Disorders of Mineral Metabolism
Seth M. Arum
Daniel T. Baran
Disorders of mineral metabolism, although common, are rarely the primary cause of admission to an intensive care unit (ICU). However, these disorders frequently exacerbate life-threatening medical situations. Calcium, magnesium, and phosphorus are the main, clinically relevant minerals that can have an important impact on general health and on the course of a critical care admission. Calcium ions regulate membrane potentials, the coagulation cascade, neurotransmitter release, hormone-receptor interactions, and intercellular communication through channels and ion exchange. Magnesium is necessary for parathyroid hormone (PTH) secretion and maintenance of serum calcium, neuromuscular function, and membrane sodium-potassium adenosine triphosphatase (ATPase) activity. Phosphate, the major intracellular anion, is also instrumental for normal cellular function. It is a component of nucleic acids, phospholipids, and high-energy nucleotides and is necessary to facilitate oxygen delivery to cells. Phosphorus can also bind calcium in the body, and thus phosphorus metabolism is related to calcium and magnesium homeostasis. Therefore, symptoms of abnormal phosphorus metabolism often reflect the abnormalities in circulating calcium and magnesium. Calcium, magnesium, and phosphate balance are controlled through the interactions of PTH, 1α, 25-dihydroxyvitamin D (1,25 D), and calcitonin (CT).
Calcium Disorders
Calcium Physiology
Ninety-nine percent of total body calcium is stored in bone, whereas less than 1% is located in extracellular fluids. The calcium found in the extracellular fluids is either free (40%) or bound to albumin or other anions (60%) [1]. It is the free ionized calcium that is biologically active. Acid-base balance affects the binding of calcium to albumin. Hyperventilation, and the resultant respiratory alkalosis, enhances the binding of calcium to albumin, thereby acutely decreasing the ionized calcium and causing symptoms of hypocalcemia despite unchanged levels of total calcium. Similarly, changes in serum protein levels affect total serum calcium. The measured total calcium level in the serum can be corrected to account for changes in serum proteins by using the following formula:

Although the formula takes into account changes in serum proteins, it does not consider the impact of alterations in pH which frequently occur during acute illness. Measuring the ionized calcium directly is another option which should not be affected by either the serum proteins or the pH.
Calcium homeostasis is a function of absorption from the intestine (primarily the small intestine by active transport and facilitated diffusion), bone resorption/formation, and urinary excretion. The average diet contains 500 to 1,500 mg of calcium per day. In young individuals, the efficiency of intestinal absorption varies inversely with the amount of calcium ingested. Approximately 300 mg of calcium is exchanged daily between plasma and bone. Serum calcium is in equilibrium with intracellular calcium and calcium in bone and is filtered through the kidney. Urinary calcium excretion (normally 100 to 300 mg of calcium per day) depends on the glomerular filtration rate and the tubular sodium resorption. Loop diuretics enhance urinary calcium excretion in conjunction with their effect on sodium excretion. It is this property that serves as a useful adjunct to lower elevated serum calcium levels in the hydrated patient.
Hormonal Regulation of Calcium
Calcium absorption, excretion, and bone resorption/formation are in large part regulated by three hormones: PTH, 1,25 D, and CT.
Parathyroid Hormone
PTH is an 84-amino acid polypeptide produced and secreted by the chief cells of the parathyroid gland [1]. Secretion of PTH is stimulated by low levels of calcium in the cytoplasm of the parathyroid chief cells. The rapid release of PTH in response to hypocalcemia is essential for calcium homeostasis. The target organs for PTH are bone and kidneys. Chronic PTH secretion stimulates osteoclasts. This results in bone resorption and dissolution of hydroxyapatite crystals, resulting in the release of calcium and phosphate. This effect is augmented in the presence of 1,25 D. The renal effects of PTH include decreased proximal tubular reabsorption of phosphate (phosphate wasting), enhanced distal tubular calcium reabsorption (calcium retention), and increased renal mitochondrial 1α-hydroxylase activity (enhanced 1,25 D production). It is through the increased production of 1,25 D that PTH indirectly increases intestinal absorption of calcium.
Magnesium is mandatory for PTH secretion and end-organ response. Studies have shown that hypomagnesemia impairs PTH secretion and the renal response to PTH administration. Much of this seems reversible with magnesium repletion [2]. Clinically, correction of the hypocalcemia can often only be achieved after correcting the hypomagnesemia.
PTH can have both anabolic and catabolic effects on bone. Anabolic effects occur with intermittent administration of low-dose PTH [3]. In fact, daily subcutaneous injections of PTH derivatives have been shown to increase bone density and decrease fracture risk in various populations [4,5]. The catabolic, bone-resorptive effects of chronic PTH secretion are likely mediated through its effects on osteoprotegerin (OPG) and receptor activator of nuclear factor kappa ligand (RANK-ligand), resulting in increased osteoclast maturation and activity. Intermittent administration of PTH does not impact the OPG/RANK-ligand system as it does with constant administration, likely explaining the differing effects on bone mass [6].
Vitamin D
Vitamin D is a steroid hormone that is essential for calcium balance and is also likely important in numerous other cellular functions [7]. Activation of vitamin D requires 25-hydroxylation in the liver and 1-hydroxylation in the kidney to form the active hormone 1,25 D. Negative feedback is exerted by 1,25 D on its own production by suppressing 1-hydroxylase activity and stimulating the enzyme 24-hydroxylase to produce the biologically inactive steroids 24,25(OH)2D and 1,24,25 (OH)3D [8].
The effects of 1,25 D are exerted through interactions with nuclear receptors located in a variety of cells, including enterocytes, parathyroid chief cells, osteoblasts, and renal tubular cells. 1,25 D increases intestinal absorption of calcium and phosphate. It has also been shown to suppress PTH gene expression as a negative feedback mechanism [8].
Calcitonin
CT is a 32-amino acid polypeptide produced by the C-cells of the thyroid [9]. It is secreted in response to elevations in serum calcium. It can also be stimulated by certain gastrointestinal (GI) tract hormones (e.g., gastrin). The primary physiologic function of CT in humans remains unclear. Medullary carcinoma of the thyroid is a malignant neoplasm of the C-cells and is characterized by elevated CT levels. However, calcium, phosphate, and PTH levels remain normal. Also, patients can have undetectable levels of CT after a thyroidectomy with no clear detrimental systemic effects.
Despite the lack of clinical consequences from endogenous CT excess or deficiency, exogenous CT is a potent inhibitor of bone resorption. It also acts on the kidneys to enhance excretion of calcium, phosphate, magnesium, and sodium [9]. Both of these mechanisms make CT useful in the treatment of hypercalcemia. The effect of CT on bone resorption is lost over time due to tachyphylaxis [9]. This phenomenon, possibly due to downregulation of CT receptors, is of clinical importance when treating patients with hypercalcemia. The excellent short-term effects of CT to lower serum calcium (within 12 to 48 hours) allow the institution of therapies that require several days to attain maximal effectiveness (e.g., bisphosphonates).
CT can also be used in the treatment of osteoporosis. The administration of a salmon CT nasal spray has been shown to decrease markers of bone turnover, increase bone mineral density at the spine, and decrease the risk of vertebral fractures in postmenopausal women with osteoporosis [10].
Hypercalcemia
Hypercalcemia is an abnormality of the balance between different body compartments and can result from increased bone resorption, decreased renal excretion, increased GI absorption, or any combination of these mechanisms.
The signs and symptoms of hypercalcemia are protean and can be divided into four groups: (a) mental, (b) neurologic and musculoskeletal, (c) GI and urologic, and (d) cardiovascular. The mental manifestations of hypercalcemia include stupor, obtundation, apathy, lethargy, confusion, disorientation, and coma. In general, for a given level of hypercalcemia, older patients exhibit more of the mental signs than younger patients. The neurologic and musculoskeletal effects of hypercalcemia are reduced muscle tone and strength, myalgias, and decreased deep tendon reflexes. The GI and urologic signs are vomiting, constipation, polyuria, and polydipsia. The major cardiovascular effect of hypercalcemia, which the intensive care physician must address, is shortening of the QT interval. In the presence of ventricular ectopic beats, the calcium-induced shortening of the QT interval increases the potential for fatal arrhythmias or asystole.
Differential Diagnosis
Elevated serum calcium measurements have been reported in approximately 1% of the general population [11]. The causes of hypercalcemia can be differentiated into two broad groups defined by whether or not the process is driven by abnormal parathyroid tissue. Hence, the groups are termed: (a) PTH-independent hypercalcemia; and (b) PTH-dependent hypercalcemia. In PTH-independent hypercalcemia, the hypercalcemia is not mediated by abnormal parathyroid tissue, and the PTH level should be appropriately suppressed. In PTH-dependent hypercalcemia, the process is driven by abnormal parathyroid tissue, and the PTH level should be elevated, or inappropriately normal.
PTH-independent hypercalcemia is more common in hospitalized patients. Hypercalcemia of malignancy is the most common cause of PTH-independent hypercalcemia. The malignancies most often associated with hypercalcemia include lung (35%), breast (25%), hematologic (myeloma and lymphoma [14%]), head and neck (6%), and renal (3%) [12]. The hypercalcemia can be mediated by secretion of parathyroid hormone–related peptide (PTH-RP), most commonly seen in squamous cell carcinomas (often lung or head and neck tumors); autonomous activation of 1,25 D (occasionally seen with lymphomas); or by lytic bone lesions/metastases [13]. See Chapter 116 for a complete discussion of the hypercalcemia of malignancy.
Other possible causes of PTH-independent hypercalcemia include granulomatous diseases, immobilization, milk-alkali syndrome, thyrotoxicosis, vitamin D or A intoxication, or Addison’s disease.
Granulomatous diseases, such as sarcoidosis and tuberculosis, can cause hypercalcemia due to autonomous 1,25 D
production by the granulomas (similar to certain lymphomas). These patients have increased intestinal calcium absorption and sensitivity to vitamin D intake. Hypercalcemia occurs in 10% of patients, though hypercalciuria has been documented in as many as 20% [14].
production by the granulomas (similar to certain lymphomas). These patients have increased intestinal calcium absorption and sensitivity to vitamin D intake. Hypercalcemia occurs in 10% of patients, though hypercalciuria has been documented in as many as 20% [14].
Immobilization causes hypercalcemia as a result of decreased bone formation and persistent bone resorption. Hypercalcemia in the immobilized individual occurs most commonly in patients with high bone turnover (e.g., adolescents during the growth spurt or individuals with Paget’s disease or thyrotoxicosis).
PTH-dependent hypercalcemia is much more common in the outpatient setting, though these patients can be hospitalized due to other issues. PTH-dependent hypercalcemia can be caused by primary or tertiary hyperparathyroidism. Another possible cause is familial hypocalciuric hypercalcemia (FHH). The routine measurement of serum calcium has altered the clinical presentation of hyperparathyroidism with most patients presenting with asymptomatic hypercalcemia.
Primary or tertiary hyperparathyroidism results from autonomous secretion of PTH despite elevated serum calcium levels. The latter occurs typically after chronic secondary hyperparathyroidism in the setting of end-stage renal failure. Hypercalcemia develops due to increased bone resorption, increased intestinal calcium absorption from stimulation of 1,25 D production, and increased renal tubular calcium reabsorption. The patient can be hypophosphatemic due to the phosphaturic effect of PTH. The hormone also induces renal bicarbonate wasting, resulting in a mild hyperchloremic acidosis. In primary hyperparathyroidism, a single adenoma is present in 80% to 85% of cases, whereas four-gland hyperplasia occurs in 15% to 20% of cases [15]. Parathyroid cancer is present in less than 1% of these patients and typically presents with much higher serum calcium levels [16].
Parathyroid hyperplasia or adenomas can also occur as part of the multiple endocrine neoplasia (MEN) syndromes. Type I MEN involves tumors of the pituitary, pancreas, and parathyroid (usually hyperplasia), whereas type II is associated with pheochromocytoma, medullary cancer of the thyroid, and primary hyperparathyroidism (hyperplasia or adenoma).
FHH is an autosomal dominant disorder characterized by hypercalcemia with inappropriately normal or elevated PTH levels. It is usually caused by a mutation in the calcium-sensing receptor gene [17]. In contrast to primary hyperparathyroidism, patients with FHH have relative hypocalciuria (fractional excretion of calcium < 0.01), do not develop nephrolithiasis or bone disease, and cannot be cured surgically, unless rendered hypocalcemic by removal of all parathyroid tissue [18].

Full access? Get Clinical Tree
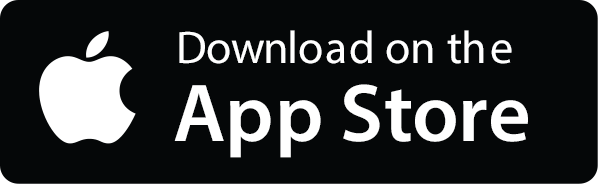
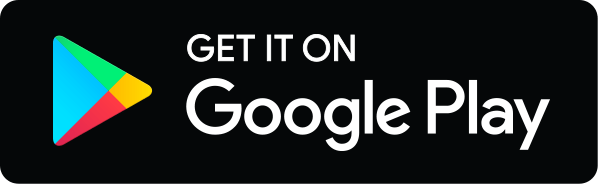
