CHAPTER 73
Dorsal Root Ganglion Stimulation: Anatomy, Physiology, and Potential for Therapeutic Targeting in Chronic Pain
INTRODUCTION
Primary sensory neurons (PSNs) process and transmit a multitude of sensory information from the periphery to the central nervous system. In this regard, they are a key component of the initial pathway by which pain (as well as a variety of other sensory information) is transmitted from both peripheral and axial structures to secondary and tertiary order neurons in the central nervous system. In an acute sense, pain transduction serves as a safety mechanism to protect tissues from injury. Classic pain reflexes that result in withdrawal from harmful stimuli protect tissues from damage. Unfortunately, in some cases, these same neurologic pathways can be dramatically altered resulting in the development of chronic neuropathic pain. One component of the sensory nervous system shown to exhibit a number of pathophysiologic changes that may contribute to chronic pain is the dorsal root ganglion (DRG), the home of the primary sensory neuron cell bodies.
ANATOMY OF THE DRG
One of the hallmarks of PSNs is that, anatomically, they are fairly unique in structure. They are considered bipolar or pseudounipolar neurons, in that a single axon exits the soma and divides to form two branches, one of which travels to the periphery and the other to the spinal cord. Positioned between the distal and proximal axons is the T junction. Studies have shown very little variability in DRG position among healthy individuals.
• The soma of the primary sensory neurons are housed in the dorsal root ganglion. There can be up to 15,000 primary sensory neuron soma housed in a single DRG depending upon spinal level (Figure 73-1).
Figure 73-1. Illustration of primary sensory neuron cell bodies within the DRG.
• The soma within the DRG can be large and stretches from distal tissue structures to the spine means it can be meters in length.
• The relative size of the soma compared to the axon is very small.
• Since much of the cell’s protein synthesis occurs in the soma, the metabolic demands on this structure are very high.
• The DRG is typically located in the foraminal space between the medial and lateral edges of the pedicles (Figure 73-2).
Figure 73-2. Lumbar DRGs from coronal MIP imaging. The pedicles are dark (white P) and the DRGs are indicated by the white arrows. Notice the relatively uniform position of the DRGs relative to the pedicles. The vast majority of DRGs from healthy volunteers lie between the medial and lateral borders of the pedicles. (Reproduced with permission from Shen J, Wang HY, Chen JY, Liang BL. AJNR Am J Neuroradiol. 2006 Nov-Dec;27(10):2098-103.)
• There is slight variability in DRG size as DRGs located more caudal tend to be larger.
• Sensory axons maintain a fairly consistent anatomical distribution as they travel to the spinal cord.
• There is an organized and convergent distribution of cellular fibers that eventually synapse in the dorsal horn.
The vascular structure of the DRG provides the metabolic intermediates to support the relatively high metabolic demands of the cells in the ganglion. This vascular structure does not create a classic blood brain barrier due to the porous nature of the structure. To this extent, the ganglion is exposed to blood-borne stimuli and may provide some sort of chemosensory function.
PHYSIOLOGY OF THE DRG
The physiology of the cells of the DRG is thought to be fairly stagnant. In recent years, we have realized that neurons in many different regions of the nervous system are some of the most plastic cells in the body. The primary function of the primary sensory neuron is to conduct action potentials from the periphery to the central nervous system, thereby generating specific sensations.
• Action potentials originating from the periphery may not necessarily invade the membrane of the soma, suggesting that the T junction may act as a filter.
• This allows action potentials to bypass the soma resulting in rapid conduction of action potentials from the periphery to the spinal cord.
• The afferent impulses can originate from the DRG, suggesting that the DRG may be an additional source of sensory input.
• The neuron contains an excitable membrane that allows the passage of selected ionic currents in a specific sequence to generate action potential.
• Disruptions of these currents will not allow the membrane to be sufficiently excitable or it may be hyperexcitable whereby sensations are experienced in the absence of a direct stimulus.
• The changes contributing to the hyperexcitability of DRG neurons are one of the major causes of chronic neuropathic pain.
Animal models have demonstrated that primary sensory neurons exhibit a number of pathophysiologic changes that may contribute to a hyperexcitable state in chronic pain. Both genetic and proteonomic changes that alter the electrophysiological membrane properties of the DRG cells have been observed (Figure 73-3). For example, reduced action potential thresholds, reduced Ca2+ conductance, sodium channel functional alterations, and altered gene expression have been observed. Furthermore, spontaneous action potentials generated in the DRG cell body have been reported. These changes have been correlated with behavioral changes that mimic chronic pain states such as hyperalgesia and allodynia, suggesting a relationship between the pathophysiological alterations and the development of pain.
Figure 73-3. Schematic outlining the pathophysiological changes to the DRG contributing to its hyperexcitable state. (A) Normal sensory pathway and (B) depiction of an altered sensory pathway whereby the primary sensory neurons are hyperexcitable, leading to the generation of neuropathic pain.
CLINICAL TARGETING OF THE DRG
Clinically, the anatomy and physiology of the DRG make it an opportunistic area to modulate nociceptive or pain transmitting neurons. A variety of surgical and nonsurgical techniques have targeted the DRG with varying levels of success. The therapeutic success of this procedure varies and the long-term clinical efficacy remains unclear.
• Dorsal root entry zone lesioning and ganglionectomy are used to denervate selected dermatomes in individuals with chronic pain conditions.
• The limited success of surgical procedures and the occurrence of deafferentation syndromes precluded their ultimate utility and continued use.
• Radiofrequency (RF) targeting of the DRG is a less invasive technique and is used in a clinical setting.
• RF can be either destructive (continuous RF) or nondestructive (pulsed-RF). Conventional RF is controversial in the DRG and has been used more commonly with denervation of the spinal joints.
The DRG is a robust neural structure that can withstand thermal insult and remain robust. The amount of energy that is delivered to the DRG in radiofrequency-based techniques is immense. Yet, very low rates of serious sensory or motor complications arising from this energy delivery have been reported.
Neuroaugmentation of DRG
Limited published data have shown that neural stimulation of the DRG may provide pain relief. Unfortunately, design limitations of current spinal cord stimulator systems preclude the ability to adequately study the potential role of DRG stimulation in the treatment of chronic pain. New systems are currently being developed to address this potentially useful stimulation therapy. These systems consist of novel leads and epidural delivery systems to aid in the placement of leads near the target DRGs (Figure 73-4). More specifically, a new system that has been developed to stimulate the DRG in the treatment of chronic, intractable pain utilizes a slightly different lead and delivery system designed to navigate toward the lateral epidural space and near or about the dorsal root ganglion (Figure 73-5).
Figure 73-4. Spinal modulation lead and delivery system for placement of specially designed leads for DRG stimulation. The lead is housed within a sheath that provides protection and stability for epidural lead placement. The lead can be deployed from the distal end of the sheath to effectively place the lead contacts near the DRG for stimulation.
Figure 73-5. Schematic view of the procedure to place proprietary leads for dorsal root ganglion (DRG) stimulation. (A) Epidural access is obtained using a loss of resistance technique; (B) the lead and delivery sheath is then fed through the delivery needle, after which; (C) the lead is steered through the epidural space toward the DRG; (D) final placement of up to 4 leads for therapy delivery.
• A small, flexible lead contained within a sheath component that provides a lead-sheath delivery combination steering to the lateral epidural space.
• Eventually, the lead deployed in such manner so that lead contacts can provide stimulation therapy.
• Once the leads are positioned near the DRG, stimulation can be tailored via pulse generator programming.
• Similar to other programmable stimulators, contacts are selected as anodes or cathodes and stimulation pulse-waves are adjusted (pulse wave height, width, and frequency) to best capture the painful anatomies.
• Paresthesias are developed and steered toward the painful areas.
• Patients can then control the stimulation therapy with a wireless programmer.
• Lead placement can vary from patient to patient depending upon several factors including differences in spinal anatomy, and desired lead location.
Figure 73-6 depicts flexible epidural needle placement techniques to effectively deliver stimulation leads to the desired DRG. Both ipsilateral and contralateral lead placement techniques are possible to adjacent spinal levels or levels multiple segments away from the epidural access point. Trial procedures are completed similar to other neurostimulator systems to gauge the level of therapy success prior to fully implanting the neurostimulator system. Procedural and postprocedural care is similar to standards typically utilized for fully implantable neurostimulator systems. Adverse event rates are low and comparable to similar technologies.
Figure 73-6. Final lead placements in anterior-posterior (AP; panel A) and lateral (LAT; panel B) views. Panel A: Note the differential epidural needle placement approaches to accommodate varying lead delivery techniques requirements. Both contralateral and ipsilateral needle trajectories can be used to delivery leads one or more levels away from the epidural access point. Panel B: LAT view showing dorsal lead placement within the foramen.
PATIENT SELECTION
DRG stimulation could potentially aid in the treatment of a number of chronic pain conditions such as:
• Discogenic spine pain
• Postherpetic neuralgia
• Chronic foot pain
These conditions have been treated using DRG stimulation and there are likely a number of other indications that would benefit from this therapy, including CRPS and FBSS. The ideal patient characteristics for this type of therapy are outlined below (Table 73-1).
TABLE 73-1. Summary of Ideal Patient Characteristics for DRG Stimulation

Full access? Get Clinical Tree
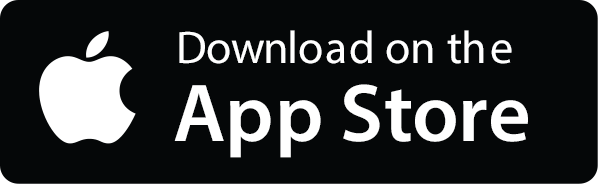
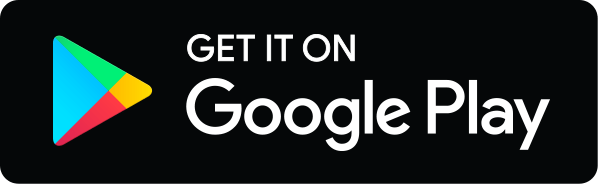