Disorders of Temperature Control Part II: Hyperthermia
M. Kathryn Steiner
Frederick J. Curley
Richard S. Irwin
This chapter reviews the pathobiology, pathophysiology, diagnosis, differential diagnosis, and treatment of four major hyperthermic syndromes—heat stroke, malignant hyperthermia, neuroleptic malignant syndrome, and drug-induced hyperthermia. Establishing the correct diagnosis and promptly instituting specific therapy are essential to management as mortality rises with any delay in treatment.
Heat Stroke
Heat stroke is a syndrome of acute thermoregulatory failure in warm environments characterized by central nervous system (CNS) depression, core temperatures usually above 40°C, and typical biochemical and physiologic abnormalities. Most cases of heat stroke occur in youths exercising in the sun, especially military recruits and athletes, or in elderly or ill patients during severe heat waves. Mortality in some series is as high as 70% [1]. During a warm summer in the United States, approximately 4,000 deaths may occur as a direct result of heat stroke [2,3,4].
Causes and Pathogenesis
Heat stroke may be subclassified by its two distinct clinical presentations: exertional and nonexertional (classic, heat stroke). Exertional heat stroke is typically seen in younger individuals exercising at higher than normal ambient temperatures. The thermoregulatory mechanisms are intact, but overwhelmed by the thermal challenge of the environment and the great increase in endogenous heat production. Nonexertional heat stroke occurs in the elderly or sick individuals during a heat wave. Patients frequently have some impairment of thermoregulatory control, and temperatures rise easily with increased thermal challenge.
The causes of heat stroke fall into two categories (Table 66.1): increased heat production and impaired heat loss.
Increased Heat Production
Endogenous heat production during exertion ranges from 300 to 900 kcal per hour. Even in conditions favoring the maximal evaporation of sweat, only 500 to 600 kcal per hour of heat may be lost. Endogenous heat production may also be increased by fever, thyrotoxicosis, or the hyperactivity associated with amphetamine and hallucinogen use. In these conditions of increased thermogenesis, especially during maximal exercise, a healthy individual with intact regulatory mechanisms may develop hyperthermia.
Impaired Heat Loss
Schizophrenic, comatose, senile, or mentally deficient patients are at increased risk of heat stroke when ambient temperatures are high, owing to impaired voluntary control [5,6]. These patients may fail to perceive a temperature rise and take appropriate action. Impermeable clothing in hot environments has a great reduction in evaporative heat loss and individuals may suffer heat stroke [7,8].
Acclimatization increases heat tolerance by increasing cardiac output; decreasing peak heart rate; and increasing stroke volume. This lowers the threshold necessary to induce sweating; increases the volume of sweating; and, via an increase in aldosterone, expands extracellular volume and minimizes sweat sodium loss [9,10]. However, unacclimatized individuals who do not mount an adaptive response are at increased risk of suffering exertional heat stroke [11].
Dehydration and impaired cardiovascular performance increases the risk of heat stroke due to a decrease in skin or muscle blood flow, thus decreasing the movement of heat from the core to the environment [10,12]. Hypokalemia increases the risk of heat stroke by decreasing muscle blood flow, impairing cardiovascular performance, and possibly decreasing sweat gland function [9,10]. Adequate fluid intake and maintenance of a normal vascular volume prevents heat stroke. Heat load places a stress on the cardiovascular system and produces hyperthermia in patients with cardiovascular dysfunction. In one report, 75% of patients with compensated cardiac failure developed overt heart failure and temperatures up to 38.0°C after as little as 4 hours’ exposure to temperatures of 32.2°C. Respiratory rate, blood pressure, and central venous pressure (CVP) also tended to rise [13].
Many drugs are known to predispose to heat stroke. Anticholinergic drugs such as phenothiazines, butyrophenones, thiothixenes, and anti-Parkinson’s medications reduce sweat activity [14]). Barbiturate overdose may produce sweat gland necrosis [10]. Diuretics promote dehydration and hypokalemia. Beta-blockers may increase the risk of heat stroke because of cardiodepression. Alcohol consumption may increase the risk of heat stroke 15-fold because of dehydration secondary to antidiuretic hormone inhibition and inappropriate vasodilation [6].
Skin disorders that impair sweat gland function, such as cystic fibrosis and chronic idiopathic anhydrosis, predispose to heat stroke [15]. Hypothalamic lesions impair thermoregulation. During the early stages of heat stroke, the hypothalamus regulates autonomic responses to limit hyperthermia to occur. In the later stages, after thermal toxicity has occurred, hypothalamic regulation is impaired [16]. Anhydrosis has been reported in up to 100% of heat stroke victims in some series [17]. The hypothalamic set point may be elevated. The exact cause of hypohidrosis remains unclear and may reflect hypothalamic dysfunction or only the secondary effects of dehydration and cardiovascular collapse. Electron microscopic studies of eccrine sweat glands in a patient with fatal exertional heat stroke show changes suggestive of sweat gland fatigue [18]. Heat stroke can, however, occur in individuals who perspire profusely, indicating that sweat gland malfunction is not the only factor contributing to the pathogenesis of the syndrome.
Table 66.1 Causes of Heat Stroke | |
---|---|
|
The increased risk of heat stroke in the elderly is predominantly due to a decreased ability to sweat and a compromised cardiovascular response to heat exposure when compared with younger individuals [8,19]. In one report, 84% of elderly patients showed no evidence of sweating at the time heat stroke was diagnosed [20]. Elderly patients are more likely to have deficient voluntary control, poor acclimatization, and they take drugs that adversely affect thermoregulation.
Pathophysiology
The primary injury in heat stroke is due to the direct cellular toxicity of temperatures above 42°C, the critical thermal maximum [21]. Cell function deteriorates owing to cessation of mitochondrial activity, alterations in chemical bonds involved in enzymatic reactions, and cell membrane instability. This toxic effect may account for the widespread organ damage seen in all three of the major hyperthermic syndromes [22].
Heat stress activates numerous cytokines that modulate the body’s response to increased temperature [23]. In most cases, the inflammatory response in heat stroke parallels that seen in heat stress from exertion. Tumor necrosis factor α, interleukin- (IL)1β, IL-2, IL-6, IL-8, IL-10, IL-12, and interferon gamma are typically increased in heat stroke. IL-6 is activated in the muscles and modulates inflammatory response by controlling cytokine levels and hepatic production of acute phase proteins. Endotoxemia from bacterial translocation of an ischemic gut further exacerbates the inflammatory response. Endothelial injury activates the coagulation cascade, promoting a prothrombotic state. Heat shock proteins are transcribed in response to heat stress and act in the brain to induce tolerance to heat stress [24].
Dehydration, metabolic acidosis, and local hypoxia alter the pathophysiologic consequences and clinical presentation of each of the hyperthermic syndromes. For example, classic heat stroke may occur with relatively little metabolic acidosis because no exertion was involved in its onset; however, it may be associated with more pronounced dehydration due to the gradual rise in temperature and prolonged sweating. Exertional heat stroke, alternatively, may be accompanied by a severe metabolic acidosis and hypoxia due to muscular exercise. It is typically associated with a more normal volume status because the onset of temperature elevation is abrupt.
Muscle Effects
Muscle degeneration and necrosis occur as a direct result of high temperatures. Muscle damage is more severe in exertional heat stroke owing to the local increases in heat, hypoxia, and metabolic acidosis associated with exertion. Significant muscle enzyme elevation and severe rhabdomyolysis are extremely common in exertional heat stroke [12,25,26] but rare in classic heat stroke [27].
Cardiac Effects
Cardiac output is increased [28] due to increased demands and low peripheral vascular resistance secondary to vasodilation and dehydration. Dehydration frequently results from sweat rates that may easily reach 1.5 to 2.0 L per hour during episodes of heat stroke [29]. CVP is initially elevated [30].
Hypotension occurs commonly as a result of high-output failure or temperature-induced myocardial hemorrhage and necrosis with subsequent cardiac depression and failure [9,12,31]. Tachyarrhythmias are frequent. Postmortem specimens show focal myocytolysis, myocyte necrosis, and hemorrhage in subepicardial, intramuscular, subendocardial, or intravalvular tissues [32].
Central Nervous System Effects
Direct thermal toxicity to brain and spinal cord rapidly produces cell death, cerebral edema, and local hemorrhage. These may lead to profound stupor or coma, almost universal features of all the hyperthermic syndromes. Seizures secondary to edema and hemorrhage are not uncommon. Because Purkinje cells of the cerebellum are particularly sensitive to the toxic effects of high temperatures, ataxia, dysmetria, and dysarthria may be seen acutely and in survivors of hyperthermia [10,33]. Progressive cerebellar atrophy has been documented by computed tomography and magnetic resonance imaging [34]. Lumbar punctures in classic and exertional heat stroke may reveal increased protein levels, xanthochromia, and a slight lymphocytic pleocytosis [12,20]. Survivors of severe heat stroke may show premature cataract formation, considered to be secondary to dehydration [35]. Up to 33% of survivors of heat stroke have at least moderate neurologic impairment after discharge from the hospital [36].
Renal Effects
Renal damage occurs in nearly all hyperthermic patients; it is potentiated by dehydration, cardiovascular collapse, and rhabdomyolysis. In classic heat stroke, acute renal failure occurs on average in 5% of patients as a result of dehydration [9]. In exertional heat stroke, acute renal failure occurs in up to 35% of cases [9,31]. Dehydration, pigment load, hypoperfusion, and urate nephropathy are thought to contribute to a clinical picture of acute tubular necrosis [31]. Other features include low serum osmolarity, moderate proteinuria, active sediment, and
characteristic machine-oil appearance of the urine. In one series, the incidence of acute tubular necrosis increased with survival time [32]. Hypocalcemia and creatine phosphokinase values above 10,000 U per L increase the risk of acute renal failure [37]. Respiratory alkalosis is common in mild hyperthermia with metabolic acidosis predominating at temperatures greater than 41°C [24].
characteristic machine-oil appearance of the urine. In one series, the incidence of acute tubular necrosis increased with survival time [32]. Hypocalcemia and creatine phosphokinase values above 10,000 U per L increase the risk of acute renal failure [37]. Respiratory alkalosis is common in mild hyperthermia with metabolic acidosis predominating at temperatures greater than 41°C [24].
Gastrointestinal Tract Effects
The combination of direct thermotoxicity and relative hypoperfusion of the intestines during hyperthermia leads to ischemic intestinal ulcerations that may result in frank bleeding [9]. Hepatic necrosis and cholestasis occurs 2 to 3 days after hyperthermic insult, and 5% to 10% of cases result in death [10].
Hematologic Effects
White blood cell counts are elevated owing to catecholamine release and hemoconcentration. Anemia and a bleeding diathesis [29] are present due to (a) direct inactivation of platelets and bleeding factors by the heat, (b) a decrease in coagulation factor synthesis owing to liver failure, (c) a decrease in platelet and megakaryocyte counts, (d) platelet aggregation [38], and (e) disseminated intravascular coagulation (DIC). Megakaryocyte counts are reduced in up to 50% of specimens, and surviving megakaryocytes are morphologically abnormal [32]. DIC is present in most cases of fatal hyperthermia [32,39], most frequently appearing on the 2nd or 3rd day after hyperthermic insult. It is thought to be due to activation of the clotting cascade by vascular endothelial damage and generalized cell necrosis [40]. In cases of DIC, cardiac, CNS, pulmonary, gastrointestinal (GI) tract, and renal complications are exacerbated. An increase in blood viscosity of up to 24% has been postulated to facilitate thromboses [41].
Endocrine Effects
Hypoglycemia may occur in severe exertional heat stroke due to metabolic exhaustion [26]. In milder heat stroke, hyperglycemia and elevations of serum cortisol have been reported [42]. Although in autopsies the adrenal glands frequently show pericortical hemorrhages, survivors show little evidence of adrenal dysfunction [22,31]. Growth hormone and aldosterone levels actually increase abruptly during severe, acute heat exposure and are thought to act to preserve volume.
Electrolyte Effects
Hyperthermia produces frequent imbalances in potassium, sodium, phosphate, and calcium levels [29,43]. In heat stroke, sweating involves the active excretion of potassium from the body, producing normal to low serum potassium levels and slightly decreased total body potassium concentrations. In cases of exertional heat stroke with severe cell injury, potassium levels may be extremely elevated owing to cell lysis. Although mild hypophosphatemia occurs frequently as a result of intracellular trapping and possible parathyroid hormone resistance, phosphate levels may decrease to less than 1 mg per 100 mL in cases of hyperthermia with severe rhabdomyolysis [43]. Calcium values may fall 2 to 3 days after cellular injury owing to intracellular precipitation. In patients with severe tissue injury rebound, hypercalcemia may occur 2 to 3 weeks after hyperthermia as a result of parathyroid hormone activation [43].
Pulmonary Effects
Direct thermal injury to the pulmonary vascular endothelium may lead to cor pulmonale or acute respiratory distress syndrome. This and the tendency toward myocardial dysfunction make pulmonary edema common. Increased oxygen demands and acidosis frequently produce a respiratory alkalosis. Metabolic acidosis is, however, the most common acid–base disorder [44].
Diagnosis
Heat stroke is usually readily suggested by history and physical examination, and the diagnosis confirmed by recording a rectal temperature above 40°C. The temperature of any individual found comatose during a heat wave should be taken. Any laborer or athlete displaying incoordination followed by stupor and collapse while exercising in the heat should be assumed to have heat stroke until proven otherwise. Because more than 6 million workers in the United States experience occupational heat stress [8], a history of the exact events precipitating collapse may be helpful.
Heat stroke should be expected in any patient exercising in hot weather or in susceptible individuals during heat waves (see Table 66.1). Coma or profound stupor is nearly always present, but the other traditional criteria of anhidrosis and core temperature above 41°C may be absent. Although anhidrosis occurs in 84% of elderly patients with classic heat stroke [20], profuse sweating is typically present in exertional heat stroke [10]. Thus, the presence of anhidrosis is helpful, but its absence is not. Likewise, by the time the patient receives medical care, the temperature may have fallen significantly owing to cessation of exertion, removal from a hot environment, or cooling measures undertaken during transport. Most patients do have a temperature above 40°C, however. Because the level of serum creatine kinase is almost always elevated, the authors believe diagnostic criteria for heat stroke should include (a) a core temperature above 40°C, (b) severely depressed mental status or coma, (c) elevated serum creatine kinase level, and (d) compatible historical setting.
Classic heat stroke occurs more frequently when ambient peak temperatures exceed 32°C and minimum temperatures do not fall below 27°C. The risk is greater in urban areas, where minimum temperatures may exceed that in surrounding communities by more than 5°C [3]. Death rates during these heat waves may exceed twice the normal rates, and heat stroke deaths usually lag behind peak temperatures by approximately 24 hours. More than 80% of heat stroke victims are older than 65 years [20,40]. Other major high-risk groups are schizophrenics, patients with parkinsonism, alcoholics, and paraplegics or quadriplegics [45,47].
Exertional heat stroke may be seen when ambient temperatures are in the 25°C range, but more frequently it occurs at higher temperatures. Exertional heat stroke is frequently seen in military recruits during basic training [11,26], amateur football players [10,48], and marathon runners [49,50,51,52]. Miners and others who labor in hot local environments are also at high risk [31]. Heat stroke remains the second leading cause of death in athletes, second only to injuries of the head and spinal cord [9].
Differential Diagnosis
Several publications outline an approach to fever in the critically ill patient [53,54]. Table 66.2 lists the common causes of hyperthermia. Hyperthermia and coma may occur with hypothalamic injury, severe infection, or endocrinopathy [55]. Hypothalamic tumors or hemorrhage may produce hyperthermia by elevating the regulated temperature set point and may be distinguished from heat stroke by the constancy of the temperature and associated defects, such as diabetes insipidus and
anhidrosis, which may be unilateral [56]. Meningitis and encephalitis usually lack the characteristic enzyme elevations and may be distinguished by lumbar puncture.
anhidrosis, which may be unilateral [56]. Meningitis and encephalitis usually lack the characteristic enzyme elevations and may be distinguished by lumbar puncture.
Table 66.2 Differential Diagnosis of Hyperthermia | |
---|---|
|
Treatment
Primary Therapy of Hyperthermia
Primary therapy includes cooling and decreasing thermogenesis. Some cooling may be achieved in the field by moving the victim to a shaded, cooler area; removing the clothes; constantly wetting the skin; and fanning or transport in an open vehicle to create a breeze. Once the victim reaches hospital, cooling and subsequent supportive care are best provided in an intensive care setting.
Cooling by evaporative or direct external methods has proved effective. Evaporative cooling methods involve placing a nude patient in a cool room, wetting the skin with water, and encouraging evaporation by using fans. In one specially designed evaporative cooling unit, patients were sprayed with 15°C water and their skin fanned at 30 times per minute with air heated to 45°C to 48°C. Temperature reduction was rapid and mortality was 11% [57]. There was no mortality in 25 patients with nonexertional heat stroke treated with cooling by covering with a cool, wet, 20°C sheet and fanning with two 35-cm electric fans. Fanning was adjusted to maintain skin temperature at 30°C to 32°C; skin temperature fell 1°C every 11 minutes [58]. In 14 patients with nonexertional heat stroke, there was one death when evaporative/convective cooling was employed. The median time to return to temperature less than 39.4°C was 60 minutes.
Direct external cooling involves immersing the patient in ice water or packing the patient in ice. Ice water immersion with massage has been effective with little complication [59]. Colder water cools more rapidly (up to 0.35°C per minute), with one study demonstrating that 2°C water cooled volunteers twice as rapidly than 8°C water [60]. Because cold skin temperatures produce vasoconstriction, however, constant massage may be necessary to allow circulation to carry heat from the core. Direct external cooling is highly effective but makes patient monitoring and management extremely inconvenient. Therefore, some authors advocate evaporative cooling as a safer cooling method in patients at high risk of cardiovascular collapse [52]. As comparative studies of cooling techniques use different water temperatures for immersion and different evaporative cooling protocols, there is no consensus on which technique is superior. In most cases, treatment will be determined by what resources are immediately available. The Israeli Defense Forces protocol involves moving the collapsed patient to the shade, removing clothing, splashing the skin with water while fanning, and transport to hospital in an open vehicle. These measures yielded a cooling rate of 0.11°C per minute [61]. The US Marine Corps protocol calls for covering the patient with sheets covered with ice and then fanning the patient. This has had no mortalities in 200 cases and has reduced temperatures to below 39°C in 10 to 40 minutes [62].
In rare instances in which evaporative and direct external cooling methods fail to reduce the temperature, peritoneal lavage with iced saline cooled to 20°C or 9°C, gastric lavage, or hemodialysis or cardiopulmonary bypass with external cooling of the blood may be necessary to reduce the temperature. Temperature should be continuously monitored and cooling stopped as it approaches 39°C. Although chlorpromazine in an intravenous (IV) dose of 10 to 25 mg has been advocated to prevent shivering during cooling, it is usually unnecessary. Cooling blankets, although commonly used, are extremely ineffective and are not recommended [63]. Dantrolene has been shown to be ineffective in reducing hospitalization rate in heat stroke [64], and although it may improve cooling rate, it did not alter mortality [65].
Therapy for Complications of Hyperthermia
Arrhythmias, metabolic acidosis, and cardiogenic failure complicate the early management of hyperthermic crises. Supraventricular tachyarrhythmias usually require no treatment because they respond to restoration of normal temperature and metabolism. Digitalis should be avoided owing to the likelihood of hyperkalemia.
Hypotension should be treated initially with normal saline and, if necessary, isoproterenol. Dopaminergic and α-agonists should be avoided because they tend to produce peripheral vasoconstriction. Volume expansion with dextran is contraindicated owing to its anticoagulating effect. Pulmonary artery and arterial catheter monitoring may be helpful in the management of hypotension because patients frequently have low peripheral resistance, dehydration, and impaired cardiac function and are at a high risk for congestive heart and renal failure. As 64% of patients may have a normal central venous pressure before resuscitation, volume expansion in most cases should be guided by intravascular pressure monitoring where available [66]. Seizures, quite common in heat stroke, usually respond to diazepam.
Blood gas status should be determined early in treatment. Blood gases drawn at temperatures above 39°C should be corrected for temperature, although to our knowledge no studies have demonstrated that this is clinically necessary. The solubility of oxygen and carbon dioxide increases as blood drawn from the patient is cooled to 37°C for analysis. This lowers the carbon dioxide and oxygen tensions and elevates the pH when compared with values present in the patient. Therefore, the patient is more acidotic and less hypoxic than the uncorrected values indicate. Normal values of intracellular pH and changes on the body’s buffering system in hyperthermia have been poorly described. Because normal values for blood gases in hyperthermic patients are unavailable, by convention the blood gas values are corrected for temperature, using any reliable nomogram, and clinical decisions are made as if the patient were euthermic [67,68,69]. The following approximate corrections have been used: for each 1°C that the patient’s temperature is above 37°C, the oxygen tension is increased by 7.2%, carbon dioxide (CO2) tension increased by 4.4%, and pH is lowered by 0.015 units. More research is needed before definite
conclusions can be made. Nevertheless, 100% oxygen should be delivered until adequate oxygenation is ensured. Bicarbonate should be administered, guided by frequently obtained arterial blood gas values. The base deficit is frequently large, and up to 30 g of bicarbonate has been required for correction. Comatose patients should have prophylactic intubation to protect their airways from aspiration.
conclusions can be made. Nevertheless, 100% oxygen should be delivered until adequate oxygenation is ensured. Bicarbonate should be administered, guided by frequently obtained arterial blood gas values. The base deficit is frequently large, and up to 30 g of bicarbonate has been required for correction. Comatose patients should have prophylactic intubation to protect their airways from aspiration.
Urine output should be closely monitored with an indwelling bladder catheter. Patients should be routinely given 1 to 2 mg per kg of mannitol over 15 to 20 minutes to promote continued urine flow and possibly decrease cerebral edema. Continuous urine output should then be maintained with intermittent doses of furosemide. In all cases of hyperthermia, serum potassium levels should be closely followed. In cases of oliguria or potential renal failure, polystyrene sulfonate should be given early because hyperkalemia frequently increases.
Moderate-to-severe liver failure is common, may prolong illness, and, in combination with renal failure, may make administration of several drugs difficult or impossible. Although no clinical data are yet available, histamine receptor type 2 (H2)–blocking drugs or proton pump inhibitors given prophylactically may decrease the incidence of GI tract bleeding.
The occurrence of DIC greatly affects mortality: Most patients who die of heat stroke have evidence of DIC [70]. Coagulation parameters such as prothrombin time, partial thromboplastin time, platelet count, and fibrinogen should be carefully followed. Should DIC occur, traditional recommendations for treatment should be followed (see Chapter 108).
Prognosis
Morbidity and mortality are directly related to the peak temperature reached and time spent at elevated temperatures. A delay in treatment of only 2 hours may result in the likelihood of death up to 70% [11,52]. When heat stroke is swiftly recognized and aggressively treated, mortality should be minimal. For example, in one series of 15 patients with exertional heat stroke, all were successfully treated with no mortality and little morbidity [11]. Another study predicts mortality of only 5% when heat stroke patients are managed properly [10]. A recent review of 34 elderly patients with classic heat stroke revealed 18% mortality. Seventy-three percent recovered without sequelae, and 9% had some residual neurologic deficit.
Although patients with temperatures as high as 46.5°C have survived without sequelae [71], mortality is increased with premorbid debility and higher maximal temperatures [44]. When ventricular fibrillation, DIC, coma lasting more than 6 to 8 hours, or high lactate levels complicate hyperthermia, mortality is predictably increased. A continued rise in growth hormone levels despite therapy has been reported to be associated with a worse prognosis [72].
With respect to morbidity, neurologic function usually rapidly returns to normal after restoration of euthermia; however, some patients may be left with a mild cerebellar disorder [73]. Hepatic and renal failure in mild and moderate cases is usually completely resolved. Moderate muscle weakness may persist for several months in patients with severe muscle damage.
Although it has not been proven, patients who have experienced hyperthermic crises should be considered at high risk to develop a recurrence on exposure to similar heat stresses and should be advised accordingly.
Malignant Hyperthermia
Malignant hyperthermia is a drug- or stress-induced hypermetabolic syndrome characterized by vigorous muscle contractions, an abrupt increase in temperature, and subsequent cardiovascular collapse. Malignant hyperthermia occurs, on average, in 1 of every 50,000 to 150,000 adult patients given anesthesia [71,74]. With treatment, mortality is between 10% and 30% [75].
Cause and Pathogenesis
The cause of the temperature increase in malignant hyperthermia is similar to that of exertional heat stroke: Increased thermogenesis overwhelms the patient’s ability to dissipate heat. When exposed to various drugs, muscles may develop sustained or repeated contractions (Table 66.3). Current evidence indicates that patients with malignant hyperthermia have a defect in calcium metabolism in skeletal muscle cell membranes [76,77,78,79]. In most cases, a defect in the ryanodine receptor (RYR1) results in release of calcium from the sarcoplasmic reticulum, resulting in muscle contraction and heat generation [80]. Heat production occurs due to sustained or repetitive muscular contractions with hydrolysis of adenosine triphosphate and the activation of catabolic pathways, hepatic and muscular glycogenolysis, and catecholamine-induced accelerated turnover of substrates and metabolism of lactate.
Although halothane and succinylcholine are involved in more than 80% of cases, malignant hyperthermia has developed after the use of many other agents as well (see Table 66.3). Stress, excitement, anoxia, viral infections, and lymphoma have also been reported to trigger malignant hyperthermia [81,82]. Some data suggest that conditions of ischemia or hypoxia are the common triggers to hyperthermia in susceptible individuals. It is generally assumed that the causes of heat stroke would also increase the likelihood of malignant hyperthermia in susceptible individuals (see Table 66.1).
Table 66.3 Drugs and Malignant Hyperthermia | |
---|---|
|
The hyperthermic reaction to anesthetics is not allergic in nature; patients may have received the same anesthetic previously or may be exposed later without developing a reaction. There is little evidence that impaired heat dissipation or altered hypothalamic regulation is instrumental in producing acute hyperthermia in these patients. However, sympathetic activity and heat dissipation may be abnormal during exercise [83].
Pathophysiology
Direct thermal injury is the predominant cause of toxicity in malignant hyperthermia. Damage results from the metabolic consequences of a sudden increase in temperature to levels frequently above 42°C. Physiologic and pathologic changes parallel those described for patients with exertional heat stroke [84]. DIC, hepatic failure, seizures, ventricular dysrhythmias, and electrolyte abnormalities are more common and severe than in heat stroke.
Vigorous muscle contracture at the onset of malignant hyperthermia almost immediately precipitates a severe metabolic acidosis, with increased CO2 production and compensatory hyperventilation. High elevations of creatine kinase, lactate dehydrogenase, and aldolase are present [65] and reflect ongoing rhabdomyolysis. Hyperkalemia follows within minutes to hours [76]. Renal failure frequently occurs in malignant hyperthermia, most likely secondary to pigment load. Dehydration and low cardiac output do not contribute until later. The degree of hypocalcemia, hypophosphatemia, and hyperkalemia varies with the duration and peak of hyperthermia and degree of secondary myonecrosis. All three are more severe in malignant hyperthermia than in heat stroke. Direct thermal injury producing cerebral edema and cerebral hemorrhage results in coma. Seizures occur in most uncontrolled cases. DIC is a nearly universal finding [85]. Initially, volume status is normal because little volume has been lost in sweat. Cardiac output increases to meet metabolic demands and in response to the vasodilation of muscle beds. Sinus tachycardia, supraventricular tachyarrhythmias, and ventricular fibrillation occur soon after temperature exceeds 40°C. Tissue hypoxia, acidosis, and hyperkalemia make ventricular arrhythmias common. Because higher maximal temperatures are usually seen in malignant hyperthermia, hepatic failure and GI tract bleeding are more prominent than in heat stroke [85]. In survivors, hepatic necrosis and cholestasis peak in 2 to 3 days and may be severe.
Diagnosis
The metabolic predisposition to malignant hyperthermia appears, in general, to be inherited in an autosomal dominant fashion, with variable penetrance and expressivity. Although multiple screening strategies have been attempted [76,86,87,88,89], tests using caffeine or halothane stimulation of excised muscle are the standard screening tests recommended by the Malignant Hyperthermia Association of the United States [90]. Their false-positive rate is near 10% and false-negative rate near zero [91]. Because there is no one noninvasive test suitable for screening the general population, screening of family members of proven cases remains the best method of identifying susceptible individuals before hyperthermic crisis occurs. RYR1 gene mutation analysis of cells from buccal samples may help identify high-risk individuals. Only 25% of those susceptible have an identified mutation. Although an identified mutation would suggest susceptibility, the absence of a mutation would not rule out susceptibility [92,93].
Although malignant hyperthermia may occur under any severe stress, it most commonly follows administration of an anesthetic agent. Malignant hyperthermia occurs at any age but is most frequent in young patients; the mean age is 22 years, 65% of patients are male, 21% have had previous uneventful anesthesia, and 76% have no family history of malignant hyperthermia [94,95]. Early signs of hyperthermic crisis vary with the anesthetic agent administered but include masseter muscle contracture after the administration of succinylcholine, muscle rigidity, sinus tachycardia, supraventricular tachyarrhythmias, mottling or cyanosis of the skin, increased CO2 production, and hypertension. Hyperthermia is typically a late sign in an acute crisis, but it may be rapidly followed by hypotension, acidosis, peaked T-waves on the electrocardiogram owing to hyperkalemia, and malignant ventricular arrhythmias [85]. In one case report, desaturation measured by oximetry preceded temperature elevation by 40 minutes [96].
Two signs may be helpful in making a prehyperthermic diagnosis: increased end-tidal CO2 and masseter spasm [97,98,99,100,101,102,103]. Monitoring of end-tidal CO2 is recommended for all anesthetic procedures and is mandatory for patients at risk of malignant hyperthermia [91,94,95]. Severe masseter spasm after succinylcholine has been recognized as an early warning sign of malignant hyperthermia; however, the decision to discontinue anesthesia in patients with succinylcholine-induced spasm remains controversial [100,101,102,103,104]. If surgery must be continued, dangerous triggering anesthetics should be avoided, dantrolene should be given or at least be immediately accessible, and temperature and end-tidal CO2 should be monitored online.
Differential Diagnosis
Because malignant hyperthermia occurs almost exclusively in the perioperative setting, the differential diagnosis is more limited than that for heat stroke (see Table 66.2) [104]. Endocrinopathies and drug reactions, not infection, are the most frequent diseases in the differential diagnosis. Thyroid storm and pheochromocytoma may be very difficult to distinguish from malignant hyperthermia in the anesthetized patient [105]. Thyroid storm is now infrequent, owing to ease and extent of preoperative thyroid function test screening and prophylaxis of patients at risk. Dantrolene in doses used for malignant hyperthermia has been shown to decrease temperature in perioperative thyroid storm [106]. The temperature rise in pheochromocytoma is typically much slower than that in malignant hyperthermia [107]. Hyperthermia, owing to narcotic administration in patients taking monoamine oxidase inhibitors, also must be considered.
Treatment
Dantrolene, a hydantoin derivative, acts by uncoupling the excitation–contraction mechanism in skeletal muscle and lowering myoplasmic calcium. This action is now known to take place directly at the RYR1 receptor [108]. Dantrolene used for less than 3 weeks rarely causes toxicity [109]. In an acute crisis, 1.0 to 2.5 mg per kg of fresh dantrolene should be administered intravenously every 5 to 10 minutes. Effects may be seen 2 to 3 minutes after injection. Although cases that required 42 mg per kg have been reported [110], most authorities advise not to exceed 10 mg per kg [75,76,110,111]. The half-life of action is approximately 5 hours [76], and because relapse may occur, oral or IV dosages of 1 mg per kg IV or 2 mg per kg by mouth every 6 hours should continue for at least 24 to 48 hours [76]. Oral dantrolene provides excellent blood levels and may be substituted once the patient is alert [112]. With dantrolene, temperatures often rapidly decrease; without it, they may increase 1°C to 2°C every 15 minutes [95,97,110]. The cost of dantrolene is currently approximately $35 per 20-mg IV dose vial. As the diluent for dantrolene contains mannitol,
urine output should be carefully monitored. Because even minute quantities of the triggering agent may continue to produce the syndrome, anesthesia should be immediately stopped, and the anesthesia apparatus, tubing, and ventilation equipment should be immediately changed.
urine output should be carefully monitored. Because even minute quantities of the triggering agent may continue to produce the syndrome, anesthesia should be immediately stopped, and the anesthesia apparatus, tubing, and ventilation equipment should be immediately changed.

Full access? Get Clinical Tree
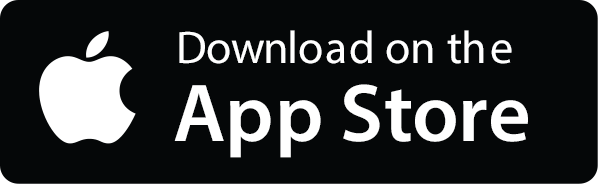
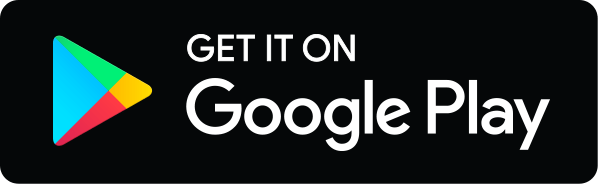