Disorders of Temperature Control Part I: Hypothermia
M. Kathryn Steiner
Frederick J. Curley
Richard S. Irwin
This chapter reviews the normal physiology of temperature regulation and the major hypothermic syndromes. Iatrogenic and intentional hypothermia are also reviewed. Three hyperthermic syndromes—heat stroke, malignant hyperthermia, and neuroleptic malignant syndrome—are reviewed in Chapter 66.
Normal Physiology of Temperature Regulation
The equilibrium between heat production and heat loss determines body temperature. In healthy, resting individuals, this equilibrium is tightly regulated, producing an average oral temperature of 36.60°C ± 0.38°C [1]. Table 65.1 is a conversion chart of temperatures in Celsius to Fahrenheit. Small shifts of this temperature set point occur, with a normal diurnal variation producing a peak temperature usually near 6:00 PM. Minute-to-minute changes in body temperature are quickly sensed, and appropriate changes are made in body heat production and loss to restore a normal balance.
Heat Production
In a neutral environment (28°C for humans), humans generate all net body heat from the energy released in the dissociation of high-energy bonds during the metabolism of dietary fats, proteins, and carbohydrates. At rest, the trunk and viscera supply 56% of the body heat, but during exercise up to 90% may be generated by the muscles. Although shivering or an increase in muscle tone may produce a fourfold rise in net heat production [2], vigorous exercise may cause a sixfold increase.
Heat Loss
Under usual environmental conditions, heat exchange with the environment takes the form of heat loss. Heat may be exchanged by radiation, conduction, convection, or evaporation [3,4,5,6]. Radiation exchange—the transfer of thermal energy between objects with no direct contact—accounts for 50% to 70% of heat lost by humans at rest in a neutral environment. Conduction involves the direct exchange of heat with objects in direct contact with the body. Large quantities of heat may be rapidly exchanged when the body is submerged in water; this is due to the much greater thermal conductivity of water as compared with air. Convection involves the exchange of heat with the warmer or cooler molecules of air that pass by the skin. Heat exchange by this mechanism increases rapidly with greater temperature differences between the skin and the air and with rapid airflow. Evaporative heat loss in humans occurs primarily through perspiration. Evaporation of sweat from the skin requires that energy be supplied by the skin, resulting in a net loss of heat from the body of 0.6 kcal per g of sweat absorbed. Unlike the other methods of heat exchange, evaporation can exchange heat loss even when a warmer environment surrounds the skin. Therefore, evaporation is the major means by which the body prevents hyperthermia in a warm environment.
Temperature Control Systems
The anatomy and regulation of the system that controls body temperature have been reviewed in depth by several
investigators [2,3,4,5,6], as outlined in the previous edition and are only briefly described here. Neurons that are directly responsive to temperature ascend from the skin, the deep viscera, and the spinal cord through the lateral spinothalamic tract to the preoptic anterior hypothalamus. When the hypothalamus perceives a temperature increase, it modulates autonomic tone to produce (a) an increase in evaporative heat loss through increased sweat output by the body’s 2.5 million sweat glands, (b) cutaneous vasodilation that allows direct flow of heat to the skin to increase convective and conductive heat losses, and (c) decreased muscle tone and activity to prevent any unnecessary heat production. When the hypothalamus perceives a temperature decrease, it modulates autonomic tone to cause (a) sweat production to cease or decrease, (b) cutaneous vasculature to constrict, and (c) muscle tone to increase involuntarily and shivering to begin.
investigators [2,3,4,5,6], as outlined in the previous edition and are only briefly described here. Neurons that are directly responsive to temperature ascend from the skin, the deep viscera, and the spinal cord through the lateral spinothalamic tract to the preoptic anterior hypothalamus. When the hypothalamus perceives a temperature increase, it modulates autonomic tone to produce (a) an increase in evaporative heat loss through increased sweat output by the body’s 2.5 million sweat glands, (b) cutaneous vasodilation that allows direct flow of heat to the skin to increase convective and conductive heat losses, and (c) decreased muscle tone and activity to prevent any unnecessary heat production. When the hypothalamus perceives a temperature decrease, it modulates autonomic tone to cause (a) sweat production to cease or decrease, (b) cutaneous vasculature to constrict, and (c) muscle tone to increase involuntarily and shivering to begin.
Table 65.1 Fahrenheit to Celsius Temperature Conversions | ||||||||||||||||||||||||||||||||||||||||||||||||||||||||
---|---|---|---|---|---|---|---|---|---|---|---|---|---|---|---|---|---|---|---|---|---|---|---|---|---|---|---|---|---|---|---|---|---|---|---|---|---|---|---|---|---|---|---|---|---|---|---|---|---|---|---|---|---|---|---|---|
|
The monoamines, baroreceptor data, hypothalamic calcium and sodium concentrations, and inflammatory cytokines (interleukin-1, interleukin-6, tumor necrosis factor-α [TNF-α]) are believed to be modulators of the anterior hypothalamic thermostat. They produce effects slowly and they have little to do with the regulation of acute temperature changes.
Voluntary responses play an important role in thermoregulation. Humans may respond to thermal stress by (a) adding or removing clothes (affecting evaporative, conductive, and radiant heat exchange), (b) moving to a warmer or cooler climate, (c) changing the level of activity, and (d) changing posture. Impairment of voluntary control places an unnecessary stress on autonomic control mechanisms and thereby predisposes to an imbalance in heat exchange and a change in body temperature.
The ability to regulate temperature effectively declines with age [7,8], probably as a result of deterioration in sensory afferents. Although younger individuals usually notice temperature changes as low as 0.8°C, older persons may not notice changes of up to 2.3°C. Moreover, because the sweat threshold increases and sweat volume decreases with age, an older individual may be more susceptible to hyperthermia than a younger person [9]. Old age may also be a liability for hypothermia because of (a) a lower basal metabolic rate, (b) a higher heat conductance due to a decline in body mass, (c) a decrease in the heat generated by shivering due to a smaller muscle mass, and (d) an inability to vasoconstrict cutaneous vessels in response to cold. In the elderly, restricted mobility or deterioration in cortical function can lead to a greater impact on the voluntary responses to temperature changes compared with the young.
Unintentional Hypothermia
Hypothermia, defined as a core temperature less than 35°C, may occur at all ambient temperatures and in patients of all ages but more commonly in the elderly. Hypothermia often occurs within 24 hours of admission in more than 3% of intensive care unit admissions [10]. Hypothermia is a diagnosis that is frequently missed and underreported. When all data are reviewed, the overall mortality from hypothermia in the United States has been conservatively estimated at 30 deaths per 1 million population per year [11]. The mortality for treated hypothermia ranges from 12% [12] to 73% [13].
Causes and Pathogenesis
The most frequent causes of hypothermia appear to be exposure, use of depressant drugs, and hypoglycemia. Understanding the causes of hypothermia (Table 65.2) and their pathogenesis enables one to develop a rational approach to treatment.
Exposure to Cold
Wet, wind, and exhaustion contribute to increased loss of body heat. Wet clothing loses 90% of its insulating value [14], rendering soaked individuals effectively nude. Exposure to rain or snow contributed greatly to the development of hypothermia in 15 of 23 incidents in hikers discussed in one review [14]. Convective heat loss because of wind may increase to more than five times baseline values, increasing with wind velocity [15]. Hikers with poor selection of clothing, campers who fail to seek appropriate shelter, or skiing in unfavorable weather can result in fatal hypothermia [15]. Victims of hypothermia display inappropriate behavior that worsens hypothermia. Up to 25% may remove their clothing and burrow, hiding under a bed or on a shelf [16]. Many quickly experience loss of coordination and then stupor or collapse. Death may occur within an hour of the onset of symptoms [15]. Immersion in water at a temperature colder than 24°C leads to extremely rapid heat loss. Core temperature drops at a rate proportional to the temperature of the water [17]. Although survival times of 1 to 2 hours have been reported for individuals immersed in water at 0°C to 10°C, death may occur within minutes.
Drugs
Alcohol, phenothiazines, barbiturates, and paralytic agents frequently produce hypothermia by depressing sensory afferents, the hypothalamus, and effector responses. Alcohol impairs the perception of cold, clouds the sensorium, and acts as a direct vasodilator [18,19]. Alcoholics are also thought to be more susceptible to exposure because of a state of relative starvation, increased conductive losses from decreased subcutaneous
fat, and high levels of blood alcohol that potentially impair the metabolic response to hypothermia by decreasing blood sugar and increasing acidosis. Most sedative–hypnotic drugs, such as barbiturates and phenothiazines, cause hypothermia by inhibiting shivering and impairing voluntary control. Phenothiazines increase the threshold necessary to produce shivering and lead to hypothalamic depression [10,20]; barbiturates decrease effective shivering [21]. Paralytic agents used to suppress ventilation prevent shivering and eliminate all voluntary control mechanisms [22,23]. Unexplained hypothermia has resulted from the administration of common antibiotics, such as penicillin [24] and erythromycin [25]. Bromocriptine may cause hypothermia by altering central dopaminergic tone [26].
fat, and high levels of blood alcohol that potentially impair the metabolic response to hypothermia by decreasing blood sugar and increasing acidosis. Most sedative–hypnotic drugs, such as barbiturates and phenothiazines, cause hypothermia by inhibiting shivering and impairing voluntary control. Phenothiazines increase the threshold necessary to produce shivering and lead to hypothalamic depression [10,20]; barbiturates decrease effective shivering [21]. Paralytic agents used to suppress ventilation prevent shivering and eliminate all voluntary control mechanisms [22,23]. Unexplained hypothermia has resulted from the administration of common antibiotics, such as penicillin [24] and erythromycin [25]. Bromocriptine may cause hypothermia by altering central dopaminergic tone [26].
Table 65.2 Causes of Unintentional Hypothermia | |
---|---|
|
Endocrine Dysfunction
Diabetic ketoacidosis, hyperosmolar coma, and hypoglycemia are frequently reported causes of hypothermia [18]. In one survey, 20% of patients with blood glucose levels less than 60 mg per dL had temperatures of less than 35°C. Hypoglycemia lowers cerebral intracellular glucose concentrations and impairs hypothalamic function [27]. In acute hypoglycemia (e.g., insulin administration), hypothermia occurs due to peripheral vasodilation and sweating. At glucose concentrations less than 2.5 mmol per L, subjects fail to perceive cold environments and fail to shiver [28]. This impairment appears transient because normal regulatory mechanisms and euthermia may be restored when normal serum glucose levels are restored.
The prevalence of hypothyroidism in patients ranges from 0% to 10%. Several patients with mild hypothyroidism have been safely rewarmed to euthermia without administration of exogenous thyroid hormone. In contrast, myxedema coma, a rare presentation of hypothyroidism, is associated with subnormal temperatures in 82% of cases [29]. It has a high mortality if not treated with exogenous thyroxine. Myxedema coma occurs most frequently in middle-aged to older women, and more than 90% of cases occur in winter [29]. Severe hypothermia with temperatures less than 30°C occurs in 15% of patients [29]. Coma arises because of a cerebral thyroxine deficiency. Hypothermia then results from a combination of loss of voluntary control mechanisms, from stupor or coma, decreased calorigenesis from thyroid deficiency, and decreased shivering, presumably from impaired hypothalamic regulation [29,30].
Panhypopituitarism and adrenal insufficiency are also rare causes of hypothermia. Unless profound insufficiency exists, these conditions rarely produce significant hypothermia in the absence of some other insult to the thermoregulatory system.
Central Nervous System Disorders
Diseases such as stroke, primary and metastatic brain tumors, luetic gliosis, and sarcoidosis may produce hypothermia by direct anatomic impingement on the hypothalamus [31,32]. Metabolic derangements from carbon monoxide poisoning or thiamine deficiency (Wernicke–Korsakoff syndrome) can also produce hypothermia, by affecting the hypothalamus [33,34,35,36,37,38]. Patients with anorexia nervosa have been shown to have multiple hypothalamic abnormalities resulting in the lack of shivering and vasoconstriction and a rapid drop in core temperature when they are exposed to cold [39]. Agenesis or lipoma of the corpus callosum has been reported to cause spontaneous periodic hypothermia by an unclear mechanism [21,40]. Several patients with multiple sclerosis have experienced transient hypothermia with flares of their neuropathy, suggesting the presence of hypothalamic plaques [41]. Drugs that are active on the central nervous system, such as neuroleptics or guanabenz, have resulted in hypothermia [42].
Spinal Cord Transection
Loss of skin and core temperature afferents, reduced body muscle mass, inability to shiver effectively, and, if mobility is compromised, inability to alter the environment make patients with spinal cord injury susceptible to thermal stress and hypothermia exposed to low ambient temperatures [43,44,45].
Skin Disorders
Skin disorders characterized by vasodilatation or increased transepithelial water loss may lead to hypothermia. Inappropriate conductive and convective heat losses in psoriasis, ichthyosis, and erythroderma have been shown to be associated with increased evaporative losses of up to 3 L per day; this computes to a potential loss of more than 1,700 kcal of heat per day [46,47]. Patients with extensive third-degree burns have been reported to have an even larger evaporative heat loss, losing up to 6 L fluid, or more than 3,400 kcal per day. When an additional cause of hypothermia is present, these patients may be in danger of severe drops in temperature. Heat loss and caloric requirements can be decreased dramatically by covering the skin with impermeable membranes to decrease evaporative losses [48,49,50].
Debility
Case reports suggest that hypothermia may occur in patients with debilitating illnesses such as Hodgkin’s disease [51]; systemic lupus erythematosus [52,53]; and severe cardiac, renal, hepatic, or septic failure. In Israel, 29% of hypothermic elderly individuals had preexistent renal failure [54]. The exact causes are unclear, but many mechanisms are likely acting in concert to produce a drop in temperature. A decrease in cardiac index from 2.8 to 1.4 L per minute results in a drop in temperature from 37°C to 35°C [55]. Temperature promptly rises when cardiac index increases. Hypothermia in hepatic failure might result from intermittent hypoglycemia. Most debilitated patients are also compromised by some degree of immobility or decreased voluntary control.
Trauma
Trauma patients often are hypothermic [56,57], due to multiple insults to the thermoregulatory system, for example, loss of voluntary control in adverse environments, the presence of alcohol in up to 62% of cases in some series, and the rapid transfusion of unwarmed blood [57]. In patients with moderately elevated injury severity scores, during the first day of hospitalization, 42% experience hypothermia, with 13% having temperatures less than 32°C [56]. The presence of shock [56] and massive transfusion [57] significantly contributed to the development of hypothermia in these patients.
Pathophysiology
Profound metabolic alterations occur in every organ system in response to a core temperature less than 35°C. Beyond the immediate cardiovascular changes induced by vasoconstriction, metabolic changes that appear to be temperature dependent occur in two phases: shivering and nonshivering. The shivering phase, usually occurring in the range of 35°C to 30°C, is characterized by intense energy production from the breakdown of stored body fuels. In the nonshivering phase, which occurs approximately less than 30°C, the metabolism slows down dramatically, resulting at times in multiple organ failure.
Shivering involves an increase in muscle tone and rhythmic contraction of small and large muscle groups. The metabolic changes during the shivering phase parallel those seen during muscular exercise. In different patient populations with
different measurement techniques, heat production has been shown to increase by four times the normal amount [58], oxygen consumption by two to five times [20], and metabolic rate by six times [59]. Central pooling of blood resulting from peripheral vasoconstriction may raise central venous pressure and slightly elevate cardiac output. Because cardiac output remains relatively close to normal and oxygen demand increases dramatically, mixed venous oxygen saturation decreases [60]. Although hepatic and muscular glycogenolysis may cause blood sugar levels to rise, this rise may not be seen in starved or exhausted patients or those with prolonged hypothermia [61,62]. The catabolism of fat increases the serum levels of glycerol, nonesterified fatty acids, and ketones. Anaerobic metabolism causes a rise in lactate levels; levels as high as 25.2 mmol per L have been reported [63]. The metabolic acidosis induced by this intense catabolism is compensated for the most part by the increased metabolism of lactate in the liver and increased minute ventilation [62]. Cortisol levels rise [13]. Most of these metabolic changes peak near 34°C or 35°C and become much less pronounced near a temperature of 30°C.
different measurement techniques, heat production has been shown to increase by four times the normal amount [58], oxygen consumption by two to five times [20], and metabolic rate by six times [59]. Central pooling of blood resulting from peripheral vasoconstriction may raise central venous pressure and slightly elevate cardiac output. Because cardiac output remains relatively close to normal and oxygen demand increases dramatically, mixed venous oxygen saturation decreases [60]. Although hepatic and muscular glycogenolysis may cause blood sugar levels to rise, this rise may not be seen in starved or exhausted patients or those with prolonged hypothermia [61,62]. The catabolism of fat increases the serum levels of glycerol, nonesterified fatty acids, and ketones. Anaerobic metabolism causes a rise in lactate levels; levels as high as 25.2 mmol per L have been reported [63]. The metabolic acidosis induced by this intense catabolism is compensated for the most part by the increased metabolism of lactate in the liver and increased minute ventilation [62]. Cortisol levels rise [13]. Most of these metabolic changes peak near 34°C or 35°C and become much less pronounced near a temperature of 30°C.
As core temperature falls toward 30°C, shivering nearly ceases and metabolism slows down dramatically. Near 30°C, metabolic rate approaches basal levels [64], and it may be half basal value by 28°C [59]. As shivering and metabolism slow down, oxygen consumption declines. At 30°C, oxygen consumption decreases to approximately 75% of basal value [64]; at 26°C to 35% to 53% [20]; and at 20°C to only 25% of basal value. This profound decrease in metabolism is reflected by changes in every organ system (Table 65.3).
Cardiovascular Function
Increasing degrees of hypothermia result in malignant arrhythmias, depressed cardiac function, and hypotension. A decrease in cardiac conductivity and automaticity [65,66,67] and an increase in refractory period [68,69] begin during the shivering phase and progress as core temperature decreases. The electrocardiogram (ECG) in mild hypothermia may show bradycardia with prolongation of the PR, QRS, and QT intervals. Below 30°C, first-degree block is usual, and at 20°C, third-degree block may be seen [61,70]. Below 33°C, the ECG commonly shows the characteristic J-point elevation (Fig. 65.1). As temperature drops below 25°C, the J wave increases [71,72], most prominent in the mid-precordial and lateral precordial leads [73]. J waves may persist 12 to 24 hours after restoration of normal temperature [74,75].
Atrial fibrillation is common at temperatures of 34°C to 25°C, and ventricular fibrillation frequently occurs at temperatures less than 28°C. The incidence of ventricular fibrillation increases with physical stimulation of the heart and is associated with intracardiac temperature gradients of greater than 2°C [76]. Purkinje cells show marked decreases in excitability in the range of 14°C to 15°C [67], and asystole is common when core temperatures drop below 20°C. Recovery of spontaneous electrical activity after hypothermic asystole may be related to protection from the calcium paradox afforded by hypothermia [77].
Table 65.3 Common Effects of Hypothermia | ||||||||||||||||
---|---|---|---|---|---|---|---|---|---|---|---|---|---|---|---|---|
|
Consequently, there is a gradual decrease in cardiac output. Systole may become extremely prolonged [78], greatly decreasing ejection fraction and aortic pressures. Ventricular compliance is severely reduced [79]. Output decreases to approximately 90% of normal at 30°C and may decrease rapidly at lower temperatures, with increasing bradycardia or arrhythmia. Regional blood flow is altered to preserve myocardial and cerebral perfusion [80]. Although blood pressure appears to be initially maintained by an increase in systemic vascular resistance (SVR) [81], systemic resistance decreases and hypotension is common [61] at temperatures less than 25°C. Oxygen demand usually decreases more rapidly than does cardiac output, causing mixed venous oxygen content to increase as the nonshivering phase begins.
Pulmonary Function
Pulmonary mechanics and gas exchange appear to change little with hypothermia [61,82,83,84]. Although the ventilatory response to an elevation in carbon dioxide tension (PCO2) may be blunted [82], there is no clear decrease in hypoxic drive [61]. As the increased oxygen demand and acidosis of the shivering phase decline, minute ventilation decreases. Tidal volume and respiratory rate decline at lower temperatures [20]. At 25°C, respirations may be only 3 or 4 per minute [19]; at temperatures less than 24°C, respiration may cease [59]. Apnea is presumed to be secondary to failure of respiratory drive at a brainstem level.
Renal Function
As blood pressure decreases during the nonshivering phase, glomerular filtration rate (GFR) may decrease by 85% [61] and renal blood flow by 75% [20], without a significant change in urine production. Maintenance of a good urine output, despite decreases in blood pressure and GFR in hypothermia, has been termed cold diuresis. This results from a defect in tubular
reabsorption. The urine may be extremely dilute, with an osmolarity of as low as 60 mOsm per L and a specific gravity of 1.002 [85]. The stimulus for this dilute diuresis may be the triggering of volume receptors as central volume increases with peripheral vasoconstriction [78], a relative insensitivity to antidiuretic hormone [75], or a direct suppression of antidiuretic hormone release [19]. Although kaliuresis and glycosuria may accompany the dilute diuresis, the net result for the patient is dehydration and a relatively hyperosmolar serum.
reabsorption. The urine may be extremely dilute, with an osmolarity of as low as 60 mOsm per L and a specific gravity of 1.002 [85]. The stimulus for this dilute diuresis may be the triggering of volume receptors as central volume increases with peripheral vasoconstriction [78], a relative insensitivity to antidiuretic hormone [75], or a direct suppression of antidiuretic hormone release [19]. Although kaliuresis and glycosuria may accompany the dilute diuresis, the net result for the patient is dehydration and a relatively hyperosmolar serum.
Neurologic Function
Hypothermic patients present with coma. Complete neurologic recovery has been described in hypothermic adults after 20 minutes of complete cardiac arrest [18] and after up to 3.5 hours of cardiopulmonary resuscitation (CPR) [85]. The mechanism by which hypothermia produces a seemingly protective effect is not well understood; it probably relates to a significant decrease in cerebral metabolism and a smaller injury by the no-reflow phenomenon [86], a mechanism whereby the brain is protected from injury until reperfusion.
Cerebral oxygen consumption decreases by approximately 55% for each 10°C decrease in temperature [87]. Cerebral blood flow decreases from 75% of normal at 30°C to only 20% of normal at 20°C [61]. The supply of nutrients and removal of wastes are adequate at these extremes given patient recovery and experimental evidence that the intracellular pH of brain tissue cooled to 20°C is unchanged even after 20 minutes of anoxia [88].
Visual [89,90] and auditory [91,92] evoked potentials demonstrate delayed latencies; latency increases as temperature decreases. The spectrum of electroencephalographic frequencies also changes with hypothermia. In healthy men cooled to 33°C by immersion, theta and beta activity increased by 17% and alpha activity decreased by 34% compared with control values [90]. Electromyography during hypothermia has been reported to be normal [93].
Hematologic Function
Hypothermia affects white blood cells (WBCs), red blood cells, platelets, and perhaps coagulation mechanisms. The WBC count in mild hypothermia remains normal to slightly elevated and drops severely at temperatures lower than 28°C [94,95]. The hematocrit usually rises in hypothermic patients at a temperature of 30°C in part due to hemoconcentration from dehydration caused by cold diuresis and in part due to splenic contraction [96]. The increase in blood viscosity in hypothermic patients appears to be due to decreased deformability of the red cell membrane [97]. After intravascular volume and euthermia have been restored, a mild anemia may last up to 6 weeks. Bone marrow aspirates obtained from these patients show erythroid hypoplasia and increased ringed sideroblasts, suggesting a maturation arrest [98]. Platelet counts drop as temperature decreases, and prolongation of the bleeding time has been noted at 20°C [94]; normal levels and function return on rewarming [99]. The decrease in platelet count is thought to be secondary to hepatic sequestration.
Gastrointestinal Tract Function
Ileus, pancreatitis, and hepatic dysfunction accompany hypothermia. Ileus is present at temperatures 30°C and lower. Subclinical pancreatitis appears to be common. Although patients usually lack symptoms of acute pancreatitis, more than half have amylase elevations greater than 550 Somogyi units and up to 80% of patients who die of hypothermia have evidence of pancreatitis at autopsy [101]. The relationship between alcohol use and pancreatitis in these patients is unclear. Hepatic dysfunction occurs commonly and involves synthetic and detoxification abilities [20]. Profoundly hypothermic patients in whom an acidosis develops are less able to clear lactate. Postmortem studies of patients who died from exposure-induced hypothermia have emphasized that gastric submucosal hemorrhage is common [102]. Duodenal ulceration and perforation may also be seen [103].
Endocrine Function
Hypothermia directly suppresses the release of insulin from the pancreas and increases resistance to insulin’s action in the periphery [104,105]. The blood glucose level rises in early hypothermia, due to glycogenolysis and increased corticosteroid levels, and remains elevated because of a decreased concentration and the action of insulin. Elevations in blood glucose, however, are usually mild; only 9% of patients in one series had blood glucose levels higher than 200 mg per dL. Changes in thyroid and adrenal function occur, but they are less well defined. The responses to thyroid stimulating hormone (TSH) and adrenocorticotrophic hormone appear blunted [61]. In hypothyroid patients, TSH increases in response to cold [106]. Although corticosteroid levels vary a great deal among patients, they rarely appear to be severely depressed [62,107,108]. Urinary catecholamine levels are increased threefold to sevenfold on average in hypothermic deaths compared with death due to other causes [102].
Immune Function
Infection is a major cause of death in hypothermic patients. Hypoperfusion increases the risk of bacterial invasion in ischemic regions of the skin and intestine. Central nervous system depression reduces the cough reflex, leaving the patient more susceptible to aspiration pneumonia. A decrease in tidal volume and minute ventilation increases the risk of atelectasis, making subsequent infection possible. Survival in hypothermia varies directly with the severity of cold-induced granulocytopenia [95,109]. Evidence from hypothermic animals with induced sepsis indicates an impaired release of PMNs from the marrow [95], as well as delayed clearance of staphylococcal [110] and Gram-negative organisms from the blood. Ineffective clearance of organisms may permit a continued low-grade bacteremia [110]. Ineffective clearance probably relates to impaired phagocytosis, migration [111], and a decrease in the half-life of circulating PMNs in hypothermia [109]. Impaired killing of bacteria by pulmonary alveolar macrophages exposed to cold in vitro has been reported and presumably increases susceptibility to pneumonia. The role of changes in antigen–antibody interactions, known to be impaired by cold in vitro, has not been clearly defined in hypothermic patients. Wound healing is delayed in patients with mild perioperative hypothermia [112]. Cytokine production may be delayed and prolonged [113]. Few human data are available regarding the activation of inflammatory mediators in hypothermia. Interleukin-6 and TNF-α are assumed to play a role in modulating an inflammatory cascade that must occur with hypothermia. Interleukin-6 concentrations fall with rewarming [114]. Thus, the hypothermic host is more susceptible to invasion by pathogens and less equipped to defend itself if invasion occurs.
Drug Clearance
Little is known about the clearance of drugs in hypothermic adults. Complex interactions of reduced cardiac output, dehydration, slowed hepatic metabolism, decreased GFR, abnormal
renal tubular filtration and reabsorption, and altered protein–drug dissociation constant alter the volume of distribution and total body clearance of many drugs [115]. The half-life of thiopental has been shown to increase 4 to 11 times at 24°C [20]. Because bile flow may be reduced by up to 75% at similar temperatures, excretion of toxins in the bile is also decreased [20].
renal tubular filtration and reabsorption, and altered protein–drug dissociation constant alter the volume of distribution and total body clearance of many drugs [115]. The half-life of thiopental has been shown to increase 4 to 11 times at 24°C [20]. Because bile flow may be reduced by up to 75% at similar temperatures, excretion of toxins in the bile is also decreased [20].
Diagnosis
The diagnosis of hypothermia may be suggested by a history of exposure or immersion, clinical examination, and laboratory abnormalities. Elderly, alcoholic, diabetic, quadriparetic, or severely debilitated patients are at high risk of hypothermia. Signs of hypothermia vary with the patient’s temperature. Cool skin, muscle rigidity, shivering, and acrocyanosis are present in most noncomatose patients. In obtunded patients, myxedema-type facies have been reported [101,116]. Although mental status changes vary widely among patients, they follow a typical pattern: between 35°C and 32°C, the patient may be stuporous or confused; between 32°C and 27°C, the patient may be verbally responsive but incoherent; and at temperatures less than 27°C, 83% of patients are comatose but able to respond purposefully to noxious stimuli [117]. Muscle tone remains increased after shivering stops. Reflexes remain normal until body temperature is lower than 27°C, when they become depressed and or absent. Plantar reflexes may be upgoing. The pupillary reflex may be sluggish below 30°C and may become fixed at temperatures less than 27°C. ECG changes are almost always present.
In the absence of an accurate temperature reading, the ECG can be used to gauge the degree of hypothermia [71,73]. J waves become prominent as temperature decreases and in the absence of a cerebrovascular accident appear to be pathognomonic for hypothermia. Prolonged PR or QT intervals in the presence of muscle tremor artifact and bradycardia strongly suggest the diagnosis. Because of the increased solubility of carbon dioxide and oxygen, blood gases reported at 37°C may show a value of partial pressure of oxygen (PO2) + PCO2 greater than 150 mm Hg on room air, a biochemical impossibility at euthermia. An elevated hematocrit, a good output of dilute urine with hypotension, ileus, and an elevated amylase are helpful but nonspecific indicators of hypothermia.
Because the symptoms of hypothermia frequently mimic those of other disorders, the diagnosis may be missed unless there is a clear history of exposure or an accurate temperature reading is taken. Thermometers calibrated to record temperatures less than 35°C must be used. Electronic temperature probes are accurate at low temperatures, can be used in several body sites, have a rapid response time, and can be left indwelling to provide online temperature readings during treatment. The lower temperature limit on individual probes must always be checked.
The site for recording the temperature is important (see Chapter 26). Oral or nasopharyngeal temperatures may not reflect core temperature because of the influence of surrounding airflow. Bladder, rectal, tympanic, esophageal, or great vessel temperatures are preferable. Bladder temperatures are accurate and convenient for initial measurements [118,119]. Great vessel temperature can be measured using the thermistor on a Swan-Ganz catheter. Esophageal temperature is mostly influenced by the inhalation of warmed air, great vessel temperature is highly affected by the infusion of heated fluids, and rectal temperature is greatly influenced by warmed peritoneal dialysis. During extracorporeal rewarming, bladder and pulmonary artery temperatures may increase faster than esophageal and rectal temperatures [119]. It may be helpful to monitor at least two core sites.

Full access? Get Clinical Tree
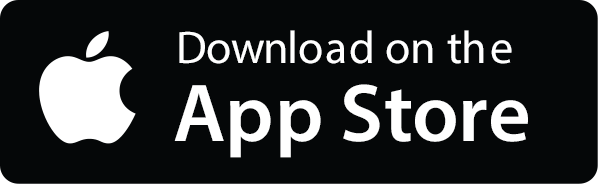
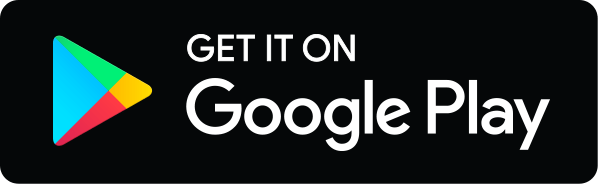