Chapter 84
Disorders of Water Homeostasis
Hyponatremia and Hypernatremia 
Principles of Body Water
Total body water (TBW) varies mainly with body weight, but it is also a function of age, sex, and fat content. It is difficult to determine the actual TBW in an ICU patient. TBW is generally assumed to be 50% to 60% of body weight in the idealized patient (50% × body weight for women and 60% × body weight for men). However, TBW may be a considerably lower percentage of body weight in the morbidly obese, and conversely as high as 70% to 80% TBW in patients with anasarca and ascites. Hence, clinical judgment and caution are essential in applying recommendations such as the algorithms in Figures 84.E1 and 84.E2 to individual ICU patients.
Water movement changes the distribution of TBW from one space to another. For example, when an osmole such as glucose is rapidly added to the ECF, water moves from the ICF to the ECF to maintain iso-osmolality between the cells and ECF, and ECF enlarges while the ICF shrinks. However, maintenance of cell volume is paramount to maintaining cell function. The cell can create additional intracellular osmoles (“idiogenic” osmoles) to prevent water movement from the cell to the ECF and thereby minimize cell shrinkage. Similarly, adding water to the ECF decreases the ECF osmolality, driving an influx of water into the cell. As an adaptive response, the cell can then expel solutes to the ECF to mitigate the gain in cell water and corresponding cell swelling.
Figure 84.E1 Schematic flow diagram to evaluate hypotonic hyponatremia in ICU patients.
Because the normal kidney has an enormous capacity to excrete water, impaired renal diluting ability is the underlying cause of most hyponatremia. ECFV, extracellular fluid volume; FENa, fractional excretion of sodium = 100 × (UNa × SCr)/(SNa × UCr), where SNa is the serum sodium concentration, UNa is the urine sodium concentration, and SCr and UCr are the serum and urine creatinine concentrations, respectively. The cutoff value for urine sodium concentration (30 mEq/L) is derived from hyponatremic patients proved to be either hypovolemic or euvolemic by their response to intravenous isotonic saline.
∗Cortisol deficiency is associated with elevated plasma vasopressin levels, accounting for the euvolemic hyponatremia characteristic of secondary adrenal insufficiency; in patients with primary adrenal insufficiency (with glucocorticoid and mineralocorticoid deficiency), however, lack of aldosterone leads to renal sodium wasting and extracellular volume contraction.
†Vomiting and nasogastric suction are associated with sodium chloride depletion and metabolic alkalosis. In steady-state metabolic alkalosis, UNa is low, reflecting hypovolemia; during episodes of vomiting (or intermittent nasogastric suction), however, acute elevations in the serum bicarbonate concentration lead to bicarbonaturia and obligate urinary cation (sodium and potassium) loss.
Figure 84.E2 Schematic flow diagram to evaluate hypernatremia in ICU patients.
Because hypernatremia is a potent stimulus to thirst, sustained hypernatremia always implies inadequate water intake. The cutoff value for renal concentrating ability (700 mmol/kg) is derived from hospitalized patients with no evidence of neurohypophyseal or renal disease. Precise cutoff values for the urine sodium concentration in hypernatremic patients with renal or extrarenal hypotonic fluid losses are not available; those provided should be considered approximations. ECFV, extracellular fluid volume; UNa, urine sodium concentration.
∗Vomiting and nasogastric suction are associated with sodium chloride depletion and metabolic alkalosis. In steady-state metabolic alkalosis, UNa is low, reflecting hypovolemia; during episodes of vomiting (or intermittent nasogastric suction), however, acute elevations in the serum bicarbonate concentration lead to bicarbonaturia and obligate urinary cation (sodium and potassium) loss.
The Physiologic Response to a Change in Total Body Water 
Workup of Hyponatremia
A pathophysiologic approach to categorize hyponatremias and identify their cause follows a series of questions (see Figure 84.E1):
1 Does the Patient Have Hypotonic Hyponatremia?
Hypotonic hyponatremia (sometimes referred to as true hyponatremia) is associated with plasma hypo-osmolality and relative TBW excess. This is distinct from isotonic hyponatremia and hypertonic hyponatremia, which are not hypo-osmolal states.
In hypertonic hyponatremia, large amounts of solutes restricted to the ECF (such as glucose and mannitol) result in water movement out of the cells. The redistribution of water to the ECF thereby reduces the sodium concentration, as commonly seen in severe hyperglycemia. For every 100-mg/dL increase in the serum glucose concentration above 400 mg/dL, one can expect a 2.4-mEq/L reduction in the serum sodium concentration. (Or alternatively, as stated in Chapter 82, every 100 mg/dL of glucose greater than 100 mg/dL decreases the serum sodium by ∼1.6 mEq/L.) Two common scenarios where this is seen are (1) uncontrolled diabetes (see Chapter 82) and (2) iatrogenic delivery of a high solute load (e.g., intravenous immune globulin [IVIG] is commonly delivered in a fluid with high sugar content). In this case, unlike hypotonic hyponatremia, cells are dehydrated. Treatment focuses on correcting the underlying cause of increased serum osmolality (e.g., insulin).
3 Why Is Renal Diluting Ability Impaired (as Evidenced by an Inappropriately Elevated Urine Osmolality)?
Hypovolemia can result from renal or extrarenal volume losses. The patient may exhibit overt signs of volume depletion such as tachycardia, orthostatic hypotension, and organ hypoperfusion. Laboratory indices such as an elevated BUN to creatinine ratio (BUN:creatinine > 20) or an elevated serum uric acid level may help identify subclinical volume loss in ICU patients. With extrarenal volume loss, the urine sodium is typically below 20 mmol/kg. Common causes for extrarenal volume loss include vomiting, diarrhea, bleeding, and “third-spacing” of fluids (e.g., pancreatitis, trauma, sepsis-induced increased vascular permeability).

Full access? Get Clinical Tree
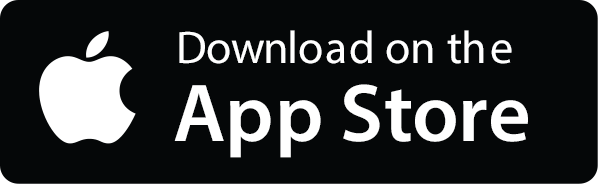
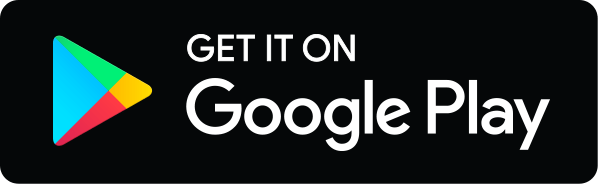