Fig. 11.1
A schematic overview of the main principal coagulopathies in ICU critical patients. DIC disseminated intravascular coagulopathy, HIT heparin-induced thrombocytopenia, NOAC novel oral anticoagulant (Modified from Levi and van der Poll [11])
In patients with extracorporeal life support such as ECMO, the risk of coagulopathy is further increased by therapeutic anticoagulation using unfractionated heparin (UFH) to prevent blood clotting and by hemodilution, both resulting in the activation and consumption of coagulation factors and platelets [12, 13].
11.2.2 Limitations of Conventional Laboratory Coagulation Tests
Standard laboratory coagulation tests (prothrombin time, PT; activated partial thromboplastin time, aPTT; platelet count; fibrinogen plasmatic concentration; D-dimer) appear to be of limited value for the precise assessment and the timely monitoring of ICU coagulopathies and for their treatment strategies, in particular in patients with critical and ongoing bleeding [4, 8, 14]. Hypothermia-induced coagulopathy might be masked by the usual sample analysis at a standardized temperature of 37 °C. The conventional “static” tests (PT and aPTT) only partially reflect the initial thrombin generation; platelet count is merely quantitative and does not detect preexisting, drug-induced or perioperatively acquired platelet dysfunction. Moreover, conventional coagulation tests do not provide information about clot stability over time, giving a limited information regarding (hyper)fibrinolysis. It is therefore of paramount importance to acknowledge the intrinsic limitations of routine coagulation tests in detecting clinically significant conditions which may influence bleeding.
11.3 Point-of-Care Devices
For an appropriate and clinically useful hemostatic evaluation of the critically ill patient, the analysis of a rapid, reproducible and reliable hemostatic profile is mandatory. Differently from the standard tests, POC techniques, including whole blood platelet function tests (impedance or turbidimetric aggregometry) and particularly viscoelastic tests (thromboelastography/thromboelastometry: TEG®/TEM®) might provide detailed results, including the correction for the core temperature of the patient. When compared to conventional laboratory test, viscoelastic assay results are available much earlier (analysis time of 20–25 min vs. laboratory turnaround time of 40–90 min) and might identify in a large part of the cases the underlying hemostatic derangement (i.e. factor deficiency, low fibrinogen, altered platelet function, increased fibrinolysis). Therefore, they can provide the indication for a targeted drug therapy or the appropriate blood component administration [6, 15]. More importantly, the results of the hemostatic interventions are real-time available, an opportunity which might become critical in this setting (rapid display of the in vitro drug effect and of the in vivo results early after the therapeutic intervention). Viscoelastic POC techniques (ROTEM®, TEG®) are based on thromboelastography, which was described several decades ago by Hartert [16]. These tests are used to measure the time until clot formation begins, the dynamics of clot formation and the firmness and stability of clots over time. A special and unique advantage provided by the viscoelastic techniques is the direct detection of hyperfibrinolysis [17]. Platelet aggregation tests combined with viscoelastic methods yield a far broader diagnostic spectrum than conventional laboratory testing of coagulation may offer [15]. However, none of the currently available POC techniques can provide adequate information about all aspects of the complex process of blood clotting. For more detailed informations regarding the techniques, the points of strengths and limitations of POC devices, see elsewhere in Chaps. 2 and 3.
11.4 Coagulation and Inflammation: Relationships and Cross talk Pathways between the Two Systems
In recent years, evidences from basic researches and clinical studies focused on the profound relationships and the costantly cross talks between the coagulation system and the inflammatory cascade. This involves the cellular elements of the immune response, the released substances (i.e. cytokines, PAMPs and DAMPs, and more recently microparticles and histones; see below) [18], the endothelium with its complex system of signals’ mechano-transducers such as glycocalyx and receptors [19] and the inflammatory response to tissue damages, due to invading pathogens or sterile injuries [20]. Blood coagulation and inflammation represent the two main ancient human host defense systems, aiming to recognize, contain and kill invaders, trying to limit tissue injury and to start repair mechanisms [21, 22]. The interplay of the coagulation and inflammatory pathways shows a formidable complexity, in which laboratory researches and animal model studies offer even more elements to clinical queries to reach (possibly) solid evidences. The need is for biomarkers and rapid diagnostic POC tools. The ultimate goal is to define precise targets for appropriate drug delivery to guide a goal-directed treatment; the final result should be to modulate both coagulation and inflammatory systems’ responses and, definitively, to counteract possible impending bleeding situations.
11.4.1 Inflammatory Response in ICU Patients due to Surgery, Trauma, and Burns: Lessons Learned from Sepsis
Tissue injury following major surgery, trauma and burns represents a formidable boost of inflammatory response, with the host immune system involved both in protection from infection (a major factor of increased morbidity, mortality and overall costs) and in the initiation and guidance of the repair mechanisms aiming at the final “restitutio ad integrum”. Immunological variations following surgery and trauma are only partially understood. They appear as characterized by an increase of expression of T-helper 2 (Th2) lymphocytes with impaired cell-mediated immunity. Moreover, an imbalance between the pro- and the anti-inflammatory pathways of Th1/Th2 occurs, with changes over time, following activation of hypothalamic-pituitary-adrenal (HPA) axis and sympathetic adrenal system activation, with the release of cortisol and catecholamines [23]. In trauma patients, the host’s immune response is widely believed to follow a bimodal sequential organized forms, represented by the systemic inflammatory response syndrome (SIRS, a pro-inflammatory activated condition) followed by a series of mechanisms of compensatory anti-inflammatory response syndrome (CARS) to counteract, balance and dump the overwhelming and possibly dangerous inflammatory reaction. However, recent data in severely injured patients with blunt trauma and burns seem to suggest a different view and this paradigm may not be correct [24]. This proposed new model accounts for a simultaneous and rapid boost of innate immunity (with activation of both pro- and anti-inflammatory genes) and suppression of those genes involved in adaptive immune response. In this paradigmatic shift of view, those complicated recoveries are delayed, resulting in a prolonged and dysregulated immune-inflammatory state.
11.5 Clinical Scenarios of Critical ICU Patients with Coagulopathy
11.5.1 Perioperative Coagulation Management in Major Surgery and Trauma Bleeding Patients
By definition, the intraoperative and the postoperative phases are summarized together as “perioperative” period. “Surgical bleeding” from an uncontrolled source, with no clinical and laboratory evidence of coagulopathy, may present as an early pathomechanism of perioperative hemorrhage in ICU. During ongoing major bleeding requiring a massive transfusion approach, coagulopathy may develop in a high percentage of cases. Depending on the patient’s compensatory capability, bleeding situations with a blood loss exceeding 20 % of the blood volume may represent an emergency as well as a risk factor for postoperative anemia, tissue hypoperfusion, transfusion requirements and associated adverse events and, ultimate, coagulopathy [4]. All these factors are independent predictors for survival outcome in postoperative critical care patients and are major drivers for resource application and costs [4, 9, 14]. Moreover, as surgery and trauma injuries are not limited to previously healthy people, the increasing number of patients treated with oral anticoagulants and antiplatelet drugs poses an additional problem to clinicians. Diagnosis of perioperative coagulation derangements in major bleeding necessitating massive transfusion needs to be addressed by appropriate coagulation tests. Repeated coagulation monitoring is the rational basis for the introduction of prothrombotic and/or antithrombotic interventions. In the last decade, there has been a paradigmatic shift in the management of these patients, with an increased awareness for the limitations of routine coagulation tests to guide coagulopathy treatment in massive bleeding, pointing out that predominant pathomechanism(s) of bleeding in the complex scenario of perioperative coagulopathy cannot be differentiated. A prolonged activated partial thromboplastin time (aPTT) may be due to “intrinsic coagulation factor” deficiency requiring specific substitution, fibrinogen deficiency requiring fibrinogen substitution, hypothermia necessitating rewarming, residual heparinization requiring protamine reversal or hyperfibrinolysis requiring antifibrinolytic drugs. Normal viscoelastic assay results and normal platelet count/function are unlikely to coincide with bleeding (high negative predictive value), which should trigger a requirement of surgical re-exploration. In major trauma patients, coagulopathy is recorded in approximately 25–30 % of patients admitted to the emergency room, being involved in up to 40 % of all trauma-related deaths [25]. Although fresh frozen plasma (FFP) transfusion is often a routine part of transfusion protocols, its efficacy is uncertain. Most notably, FFP administration is also associated with acute lung injury (TRALI), transfusion-associated cardiac overload (TACO) and nosocomial infections [26, 27]. Additionally, trauma-associated coagulopathy is a very complex biological process; for the updated informations of early trauma-induced coagulopathy (TIC) and its detection and treatment approach, see Chap. 7. However, care of the most injured trauma patients does not end in the trauma resuscitation bay and/or in the operating room. ICU physicians must be skilled to receive patients at any point along the continuum of care, both with an assessment of the patient’s physiologic status and addressing ongoing needs in a prompt and rapid fashion [28]. Once the patient is more stable, the ICU physician must begin the mainstays of care focusing on clinical issues such as organ function support, potential for infectious, thromboembolic events and the need for planned re-exploration (e.g. in case of open abdomen strategy) and staged definitive repair.
The resuscitation strategies from surgery or trauma injury are based on the prerequisite of stopping hemorrhage. Life-threatening coagulopathy is one of the most dangerous complications of patients in severe hemorrhagic shock and increased transfusion requirements are generally predictive of subsequent organ dysfunction; moreover, there are data showing that persistent bleeding with ongoing coagulopathy of patients admitted to the ICU is independently associated with increasing in morbidity and mortality [29, 30]. In these critical care settings, there are more and more urgent needs for the ICU physicians for reliable POC hemostatic monitoring devices, with clinical relevance in conditions of severe coagulopathy due to massive hemorrhage. There is increasing evidence that viscoelastic monitoring systems such as TEG (Haemonetics Corp., Niles, IL, USA) and ROTEM (Tem Innovations GmbH, Munich, Germany) are useful for detecting clinically relevant hemostatic alterations in surgical and trauma patients with critical bleeding and diffuse coagulopathy [31, 32]. Viscoelastic monitoring has been much more widely used in Europe than in the United States, both for perioperative and ICU management of the hemorrhagic patients [3, 33, 34]. In the recent published ESA Guidelines on Perioperative Bleeding Management, the authors suggest the use of viscoelastic tests (ROTEM/TEG) for monitoring perioperative hemostatic function in major orthopedic surgery and neurosurgery (Grade of Evidence: 2C), as well as a recommendation in visceral and transplant surgery for a targeted coagulation disorders management (Grade of Evidence: 1C) [7].
11.5.2 Burn Injury
Severe burn injury (burned body surface area >15 % in adult and >10 % in children) triggers a SIRS with serious metabolic derangements. One of the best known systemic manifestations evidenced in the first hours after a major burn trauma is related to systemic microcirculation damage with increased capillary permeability and protein leakage into the interstitial space, with clinical evidence of huge generalized edema and hemodynamic features of hypovolemic shock. Adequate fluid replacement is mandatory in the first hours after a traumatic burn. However, in burn patients other systemic disorders are also accompanied by SIRS such as cardiac dysfunction, acute respiratory distress syndrome, acute renal failure, increased intestinal permeability resulting in bacterial translocation, hypermetabolism, hypercatabolism and sepsis [35]. These severe alterations in homeostatic balance may escalate in multiple organ failure (MOF) and death. Therefore, research seeking new mechanisms by which to attenuate inflammation after severe burn injury is urgently needed. The goal for early excision of burns is the reduction in release of inflammatory mediators and bacterial colonization of wounds. This currently worldwide adopted strategy can attenuate SIRS phenomena and reduce the incidence of metabolic derangements, sepsis and multiorgan failure. Once performed as early as possible, excisions and immediate wound closure have shown an improvement in survival, with a decrease in ICU and hospital length of stay [36]. Once activated, inflammation and coagulation systems in septic burn patients can definitively lead to increased mortality [37]. Coagulation system derangements during the early phases of burn injury are characterized by activation of procoagulation pathways, enhanced fibrinolysis and consumption of natural anticoagulant factors. Both the thrombotic and fibrinolytic pathways are triggered proportionally to the degree and the extent of the burn [38–40]. Conflicting results about a possible, early acute burn-induced coagulopathy with similarities to TIC are reported. Sherren and associates showed that, in major burns, the dysfunction of the procoagulant, anticoagulant and fibrinolytic pathways may occur early [41], arguing that, to date, the existent trials were focused on the incidence and the time course of DIC and a hypercoagulable state. The initial coagulation test reported values referred to the first 24–36 h; therefore, if any laboratory evidence of the coagulation derangements was observed, it was often delayed [42, 43]. Lu and associates found in their single-center, retrospective study that the screening hematologic profile of burn patients at admission was normal and the standard screening assays did not suggest the existence of an admission coagulopathy analogous to TIC [44]. The current trend for increased survival in burn patients has been attributed to various improvements in care, including early total burn wound excision and grafting, both with dedicated intensive care units and an improved infection control due to a more coordinated multidisciplinary approach [45, 46]. A major challenge for the anesthesiologists regards blood loss and transfusion management during early burn wound excision. The surgical approach is often associated with severe bleeding [47]. Despite multiple attempts to reduce blood losses, patients still need large amounts of allogeneic blood products both with blood derivatives (red blood cells, FFP, platelet concentrates and coagulation factor concentrates) [48–50]. Schaden and associates reported data from a prospective, single-center, randomized study focused on the management of coagulation disorders through a treatment algorithm centered on POC (ROTEM) evaluation, aiming at reduction of allogeneic blood products transfusions during surgical burn wound excisions [51]. The primary endpoint was the evaluation of the cumulative transfused number of allogeneic blood units on the day of surgery. Given the limitations due to the power of the study, a significant decrease in blood product transfusions was observed in the ROTEM-based algorithm group. No FFP was administered in the algorithm group. Low fibrinogen levels or hyperfibrinolysis was not significant pathomechanisms requiring goal-directed treatment.
11.5.3 Severe Sepsis
Hemostatic derangements represent a common early event in patients with severe sepsis. Sepsis is characterized by a procoagulant state and the resultant hypercoagulability may lead in the most severe cases to DIC. An excessive activation of the coagulation system involves platelets and coagulation factor consumption, which may shift the hypercoagulant state into a hypocoagulant state, ultimately leading to the hemorrhagic complications [52].
For the diagnosis of DIC, a simple scoring system has been developed from the International Society of Thrombosis and Haemostasis, which can be adopted and repeated daily for the management of these patients [11].
In clinical practice, it is well known that commonly used biomarkers of sepsis such as procalcitonin and interleukin-6 (IL-6) may also increase in patients with trauma or surgery even without infection. However, thromboelastometry variables may play a potential role as early coagulopathy biomarkers in critical septic patients [53]. Adamzik and associates [54] reported in their observational cohort study (56 patients with severe sepsis and 52 patients after major surgery) that the thromboelastometry-derived lysis index was a more reliable biomarker of severe sepsis compared with procalcitonin, IL-6 and C-reactive protein. More interestingly, a multivariate analysis of another cohort study of 98 septic patients evidenced that the absence or presence of at least one pathological thromboelastometry variable allowed better prediction of 30-day survival in severe sepsis, compared with the simplified acute physiology system (SAPS) II and sequential organ failure assessment (SOFA) scores [55]. This again highlights the relevance of the coagulation system and the possible role in the next future of POC techniques in critical septic patients. In a very recent multicenter prospective observational study, Haase and associates investigated the outcome in patients with severe sepsis evaluated with repeated TEG measurements (standard TEG and functional fibrinogen) [56]. This was a subgroup of the 6S Trial, comparing hydroxyethyl starch (HES) vs. Ringer’s acetate as fluid resuscitation in severe septic patients [57]. Two hundred sixty patients were assessed consecutively for 5 days, and data including death and bleeding were retrieved from the original databases. For all TEG variables, a hypocoagulability state was significantly associated with an increased risk of death. Patients treated with HES had lower values of functional fibrinogen maximum amplitude compared with patients who received Ringer’s acetate with a consistent increased risk of bleeding (hazard ratio 2.43, 95 % CI 1.16–5.07). However, to date, a clear relationship between viscoelastic test variables and clinically relevant outcomes such as hemorrhage and mortality has not yet been demonstrated in patients with sepsis, because previous published studies were relatively low powered and only few made sequential measurements [58].
11.6 Therapeutical Options in Managing Coagulopathies in ICU Patients
The cornerstone of the therapeutical strategies in managing coagulopathy in the critical patients is that transfusional decisions should not be based only on coagulation test results, rather being guided by global clinical evaluation and possibly through an individualized approach. In a stable patient with no clinical signs of an ongoing bleeding, an unexpected abnormal coagulation test result must be repeated, in order to exclude possible sampling and/or laboratory errors.
The need to transfuse allogeneic blood products and/or blood derivatives is indicated for patients who are actively bleeding; actually, limited data exist regarding a prophylactic use of blood products in order to prevent a possible hemorrhage [2, 15]. Moreover, the very recent Practice Guidelines for Perioperative Blood Management by the ASA Task Force on Perioperative Blood Management emphasized new evidences including preoperative evaluation, assessment for transfusion risk and the use of adjunctive therapies to prevent and/or to treat bleeding [59]. These updated ASA guidelines promote the use of transfusion algorithms, in particular those based on thromboelastographic evaluations, blood ordering schedules and restrictive transfusion regimens. They advocate for implementing the adoption of pharmacologic therapies aiming to reduce allogeneic blood transfusions, using alternatives like coagulation factors concentrates (e.g. prothrombin complex concentrate) for rapid reversal of vitamin K antagonists or antifibrinolytic drugs during selected cardiac and noncardiac procedures with a high risk of bleeding.
A brief overview on the principal therapeutical options as blood components and blood derivates used in treating coagulopathy in the bleeding ICU patients is presented.
11.6.1 Fresh Frozen Plasma (FFP)
Transfusions with FFP are increased in recent years, with a usage up to 39 % of all patients admitted in the ICU [60, 61]. The most common clinical indications for FFP transfusion are prolonged PT or aPTT coagulation tests, meaning a decrease in coagulation factors with a major contribution and an increased risk for bleeding. FFP transfusion is able to increase the levels of coagulation factors normalizing coagulation tests and decreasing bleeding [62]. However, several observations should be applied to these rationales. A reduction in coagulation factor levels can increase the risk of bleeding but only when coagulation factors activity decreases below the minimum hemostatic threshold of 30 %, which correlates with an INR >1.7 [63]. FFP transfusions increase coagulation factor levels, but the effect on the laboratory tests (INR and aPTT) depends on two factors: the amount of transfused FFP (which is quite large to reach an effective result) and the degree of the coagulopathy [60]. Finally, a recent systematic review of 80 randomized trials failed to identify any evidence of effectiveness for FFP [64].
11.6.2 Thrombocytopenia and Platelet Dysfunction
For thrombocytopenia, the main trigger for transfusion occurs in approximately 35–45 % of the ICU patients; approximately, 15–30 % of patients who develop thrombocytopenia in the ICU will receive platelet concentrates [65, 66].
The rationales for transfusion of platelets in the critically ill patient are (1) a therapeutic intervention to stop bleeding, (2) prophylactically prior to a surgical intervention or (3) for patients at a high suspicion of bleeding risk including those with combined coagulation deficits, platelet dysfunction due to treatment with antiplatelet agents or renal disease.

Full access? Get Clinical Tree
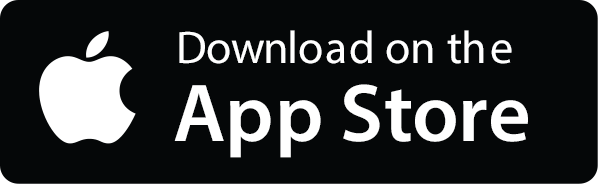
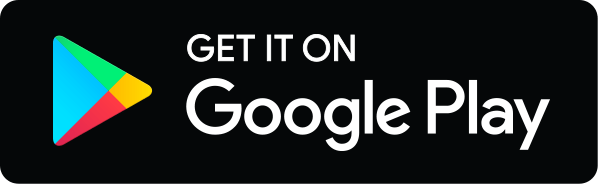