drownings. In one study, all bathtub-related submersions in children younger than 5 years occurred while the child was bathing unattended or with another young child [19]. The use of infant bath seats, while providing some sense of security to parents, may actually predispose to submersion accidents as the child may slip and become trapped by the seat, making it impossible to escape the water [20]. The practice of leaving infants to bathe in the custody of a toddler is inappropriate and should be discouraged [11,12]. Immersion in large industrial buckets used for home cleaning may also make up a substantial percentage of drownings of infants and toddlers [21].
Child Abuse
Unfortunately, submersion injuries in children are sometimes inflicted intentionally. One study indicated that 29% of all nonfatal pediatric drownings in bathtubs were purposely caused to inflict harm on the child. Another 38% of all nonfatal pediatric drownings revealed evidence of severe neglect [22]. In general, these children are younger than average for submersion injuries, and many have signs of previous abuse on close examination.
Seizures
Drowning is 15 to 19 times more common in people with epilepsy than in the general population [23]. In one study, a history of seizure disorder was found in 17 of the 293 cases of drowning that were reviewed [9]. This contrasts with the prevalence of seizures of 6 per 1,000 in the general population. Poor adherence to anticonvulsant regimens often plays a role. Many drownings of epileptic children occur in the bathtub [24]. Seizures that include a tonic component may be the most dangerous to victims. Tonic seizures include a forced exhalation component that increases body density and causes the victim to sink. When the tonic component relaxes, the negative intrathoracic pressure leads to an inhalation that will then be composed of water [25]. The intensity of supervision needed by epileptics in a water environment is frequently underestimated.
Boating Accidents
Of the 710 boating fatalities in the United States in 2006, 70% were due to drowning [2]. Both alcohol intake and failure to use personal flotation devices contributed to these deaths [9,26]. A blood alcohol level of 0.10 g per 100 mL is estimated to increase the risk of death associated with boating by a factor of 10 [6].
Aquatic Sports
Water-related activities produce approximately 140,000 injuries annually. Diving, surfing, and water skiing account for 77% of the 700 spinal cord injuries produced annually by aquatic sports. Diving and sliding headfirst produce the most serious injuries as a result of striking the bottom or side of a shallow body of water [10]. Patients who experience these injuries are at subsequent risk of drowning. In addition, injuries associated with the use of personal watercraft contribute to drowning incidence [27].
Pathophysiology
General Considerations
Two mechanisms produce the major pathologic changes responsible for morbidity in drowning: anoxia and hypothermia.
Anoxia
Most drownings are thought to follow a common pattern [29]. The drowning sequence begins with a period of breath holding because the victim’s mouth and nose are below the level of the water. This voluntary breath holding is often followed by an intense laryngospasm that prevents breathing. This laryngospasm is usually due to water present in the pharynx or larynx. This prolonged inability to breathe renders the patient hypoxemic and hypercapnic. The laryngospasm eventually abates, followed by involuntary breaths with aspiration of varying amounts of water. In addition, water may be swallowed that is eventually regurgitated and aspirated. Eventually, the victim becomes unconscious and cardiac arrest occurs.
Hypothermia
The impact of hypothermia is complex. Survival after extremely long submersion is generally considered possible only when the victim has been submerged in icy water. There are reports of survival in children submerged up to 66 minutes [30,31,32]. Most authors believe that to achieve such spectacular survivals after long submersions, the core body temperature must be reduced quickly and the brain’s metabolic activity slowed down in equally rapid fashion to prevent hypoxic damage to the brain. Factors that make this more likely in children include the increased relative body surface area, thin layer of subcutaneous fat, and smaller head size. In addition, children may ventilate water earlier in their submersion, and they may retain more water in the upper airway [33]. These factors may also play a role in rapid cooling.
On the other hand, humans tolerate hypothermia poorly. In the most well-known example, the deaths after the sinking of the Titanic occurred not because of inability to float in most cases but because of hypothermia caused by exposure to extremely cold water. Changes in human metabolism in response to hypothermia occur in two phases: the shivering phase and the nonshivering phase. Shivering occurs at a central temperature of 30°C to 35°C. The nonshivering phase occurs below 30°C, when muscle contractions nearly cease and oxygen consumption and metabolic rate decrease.
Shivering and voluntary muscular movements, which in a cold dry environment work together to increase heat production with minimal increase in heat loss, are ineffective in cold water [34]. Both shivering and voluntary muscular movements increase blood flow to the extremities, thereby increasing conductive heat loss. Voluntary movements of the extremities also stir the surrounding water and can increase heat loss from convection [35]. Body type may also play a major role. Obese men tolerate submersion in cold water longer than thin men due to increased insulation from body fat [36]. Water nearly eliminates the insulative function of clothing by replacing the air between the fibers, thereby increasing heat conductance.
Submersion in very cold water can acutely lead to death in three ways. First, a vagally mediated asystolic cardiac arrest may occur (immersion syndrome) [37]. Second, hypothermia produces an increased tendency toward malignant arrhythmias separate from this immediate response. Cardiac arrest from ventricular fibrillation is common at core temperatures below 25°C, and asystole occurs at less than 18°C [38]. These arrhythmias may be refractory to resuscitative efforts until the body temperature has been increased. Third, a decrease in core temperature can cause loss of consciousness and aspiration from the victim’s inability to keep the head above water. This leads to aspiration of water and the sequence of events described previously.
Pulmonary Effects
The effects of aspiration of various water solutions on lung injury have been studied in animals [39,40]. Sterile water was found to be the most disruptive of pulmonary function. Normal and hypertonic saline solutions also cause significant increases in (PA-a)O2 gradient and shunt fraction, with a decrease in the PaO2 to FIO2 ratio. Decreases in arterial oxygen saturation and dynamic compliance as well as increases in minute ventilation, mean pulmonary artery pressure, and shunt fraction are seen in sheep after bilateral aspiration of either fresh- or seawater [40].
On a microscopic level, freshwater and saline solutions may cause their adverse pulmonary effects by different mechanisms. Atelectasis due to increased surface tension, bronchoconstriction, and noncardiogenic pulmonary edema all play a role in the development of hypoxemia at different times after freshwater aspiration [40,41]. Freshwater acts in part by inactivating surfactant in the alveoli and in part by damaging type-II pneumocytes, thereby preventing the production of surfactant for up to 24 hours [42,43]. The combination of these effects may damage the alveolar capillaries and interstitium and lead to the acute respiratory distress syndrome (ARDS). Hypertonic seawater may draw additional fluid from the plasma into the alveoli, thereby causing pulmonary edema despite a decreased intravascular volume [39]. The fluid-filled alveoli are then unavailable for efficient gas transfer, and a ventilation–perfusion mismatch occurs. This fluid may also damage the type-II pneumocytes by hypoxic and osmotic effects [41]. Aspiration of gastric contents and particles in the water complicates both fresh- and saltwater drowning. In clinical practice, the difference in the situation caused by freshwater and saltwater aspirations is small. In both cases, pulmonary edema causes decreased respiratory system compliance and hypoxemia. Unless specific therapies are developed that target the different mechanisms, there is probably little advantage in emphasizing the described differences.
Several other mechanisms of lung injury may occur with nonfatal drowning. Bacterial pneumonia, barotrauma, mechanical damage from cardiopulmonary resuscitation (CPR), chemical pneumonitis, centrally mediated apnea, and oxygen toxicity can cause respiratory deterioration in the postresuscitation period [41]. These must be considered along with ARDS in cases of respiratory distress occurring 1 to 48 hours after the event.
Neurologic Effects
The pathologic effects that most affect prognosis in drowning are related to the central nervous system (CNS). Cerebral injury is produced as a result of anoxia due to gas exchange impairment and subsequent cardiopulmonary arrest.
Anoxic damage begins 4 to 10 minutes after cessation of cerebral blood flow in most situations [44]. The actual time course and clinical significance of anoxia in a specific drowning victim is notoriously uncertain in cases of drowning because of the emotional condition of the witnesses and because the impact of hypothermia is difficult to judge [45].

Full access? Get Clinical Tree
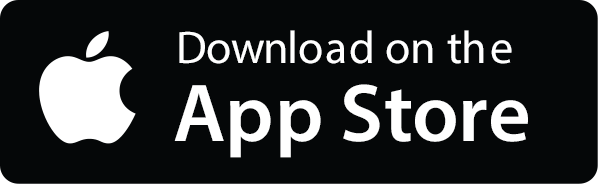
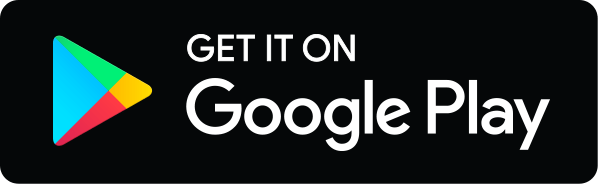