Carbonic Anhydrase Inhibitors
Acetazolamide is the prototype of a class of sulfonamide drugs that bind avidly to the enzyme carbonic anhydrase, producing noncompetitive inhibition of enzyme activity, principally in the proximal renal tubules as well as the collecting ducts (see Fig. 22-1; Table 22-1).3 A Na+-H+ exchanger allows absorption of Na+ in exchange for secretion of H+ into the renal tubule. HCO3− and H+ combine in the lumen of the proximal tubule to produce H2CO3. The enzyme carbonic anhydrase catalyzes the otherwise slow breakdown of H2CO3 into CO2 and H2O; CO2 diffuses readily into the tubular cells, where cytoplasmic carbonic anhydrase catalyses the reverse reaction leading to HCO3−, which then follows an electrochemical gradient across the basal membrane into the interstitium. The net result is absorption of HCO3−. Inhibition of carbonic anhydrase in the proximal renal tubule by this class of diuretics results in decreased reabsorption of Na+, HCO3−, and water.1,3

Pharmacokinetics and Pharmacodynamics
After oral administration, acetazolamide is excreted unchanged by the kidneys. The dose should be adjusted in patients with renal failure and the elderly.4 Acetazolamide completely blocks membrane-bound and cytoplasmic carbonic anhydrase in the proximal tubule and to a lesser extent in the collecting ducts, preventing Na+ and HCO3− absorption.3 This increased excretion of HCO3− results in an alkaline urine and metabolic acidosis. Natriuresis associated with carbonic anhydrase inhibitors is modest, with an increase in fractional Na+ excretion of up to 5%.3 The increased delivery of Na+ to the distal tubules leads to potassium loss. Most of the chloride is reabsorbed in the loop of Henle,3 leading to the excretion of an alkaline urine in the presence of hyperchloremic metabolic acidosis.
Clinical Uses
In addition to its diuretic properties, acetazolamide is administered to decrease intraocular pressure in the treatment of glaucoma. There is a high concentration of the carbonic anhydrase enzyme in the ciliary processes; inhibition of the enzyme activity by acetazolamide results in decreased formation of aqueous humor and consequently a decrease in intraocular pressure.2 Similarly, formation of cerebrospinal fluid is also inhibited by acetazolamide. Accordingly, acetazolamide has been used in the treatment of idiopathic intracranial hypertension.2 Idiopathic intracranial hypertension, previously referred to as benign intracranial hypertension or pseudotumor cerebri, is characterized by increased intracranial pressure (ICP) in the absence of tumors or other causes and manifests with headaches, pulsatile tinnitus, and papilledema and visual changes secondary to the elevated ICP, which can progress to vision loss. Women are more likely to be affected, especially obese women in their third decade of life. When treatment with acetazolamide fails, surgical placement of a ventriculoperitoneal shunt to reduce the elevated ICP is an option. Lumbar punctures, in addition to being diagnostic by providing direct measurement of the elevated ICP, can provide symptomatic relief via removal of cerebrospinal fluid.
Acetazolamide may also be beneficial in the management of familial periodic paralysis because the drug-induced metabolic acidosis increases the local concentration of potassium in skeletal muscles.2 Similarly, acetazolamide, by producing metabolic acidosis, may stimulate the respiratory drive in patients who are hypoventilating in a compensatory response to respiratory alkalosis, as occurs with altitude sickness. Altitude sickness, which can be prevented by a slow acclimatization process, develops following rapid ascent to high altitudes.2 The hypoxia at high altitudes is counteracted by hyperventilation, which leads to respiratory alkalosis, which depresses ventilation. Acetazolamide-induced metabolic acidosis can reverse this hypoventilation.2 Conversely, the loss of bicarbonate ions necessary to buffer carbon dioxide may result in the exacerbation of respiratory acidosis in patients with chronic obstructive airway disease, leading to central nervous system (CNS) depression.
Side Effects
There is a high incidence of systemic side effects associated with the use of acetazolamide such as fatigue, decreased appetite, depression, and paresthesias,4 which could be secondary to the development of acidosis.4 Acetazolamide dose should be reduced in patients with chronic renal insufficiency and avoided in patients with severe chronic renal insufficiency because of the increased risk of metabolic acidosis.2
Loop Diuretics
Furosemide, torasemide, azosemide, bumetanide, and ethacrynic acid are diuretics that inhibit reabsorption of sodium, potassium, and chloride by impairing activity of the Na+-K+-2Cl− transport protein in the medullary portions of the thick ascending limb of the loop of Henle. This area of the nephron is impermeable to water and accounts for the reabsorption of 20% to 30% of filtered Na+.1,2 Because of their site of action, loop diuretics are the most potent diuretics and have a dose-dependent response.2 Diuretics in general and loop diuretics in particular are first-line therapy in patients with fluid retention resulting from heart failure.2
Pharmacokinetics and Pharmacodynamics
Ethacrynic Acid
Ethacrynic acid is no longer in clinical use because of its side effect profile. Ototoxicity, a common dose-dependent side effect of loop diuretics, is more common with ethacrynic acid.2 Nausea is another common side effect.
Furosemide
Furosemide is effective when administered orally or intravenously (IV). However, absorption of orally administered furosemide varies between patients from 10% to 100%, with an average bioavailability of 50%.2,5 Protein binding is extensive, with approximately 90% of the drug bound to albumin. Glomerular filtration and renal tubular secretion account for approximately 50% to 60% of furosemide excretion. The remaining 40% to 50% is conjugated to glucuronide in the kidneys.2,5,6 The elimination half-life is 1 to 2 hours, resulting in the short duration of action. Furosemide has a rapid onset, producing diuresis within 5 to 10 minutes of administration, with a peak effect at 30 minutes and duration of action of 2 to 6 hours. In order to achieve natriuresis, furosemide needs to reach the site of action within the kidneys. In patients with normal renal function, 40 mg of IV furosemide will produce maximal natriuresis.5 Because of decreased drug delivery to the tubule in chronic renal insufficiency, the dosing of loop diuretics should be increased in these patients.2,5 Maximal diuresis can be achieved with an IV bolus of 160 to 200 mg, administered slowly to avoid the occurrence of tinnitus.5 Doses larger than 200 mg will not result in increased natriuresis.5 In addition, in patients with chronic renal insufficiency, a loading dose of furosemide followed by a continuous infusion may be used to achieve a sustained diuresis rather than repeated boluses.1,5 More recently, the Diuretic Optimization Strategies Evaluation (DOSE) trial, a prospective randomized study of the use of loop diuretics in patients admitted with acute heart failure, did not find a significant difference in symptomatic relief or improvement of renal function with the use of high-dose diuretics or with continuous diuretic infusion, compared to low-dose or repeated diuretic boluses respectively.7 The combination of furosemide with a different class of diuretic, such as a thiazide diuretic, may increase the response.5
Bumetanide and Torasemide
Bumetanide has a bioavailability of 80% to 100% after oral administration and can be administered orally, IV, or intramuscularly. It is 40 times more potent than furosemide except in its effect on potassium excretion.2 Similar to bumetanide, torasemide’s metabolism is mostly by the liver,2 and in patients with liver failure, there is increased drug delivery to the kidneys.5 Torasemide is twice as potent as furosemide and has a longer duration of action, with a plasma half-life of 3 to 4 hours5 allowing for a once a day dosing regimen.8
Clinical Uses
Loop diuretics are not the first-line treatment for hypertension in patients with normal kidney function. However, they are first-line diuretics in patients with renal insufficiency.1,5,8 The antihypertensive effect of loop diuretics is due to their ability to decrease intravascular fluid volume and eliminate salt. Compared to furosemide, the long-acting drug azosemide produces better blood pressure control, preserving the normal 10% decline in blood pressure in many individuals that occurs at night (nocturnal dipping) and is associated with better long-term outcomes.
Loop diuretics are commonly used in patients admitted with acute exacerbation of heart failure.7,8 Diuresis leads to loss of water and salt with resulting decrease in intravascular volume thus lowering ventricular filling pressure and reducing pulmonary edema.6 In addition, loop diuretics induce renal synthesis of vasodilatory prostaglandins, further enhancing their diuretic effects by increasing renal blood flow and leading to a redistribution of cortical blood flow.6 Treatment with torasemide was found to decrease readmissions related to heart failure when compared to furosemide.1
Furosemide decreases ICP by inducing systemic diuresis and decreasing cerebrospinal fluid production. This diuretic-induced decrease in ICP is not accompanied by changes in cerebral blood flow or plasma osmolarity. Furosemide can be administered as single-drug therapy (0.5 to 1.0 mg/kg IV) or as a lower dose (0.1 to 0.3 mg/kg IV) in combination with mannitol. Alterations in the blood–brain barrier do not influence the immediate or subsequent effects of furosemide on ICP. This characteristic contrasts with that of mannitol, which may produce rebound intracranial hypertension if a disrupted blood–brain barrier allows mannitol to enter the CNS. A combination of furosemide and mannitol is more effective in decreasing ICP than either drug alone, but severe dehydration and electrolyte imbalance are also more likely.
In the presence of symptomatic hypercalcemia, furosemide may be used to lower the plasma concentration of calcium by stimulating urine output.
Side Effects
Side effects of loop diuretics most often manifest as abnormalities of fluid and electrolyte balance. They can lead to hypokalemia and increase the likelihood of digitalis toxicity. As with thiazide diuretics, loop diuretics may cause hyperuricemia, but this is rarely clinically significant. Likewise, hyperglycemia, although possible, is less likely to occur than with thiazide diuretics. Acute or chronic treatment of patients with diuretics, including loop diuretics, may result in tolerance to the diuretic effect (“braking phenomenon”). Acute tolerance is presumed to reflect activation of the renin-angiotensin system to retain sodium and water in the presence of a contracted extracellular fluid volume.5 With chronic use of diuretics, there is evidence of a compensatory hypertrophy of those portions of the renal tubule (especially distal convoluted tubules) responsible for sodium retention, leading to decreased diuretic effectiveness.5 When tolerance develops in a patient treated chronically with furosemide, it may be possible to reestablish a diuretic effect with the administration of a thiazide diuretic, which blocks the hypertrophied Na+ reabsorption sites.5
Loop diuretics should only be administered to patients with a normal or increased intravascular fluid volume. Hypotension may result from administration of loop diuretics to hypovolemic patients exacerbating renal ischemic injury and concentrating nephrotoxins in the renal tubules. Accordingly, loop diuretics should be avoided in patients with acute renal insufficiency.2,9
Furosemide increases renal tissue concentrations of aminoglycosides and enhances the possible nephrotoxic effects of these antibiotics. Cephalosporin nephrotoxicity may also be increased by furosemide. In addition, loop diuretics potentiate nondepolarizing neuromuscular blockade.2 Furosemide has been associated with an allergic interstitial nephritis similar to that occasionally produced by penicillin. Cross-sensitivity may exist when a patient allergic to other sulfonamides is given furosemide. The renal clearance of lithium is decreased in the presence of diuretic-induced decreases in sodium reabsorption, and plasma concentrations of lithium may be acutely increased by the IV administration of furosemide in the perioperative period.10
Ototoxicity, either transient or permanent, is a rare, dose-dependent complication associated with the use of loop diuretics. This side effect is most likely to occur with prolonged increases in the plasma concentration of these drugs in the presence of other ototoxic drugs such as aminoglycosides or in the presence of chronic renal insufficiency.2
Thiazide Diuretics
Thiazide diuretics are most often administered for long-term treatment of essential hypertension in which the combination of diuresis, natriuresis, and vasodilation are synergistic. Included in this class of drugs are hydrochlorothiazide and thiazide-like drugs, such as chlorthalidone and indapamide. Hydrochlorothiazide is the second most frequently prescribed antihypertensive medication, and thiazides are usually administered in combination with other antihypertensives.11 Thiazide diuretics may also be used to mobilize edema fluid associated with renal, hepatic, or cardiac dysfunction. Less common uses of thiazide diuretics include management of diabetes insipidus and treatment of hypercalcemia.
Pharmacokinetics and Pharmacodynamics
Thiazide diuretics inhibit the Na+-Cl− cotransporter in the cortical portion of the ascending loop of Henle and the distal convoluted tubule, inhibiting reabsorption of 5% to 10% of the filtered sodium2 (see Fig. 22-1). Enhanced distal delivery of Na+

Full access? Get Clinical Tree
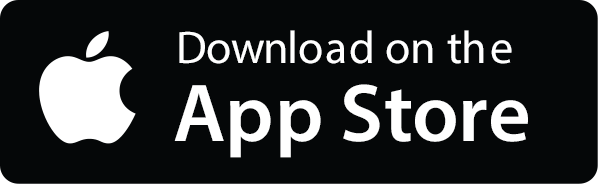
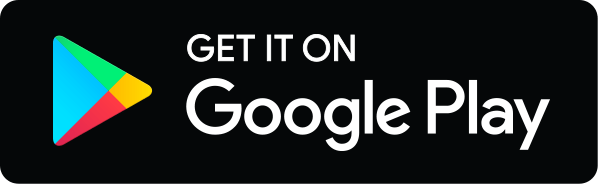