This chapter will discuss the spinal cord, peripheral nerves, neuromuscular junction (NMJ), and their associated disorders. Focus will be placed on those disorders that potentially cause respiratory muscle weakness and hemodynamic instability. These are common findings in neurological disease that necessitate intensive care.
The spinal cord is divided longitudinally into the cervical, thoracic, lumbar, and sacral cord. The cervical cord is made up of 8 segments, the thoracic cord is made up of 12 segments, the lumbar cord is made up of 5 segments, and the sacral cord is made up of 5 segments. There is also a single coccygeal segment, for a total of 31 spinal cord segments. Each segment of the cord gives off a ventral and dorsal spinal nerve root, which ultimately become the peripheral motor and sensory fibers, respectively. The cord is surrounded along its entire length by the bony vertebral column. Each vertebral body, except for C8, corresponds to its respective spinal cord segment. There is no C8 vertebral body. From C1 to C7, each root exits above its respective vertebral body. The C8 nerve root exits between the C7 and T1 vertebrae, and each root inferior to this level exits below its respective vertebrae. The spinal cord itself extends to approximately the lower margin of the first lumbar vertebral body (L1) in adults. Below this level, the spinal canal contains the lumbar, sacral, and coccygeal spinal roots that make up the cauda equina.
Spinal cord injury (SCI) is a potential cause for neurogenic shock and respiratory compromise and is therefore an important reason for admission to the intensive care unit.
Most SCIs are traumatic. In the United States in 2014, the incidence of traumatic SCI is approximately 40 cases per million people. The most common causes are motor vehicle accidents, falls, violent acts, and sports injuries.1 Acute causes of SCI lead to fractures and dislocations of the vertebrae, resulting in displaced bony fragments and disc material. The direct mechanical injury to spinal cord axons caused by these events is known as primary injury, and also includes compression and laceration. Secondary injury may continue for hours to days after the initial injury, and includes ischemia, increased intracellular calcium, extracellular glutamate, free radicals, inflammation, and apoptosis.2 In a cat model, as a result of these secondary phenomena, spinal cord edema develops within hours of injury, becomes maximal by day 3 to 6, and begins to recede by day 9.3
The severity is graded using the American Spinal Injury Association (ASIA) Impairment Scale (Fig. 17-1). Spinal cord injury is first determined to be complete or incomplete. A complete lesion is defined by the total absence of sensory and motor function in the lower sacral segments, S4 to S5. An incomplete injury will leave partial preservation of sensory and/or motor function, known as sacral sparing. Assessment for sacral sparing involves evaluation of sacral innervated muscle strength (anal sphincter tone) and regional reflexes, such as anal wink and bulbocavernosus reflex. The neurological level is then determined, which is the most caudal spinal segment with normal bilateral sensory and motor function.4 The ASIA Impairment Scale grades a complete injury as A, with grades B-E representing varying degrees of injury (grade E is a normal exam).
FIGURE 17-1
American Spinal Injury Association: International Standards for Neurological Classification of Spinal Cord Injury. (Scale updated November 2015. © 2011 American Spinal Injury Association. Reprinted with permission. http://asia-spinalinjury.org/wp-content/uploads/2016/02/International_Stds_Diagram_Worksheet.pdf.)

Recovery after spinal cord injury mostly occurs during the first 6 to 9 months and relates to the severity of injury. A complete injury may convert to an incomplete injury in about 10% to 20% of patients, with the conversion from ASIA grade A to grade D occurring in only a minority of those patients.4 Very few patients with complete injury regain the ability to ambulate, whereas 20% to 50% of those with an initial injury severity of ASIA grade B may ambulate by 1 year.4
Methylprednisolone is the only treatment in the acute setting of traumatic, nonpenetrating SCI that has been suggested by clinical trials to improve outcomes. The National Acute Spinal Cord Injury Study (NASCIS) II enrolled patients between 1985 and 1988 and compared methylprednisolone, naloxone, and placebo in acute traumatic SCI. At 1 year, no statistically significant differences in motor or sensory function were found between groups. However, in a subgroup analysis, those treated with methylprednisolone within 8 hours of injury demonstrated significantly improved motor recovery. Administration of methylprednisolone in acute SCI became standard of care in the years after NASCIS II.2,5,6 This data has been heavily scrutinized in the past, with most critiques focusing on the post-hoc subgroup analysis, clinical significance of the reported motor improvement, and poor reproducibility. Others cite the risks associated with high-dose steroids, particularly wound infections, systemic infections, and gastrointestinal hemorrhage.2 In 2013, the American Association of Neurological Surgeons and Congress of Neurological Surgeons stated that, based on the available evidence, the use of glucocorticoids in acute spinal cord injury is not recommended.7 Some groups maintain the use of steroids as a treatment option (rather than a treatment standard) and to be considered in the clinical setting, arguing that the catastrophic results of SCI may lead to patient preference to receive treatment.7
Although trauma is the leading cause of acute SCI, a myriad of conditions can present similarly. Table 17-1 provides a differential diagnosis for nontraumatic acute SCI.8 Figure 17-2 illustrates the vascular supply of the spinal cord and tracts. Figure 17-3 illustrates spinal cord syndromes. Table 17-3 further elaborates on spinal cord syndromes. Figure 17-4 illustrates the sympathetic and parasympathetic nervous system.
Steps in Classifying Spinal Cord Injury | Grades and Definitions |
---|---|
1. Assess sensory level on both sides for pin prick and light touch. | Sensory Grading:
|
2. Assess motor level on both sides. | Motor Grading:
|
3. Assess Neurologic Level of Injury (NLI) |
|
4. Complete or Incomplete |
|
5. Determine ASIA Impairment Scale |
|
FIGURE 17-2
Spinal Cord Vascular Supply Distribution and Tracts. The anterior sulcus artery and the circumflex artery arise from the anterior spinal artery. The afferent or ascending tracts are: The Dorsal Column Medial Meniscus (1A = Gracile Fasciculus and 1B = Cuneatus Fasciculus); 2A = Posterior Spinocerebellar tract; 2B = Anterior Spinocerebellar Tract; 3 = Anterior and lateral spinothalamic tract; and 4 = spino-olivary fibers. The motor or descending tracts are: 5A = lateral corticospinal tract; 5B = anterior corticospinal tract; 6 = rubrospinal tract; 7A and 7B = reticulospinal tracts; 8 = olivospinal tract; and 9 = vestibulospinal tract.

FIGURE 17-3
Spinal cord syndromes.9 (Reprinted with permission from Crout TM, Parks LP, Majithia V. Neuromyelitis optica (Devic’s syndrome): an appraisal. Curr Rheumatol Rep. 2016;18(8):54.)

FIGURE 17-4
Sympathetic (left) and parasympathetic (right) nervous system. Sympathetic preganglionic neurons are located within the lateral horns of the T1–L2 spinal segments, and are under tonic excitatory input from descending pathways originating in the medulla. Preganglionic parasympathetic neurons are located in the brainstem and the S2–S4 spinal segments.20 (Reprinted with permission from Neary D, Crossman AR. Neuroanatomy: An Illustrated Colour Text. 5th ed. Edinburgh: Churchill Livingstone; 2015. Internet resource.)

The symptomatology associated with each process listed in Table 17-1 depends on the spinal cord levels involved and the tracts of the spinal cord involved. For example, neuromyelitis optica (NMO) is an inflammatory disorder of the central nervous system characterized by severe demyelination and axonal damage that predominantly targets the optic nerves and spinal cord.9 The brain parenchyma tends to be relatively spared in NMO. A common presentation of NMO is with transverse myelitis (TM), an inflammatory spinal cord dysfunction that may involve both gray and white matter. The transverse myelitis of NMO is typically longitudinally extensive (extending across 3 or more contiguous vertebral segments). If the lesion extends from the brainstem or high spinal cord downward, patients may present with quadriplegia and bladder dysfunction, and potentially with respiratory distress because of involvement of the muscles of respiration innervated by the cervical roots.
Acute spinal cord infarction, on the other hand, most often occurs because of occlusion of the anterior spinal artery (ASA).8 The ASA is fed along its length by radicular arteries, the largest of which is the artery of Adamkiewicz arising from the 9th to 12th intercostal arteries. The artery of Adamkiewicz is an important arterial supply of the ASA’s distribution over the lower third of the spinal cord, including the conus medullaris, and is vulnerable to occlusion by aortic aneurysm and aortic repair procedures. Rostral to the artery of Adamkiewicz, there is relatively low collateral flow, making the midthoracic region of the cord particularly vulnerable to systemic hypoperfusion.8 Other causes of spinal cord infarctions include cardiogenic embolism, aortic dissection, aortic thrombosis, vertebral artery dissection, spinal vascular malformations, or cocaine abuse.10
Because the ASA supplies the corticospinal and spinothalamic tracts, while the posterior spinal artery supplies the posterior columns, most patients with a spinal cord infarct will present with flaccidity and loss of reflexes below the level of infarction, and loss or reduction of sensation to pain and temperature, with relative sparing of vibratory and proprioceptive sense. Acute back pain is commonly associated and occurs near the level of infarction.8
Certain pathological processes preferentially involve the anterior horn cells of the spinal cord. West Nile virus, for example, can present in a similar way to polio virus, leading to progressive asymmetric weakness over as short a time as 48 hours. This typically occurs in the setting of meningitis or encephalitis. Facial weakness and bulbar symptoms, such as dysphagia or dysarthria, increase the risk for impending respiratory failure. In 1 study, respiratory failure requiring intubation was seen in 38% of patients with West Nile virus–associated paralysis.11 Amyotrophic lateral sclerosis (ALS) is a neurodegenerative process that also involves the anterior horn cells, although progression to respiratory failure tends to occur over the course of months to years.
Impairment of ventilatory musculature may lead to acute respiratory compromise and the need for mechanical ventilation. In a study of respiratory failure among patients with cervical SCI, 90% of cases that required mechanical ventilation occurred in the first 3 days after injury.12
The primary muscle of inspiration is the diaphragm, which is innervated by the phrenic nerve. The phrenic nerve is derived from the C3 to C5 nerve roots. Accessory muscles of inspiration include the scalenes, external intercostals, clavicular portion of the pectoralis major, and sternocleidomastoids. Muscles of expiration include the abdominal wall muscles and the internal intercostals. Although expiration is normally passive, these muscles are required for forced exhalation, such as is needed to effectively cough.
Injury to the spinal cord above the level of C3 will produce near-total paralysis of ventilation. Patients with injury to the high cervical cord will therefore require immediate mechanical ventilation. Injury from C3 to C5 will produce variable levels of weakness of both the diaphragm and the accessory muscles, and typically also will require intubation.8,13 In patients with injury below C5, the diaphragm is spared and expiration will occur via passive recoil of the chest wall. However, these patients will still suffer from a weak cough mechanism and are at risk for respiratory fatigue in the setting other systemic pulmonary conditions such as pneumonia.14 Table 17-2 lists the nerve and associated muscle of inspiration and expiration. Figure 17-3 illustrates the spinal cord syndromes, and Table 17-3 lists the spinal cord syndromes, associated tracts, causes, and symptoms.8,13-15
Innervation | Muscles of Inspiration |
---|---|
Phrenic nerve (C3–C5) | Diaphragm |
C4–C8 | Scalenes |
Thoracic nerve roots | External intercostals |
Spinal accessory nerve | Sternocleidomastoid |
Lateral pectoral nerve (C5–C7) | Pectoralis major, clavicular head |
Muscles of Expiration | |
Lower thoracic, lumbar nerve roots | Abdominal wall muscles |
Thoracic nerve roots | Internal intercostals |
Spinal Cord Syndrome | Involved Tracts | Causes | Symptoms |
---|---|---|---|
Posterior cord syndrome | Bilateral dorsal columns, corticospinal tracts, descending central autonomic tracts | Multiple sclerosis, subacute combined degeneration, vascular malformations, atlantoaxial subluxation | Dorsal columns: loss of vibration and proprioception, gait ataxia, paresthesias Corticospinal: weakness Autonomic: urinary incontinence |
Anterior cord syndrome | Bilateral corticospinal tracts, spinothalamic tracts, descending autonomic tracts | Anterior spinal artery stroke, intervertebral disc herniation | Corticospinal: weakness Spinothalamic: loss of pain and temperature Autonomic: urinary incontinence |
Central cord syndrome | Decussating spinothalamic fibers at level of lesion | Syringomyelia, hyperextension injury with underlying cervical spondylosis, intramedullary tumor | Loss of pain and temperature along respective dermatomes Sensation normal above and below lesion |
Brown-Sequard syndrome | Unilateral dorsal column, spinothalamic tract, corticospinal tract | Stab wounds, bullet wounds, demyelination | Dorsal columns: ipsilateral loss of vibration and proprioception Spinothalamic: contralateral loss of pain and temperature Corticospinal: ipsilateral weakness |
Conus medullaris syndrome | Terminus of spinal cord | Lesions at L1–L2; intervertebral disc herniation, fracture, tumor | Flaccid paralysis of bladder and rectum, impotence, saddle anesthesia, bilateral leg weakness |
Cauda equina syndrome | Lumbosacral nerve roots below the conus medullaris: L2–L5, S1–S5 | Disc herniation, lumbar spondylosis, epidural abscess, acute or chronic inflammatory demyelinating polyradiculoneuropathy, carcinomatous meningitis, viral infection, Lyme disease, tuberculosis | Low back pain with radiation to legs, bilateral leg weakness and sensory loss to all modalities, bladder and sphincter paralysis |
The term spinal shock refers to sudden and transient suppression of all neural functions below the level of a spinal cord lesion. In this state, a flaccid paralysis of skeletal muscle occurs, and all tendon, cutaneous, and autonomic reflexes are abolished or greatly reduced.16 Neurogenic shock refers to a state of hemodynamic instability following spinal cord injuries, or in some case, severe head trauma.
The sympathetic preganglionic neurons are located within the lateral horns of the spinal gray matter of the thoracic and upper lumbar spinal segments (T1–L2). These neurons are under tonic excitatory input from descending pathways originating in the medulla. High spinal cord lesions, especially at the level of the cervical cord or high thoracic cord, may disrupt these sympathoexcitatory fibers leading to alterations in sympathetic and parasympathetic control of cardiovascular dynamics.17
Initially after injury, sympathetic hypoactivity occurs. Over time, there is also alteration in morphology of the sympathetic preganglionic neurons, plastic changes within spinal circuits (including sprouting and the formation of inappropriate synaptic connections), and the development of peripheral α-adrenergic receptor hyperresponsiveness.18
During the period of sympathetic hypoactivity, there is uninhibited parasympathetic input via an intact vagus nerve. Profound hypotension and persistent bradycardia may occur, which are common features of neurogenic shock. The mainstay of treatment in neurogenic shock is pressor support as needed, as well as maintenance of appropriate blood volume. It is recommended that mean arterial pressure be maintained at a level of at least 85 to 90 mmHg for the first week after acute SCI in order to promote adequate spinal perfusion19 (the extent and duration of the resulting hypotension is associated with the severity of spinal cord injury, with greater and more complete injuries requiring longer duration of pressor therapy.17
Autonomic dysreflexia (AD) refers to the loss of coordinated autonomic response to various cardiovascular demands. Whereas loss of supraspinal sympathoexcitatory pathways can lead to neurogenic shock and chronic low resting blood pressure, AD is a potentially life-threatening condition in which sudden, extreme rises in blood pressure result from uncontrolled surges in sympathetic tone in response to noxious stimuli.20 Autonomic dysreflexia is typically seen in patients with spinal cord lesions above the level of T6. With a noxious impulse below the level of the lesion, an afferent signal stimulates the sympathetic neurons in the spinal cord in the intermediolateral gray matter. Normal descending inhibitory pathways are unable to pass the level of injury, resulting in widespread vasoconstriction below the level of injury, particularly in the splanchnic vasculature. Peripheral resistance increases, and blood volume that is normally congested is shunted into the systemic circulation. This combination of increased vascular tone and increased volume leads to sudden, potentially dangerous rises in blood pressure, to upwards of 300 mmHg systolic and 200 mmHg diastolic.20,21 Potentially severe consequences of this rise in blood pressure include intracerebral hemorrhage, retinal detachment, or seizures.
In response to sympathetic drive, intact baroreceptors then activate the parasympathetic response. While there is vasoconstriction below the lesion, parasympathetic response activity results in peripheral vasodilation above the level of the lesion, which can cause severe headache, sweating, facial flushing, and nasal congestion.20 This may additionally be accompanied by bradycardia. Arrhythmias such as atrial fibrillation, premature ventricular contractions, and atrioventricular conduction abnormalities have been reported.20,21
Although it has been reported, AD is typically not seen in lesions below the level of T6, because intact splanchnic innervation allows for preserved vasodilation of the splanchnic vasculature. Autonomic dysreflexia is most commonly seen in the chronic stages after SCI; however, it has been proposed that early cases of AD are under-recognized. Krossioukov and colleagues reported cases of autonomic dysreflexia occurring as early as 4 days after SCI.18

Full access? Get Clinical Tree
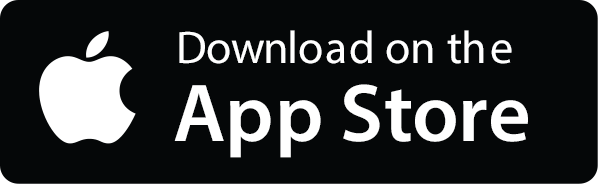
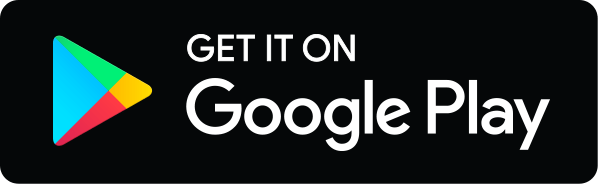