Disease-Specific Nutrition
Mickey M. Ott
Bryan R. Collier
Douglas Seidner
Introduction
In the critically ill patient, the constant barrage of multiple physiologic derangements quickly leads to malnutrition. The hypermetabolic response to stress changes the nutritional requirements of these individuals, but failure of the various organ systems complicates the issue. Renal, hepatic, and pulmonary function must be considered when prescribing nutritional therapy in the intensive care unit (ICU). This chapter will discuss the metabolic abnormalities associated with these disease processes, the nutritional assessment of the patient in organ failure, and propose evidence-based guidelines for nutritional support in these disease-specific populations.
Renal Failure
Despite many recent advances in medical therapy, management of the critically ill patient with renal failure remains a challenging endeavor. Acute renal failure (ARF) is associated with an overall mortality rate of 50% to 90%, depending on its derivations and comorbid conditions [1]. Hypotension and hypovolemia, secondary to excessive fluid losses, inadequate fluid replacement, or decreased cardiac output are the leading causes of renal failure in the ICU. Factors such as shock or sepsis and exposure to nephrotoxic drugs or blood transfusions can also predispose patients to renal dysfunction. Early diagnosis and restoration of circulating blood volume to the kidneys may decrease the risk of permanent damage; however, the course to renal recovery is often a complicated one. The patient in chronic renal failure (CRF) is also at increased risk for morbidity, as these patients will likely present with protein-calorie malnutrition at baseline. Moreover, the nutritional support of the patient on dialysis will offer a unique challenge to the critical care physician.
Malnutrition and Hypermetabolism
In general, renal failure is characterized by altered nutrient metabolism, defective metabolic waste excretion, inadequate nutrient intake, and excessive nutrient losses. Approximately 10% to 70% of patients with CRF undergoing maintenance dialysis are severely malnourished [2,3]. In these patients, malnutrition is most often the result of poor dietary intake secondary to uremia-induced gastroparesis, poor-tasting highly restrictive diet prescriptions, and medications with gastrointestinal side effects. Diminishing creatinine clearance levels have been linked to a spontaneous decline in the dietary protein intake of CRF patients as well [3,4]. In addition, patients with acute renal failure and critical illness represent by far the largest group receiving supplemental nutrition [5].
The dialysis dose also plays a significant role in the development of malnutrition. The protein catabolic rate of patients undergoing dialysis can be calculated to estimate daily protein intakes of these individuals [6]. In a 1983 investigation by Acchiardo et al., daily protein intakes of less than 0.8 g per kg, as measured by protein catabolic rate, correlated with an increased morbidity and mortality rate compared to patients with greater protein intakes [7]. A subsequent study by Lindsey and Spanner demonstrated a strong linear relationship between dialyzer urea clearance, duration of dialysis, and volume dialyzed (collectively expressed as Kt/V) and protein catabolic rate [8]. It is suggested by this correlation that an adequate dose of dialysis is influential on sufficient nutrient intake and the prevention of malnutrition in chronic dialysis patients [9].
Critical illness imposes an even greater metabolic stress and nutritional demand on the patient with renal dysfunction. Protein-calorie malnutrition (PCM) is reportedly present in 25% to 60% of individuals undergoing continuous renal replacement therapy (CRRT) within the intensive care unit [10]. It is important to note that the increased energy expenditure seen in these patients is a direct result of the hypermetabolic response to infection and injury and not of the renal failure itself. Indirect calorimetry has been used to show that the intensity of renal dysfunction has no direct bearing on energy expenditure [11]. Renal failure is, however, the root of several metabolic alterations that often interfere with nutritional status and overall stability of the critically ill patient.
Metabolic Abnormalities
Common metabolic abnormalities associated with ARF include glucose intolerance, impaired lipolysis, increased protein catabolism, decreased protein synthesis, fluid and electrolyte imbalance, and metabolic acidosis (Table 192.1). Although renal excretion of insulin is diminished, insulin resistance coupled with the stress of sepsis or injury can lead to significant hyperglycemia in this patient population. Decreased activity of lipolytic enzymes, such as hepatic triglyceride lipase and lipoprotein lipase, may reduce clearance of parenterally infused triglycerides by as much as 60% in ARF patients versus controls with intact renal function [12]. Adequate energy provision may thus be hindered by altered carbohydrate and fat metabolism. Nonprotein calorie requirements in ARF patients are best met with formulas providing mixed substrates in the ratio of 50% to 70% as carbohydrate and 30% to 50% as fat [13].
Table 192.1 Metabolic Responses to Acute Renal Failure | ||||||||||||||||||||||||||||||||||||
---|---|---|---|---|---|---|---|---|---|---|---|---|---|---|---|---|---|---|---|---|---|---|---|---|---|---|---|---|---|---|---|---|---|---|---|---|
|
Several factors contribute to increased protein catabolism and overall negative nitrogen balance in ARF patients. In accordance with the metabolic response to injury, patients with renal failure experience an increase in gluconeogenesis, leading to the breakdown of skeletal muscle proteins for use as energy and for synthesis of acute-phase proteins. Metabolic acidosis, frequently seen in renal failure, can trigger skeletal muscle protein breakdown as well. Reduction in muscle protein synthesis in this population has been linked to diminished cellular uptake of glucose and amino acids secondary to insulin resistance, altered cellular ion transport mechanisms, and defective intracellular synthesis [14,15].
Varying protein and energy provisions also influence protein catabolism and nitrogen balance in ARF patients. A 1996 investigation of 40 ICU patients with ARF receiving continuous venovenous hemofiltration revealed that at levels of protein administration above 1.5 g per kg per day, increasing energy provisions are associated with an increase in protein catabolism [16] (Fig. 192.1). Increasing energy provisions had a protein-sparing effect at lower levels of protein administration. Net nitrogen balance was also examined in this population (Fig. 192.2). Protein administration rates of 1.5 to 2.0 g per kg per day were associated with a positive net nitrogen balance,
although at these elevated levels of protein provision, lower-energy administration rates were necessary to prevent protein catabolism and promote more favorable nitrogen balance. Final nutrient recommendations were for 1.5 to 1.8 g protein per kg per day with energy levels between 25 to 35 kcal per kg per day in critically ill ARF patients on continuous venovenous hemodialysis (CVVHD) [5,16].
although at these elevated levels of protein provision, lower-energy administration rates were necessary to prevent protein catabolism and promote more favorable nitrogen balance. Final nutrient recommendations were for 1.5 to 1.8 g protein per kg per day with energy levels between 25 to 35 kcal per kg per day in critically ill ARF patients on continuous venovenous hemodialysis (CVVHD) [5,16].
Close monitoring of fluid status is crucial to the maintenance of adequate intravascular volume and renal perfusion. Fluid is typically restricted to 1.0 to 1.5 L per day in nondialysis anuric or oliguric patients. Concentrated enteral or parenteral formulas are often required to meet daily nutrient needs under these circumstances. Dialysis, with special emphasis on CRRT, allows for a liberalization of fluid provisions to thereby permit an adequate supply of protein and energy to the renal patient. In the ICU setting, ARF patients tend to be severely volume overloaded with fluid shifting to the extravascular space secondary to hypoalbuminemia. Even while on some form of CRRT, maintenance of fluid balance is challenging in these patients and importance should be given to adequate protein provision for repletion and reversal of the effects of low serum albumin levels. In CRRT, there is a loss of at least 0.2 g amino acids per liter of ultra filtrate (up to 10 to 15 g amino acids per day), and of 5 to 10 g per day of proteins. Vitamins are also lost in significant amounts; however, there does not appear to be lipid losses across the filter [5].
Serum electrolyte levels fall within a wide range of highs to lows depending on renal excretion, extent of catabolism, and type and duration of dialysis [17]. Increased catabolism of skeletal muscle protein releases phosphorus, potassium, and magnesium into the bloodstream, leaving elevated serum electrolyte values. Because of this, parenteral nutrition (PN) formulations for renal patients are often made with low levels of these cations. A 1998 case report demonstrated the dangers of undershooting electrolyte needs in a frequently malnourished CRF population [18]. Introducing a carbohydrate load parenterally or even enterally to a malnourished patient stimulates insulin release and cellular anabolism, thereby enhancing intracellular ion transport [19]. The subsequent decline in serum electrolyte levels with resulting clinical complications is referred to as the refeeding syndrome. This case study reported four CRF patients who developed significant hypophosphatemia after starting PN due to inadequate electrolyte provisions in combination with intracellular shifts [18]. It is thus recommended that dextrose infusions be started gradually and serum electrolytes be monitored closely to correct for potential abnormalities in this population. Depressed serum ionized calcium levels are a common result of hyperphosphatemia and uremia. Supplementation is most often necessary to prevent release of calcium from the bone. Multivitamin preparations standard to enteral and parenteral formulas are adequate for most ARF and CRF patients. Support exists in the literature that vitamin C should not exceed 30 to 50 mg per day, because inappropriate supplementation may result in secondary oxalosis. If signs of vitamin A or other toxicities are observed, daily provision may need to be withheld. The kidney normally excretes trace elements; however, excess accumulation in renal failure is unlikely as gastrointestinal tract losses also occur. The micronutrient milieu may also be affected by the mode and dose of renal replacement therapy. Recent data show that prolonged CRRT results in selenium and thiamine depletions despite supplementation at recommended amount [5].
Standard daily doses of trace elements may be safely given to most patients in renal failure. Iron deficiency anemia is a commonly documented finding among end-stage renal disease patients. Recent research has focused on anemia and carnitine, an amino acid with a central role in long-chain fatty acid oxidation. Deficiency of carnitine has been associated with dialysis, and supplementation of L-carnitine has led to the improvement
of hematocrit levels in HD patients [20]. Metabolic acidosis in this population is a result of diminished acid excretion, increased protein catabolism, and daily protein intake [21]. Bicarbonate should be given enterally or intravenously to maintain pH more than 7.2 or serum bicarbonate more than 17 mEq per L. When supplementing by PN, sodium or potassium acetate is given because bicarbonate forms an insoluble precipitate with calcium in PN solutions.
of hematocrit levels in HD patients [20]. Metabolic acidosis in this population is a result of diminished acid excretion, increased protein catabolism, and daily protein intake [21]. Bicarbonate should be given enterally or intravenously to maintain pH more than 7.2 or serum bicarbonate more than 17 mEq per L. When supplementing by PN, sodium or potassium acetate is given because bicarbonate forms an insoluble precipitate with calcium in PN solutions.
Nutrition Assessment
The identification of malnutrition and timely initiation of nutrition support in critically ill patients with renal failure may not only reduce their degree of protein depletion but also increase their chances for survival [1]. Unfortunately, common measures of nutritional status, such as serum albumin, serum transferrin, weight changes, and anthropometrics tend to fluctuate with the alterations in fluid balance inherent to this patient population. Despite this, serum albumin is considered the strongest laboratory predictor of mortality for hospitalized patients with renal failure [22]. Daily monitoring of weight and intake and output records can help to assess fluid balance in these individuals. It is essential to use a dry weight (i.e., free of edema or ascites) when examining alterations in weight status. The dry or adjusted body weight of a renal patient may then be used to more closely estimate daily nutrient needs.
Continuous Renal Replacement Therapy (CRRT) Versus Intermittent Hemodialysis (HD)
It is commonly thought that dialysis therapy can be relied on to correct many of the metabolic derangements associated with acute and chronic renal failure. This may be true under most circumstances; however, research and patient care experience have shown that patients receiving intravenous (IV) nutrition are at greater risk for fluctuating serum chemistries despite regular dialysis treatments [1,18]. HD can also increase the risk of hypotension and may add to the hemodynamic instability of the ICU patient by limiting the removal of adequate fluid. CRRT is useful for 24-hour-per-day clearance of nitrogenous wastes, metabolic by-products, and excess fluids. CRRT is often preferable to HD in the critical care setting because it reduces the risks for fluid and electrolyte disorders and hypotension while allowing for more liberal fluid and nutrient provisions. In patients with severe fluid intolerance, slow continuous ultrafiltration may be necessary. Protein losses can be as high as 10 to 13 g per HD session versus 5 to 10 g per day in CRRT [23,24]. Amino acids can be added to the hemodialysate solution to promote retention of nitrogen balance [25]. Consideration should be given to typical glucose content of the dialysate, as this may make a significant contribution to the caloric load of patients already exhibiting some form of glucose intolerance. Dialysate of CRRT is approximately 1.5% glucose, thereby contributing up to 600 glucose calories during a 10-L per day dialysis infusion [26].
Enteral and Parenteral Formulations
A wide array of enteral nutrition products has been designed for patients in varying stages of renal disease (Table 192.2). Formula selection depends largely on the individual’s fluid allowance [27]. For predialysis ARF patients in need of short-term enteral nutrition, a formula containing only essential amino acids and histidine with little or no vitamins, minerals, and electrolytes may be appropriate. Products with reduced levels of protein, phosphorus, potassium, magnesium, and vitamin A are useful for patients with chronic renal insufficiency, yet not on dialysis. Moderate protein formulas with low electrolyte content are often indicated for patients receiving intermittent dialysis treatments. All enteral products designed for use in renal dysfunction are concentrated in volume (2 kcal per mL) to aid in fluid management. It is best to initiate tube feedings at a slow rate in this population and advance the feeding rate gradually to prevent osmotic diarrhea. Because patients on CRRT demonstrate improved clearance of nitrogenous wastes, fluid, and electrolytes, standard enteral formulas may be used. In this case, selection likely depends more on accompanying clinical conditions than on renal status. For example, a low-carbohydrate formula may be more appropriate for the CRRT patient with glucose intolerance than the typical calorically dense renal formulas.
Table 192.2 Specialty Enteral Products for Use in Renal Failure | ||||||||||||||||||||||||||||||||||||||||||||||||||||||||||||||||||||||
---|---|---|---|---|---|---|---|---|---|---|---|---|---|---|---|---|---|---|---|---|---|---|---|---|---|---|---|---|---|---|---|---|---|---|---|---|---|---|---|---|---|---|---|---|---|---|---|---|---|---|---|---|---|---|---|---|---|---|---|---|---|---|---|---|---|---|---|---|---|---|
|
Delayed gastric emptying related to dialysis treatment, diabetes, high blood urea nitrogen levels, hyperglycemia, or postoperative gastrointestinal complications can lead to enteral feeding intolerance in patients with renal failure. PN is indicated when the enteral route cannot safely be used to fully meet daily nutritional requirements. In general, standard amino acid solutions can be used. When fluid volume restriction is necessary, concentrated 15% amino acids solutions are helpful. A parenteral amino acid solution of equal amounts of essential amino acids and standard amino acids at a dose of 1 g per kg
per day may be given in cases of severe intolerance to standard mixtures despite intensive dialysis. Patients receiving enteral or parenteral preparations using essential amino acids as the sole source of nitrogen have demonstrated conflicting results concerning improvement in nitrogen balance and overall recovery of renal function [14,28,29]. Also, nonessential amino acids may become conditionally essential for protein synthesis and ammonia detoxification when the patient is under the stress of certain disease states. Thus, the use of formulations containing only essential amino acids should be reserved for less than 2 weeks of treatment in quantities less than 0.5 g per kg per day for patients with worsening renal function who are unable to begin dialysis.
per day may be given in cases of severe intolerance to standard mixtures despite intensive dialysis. Patients receiving enteral or parenteral preparations using essential amino acids as the sole source of nitrogen have demonstrated conflicting results concerning improvement in nitrogen balance and overall recovery of renal function [14,28,29]. Also, nonessential amino acids may become conditionally essential for protein synthesis and ammonia detoxification when the patient is under the stress of certain disease states. Thus, the use of formulations containing only essential amino acids should be reserved for less than 2 weeks of treatment in quantities less than 0.5 g per kg per day for patients with worsening renal function who are unable to begin dialysis.
Intradialytic parenteral nutrition (IDPN) is another means of nutritional support designed for use in malnourished HD patients unable to meet full protein and energy requirements by the oral or enteral route. IDPN may offer some advantages over PN in that dedicated vascular access is not needed and administration is done during dialysis therapy to avoid fluid overload. On the other hand, IDPN alone cannot provide adequate daily nutrition; it places the patient at high risk for hyperglycemia with insulin resistance, and expenses of the treatment are comparable to PN [30]. Several trials have attempted to demonstrate the efficacy of IDPN with favorable results; however, limitations in study design have left health care professionals wary of supporting its use in clinical practice [31]. A recent prospective study of 16 malnourished HD patients receiving IDPN revealed a significant weight gain after 6 months of IDPN treatment [32]. No control group was used in this study and no other outcome variables (i.e., morbidity, mortality) were adequately evaluated. At present IDPN should not be used as a substitute for total PN, especially in the critical care setting.
Summary of Nutritional Recommendations
Primary efforts of the caregiver should be directed toward management of the various nutritional and metabolic disorders commonly associated with renal failure. Adequate nutrient provision may optimize renal function, improve nutritional status, and raise the chances of survival in ARF patients [33]. Protein and energy requirements are largely dependent on the underlying causes of renal failure in the critically ill patient. ARF secondary to sepsis or severe injuries places a far greater nutrient demand on patients than that of nephrotoxic drug-induced ARF. The Harris–Benedict equation is used to calculate basal energy requirements, which is then multiplied by an activity and stress factor to determine total energy expenditure. Estimates of total energy expenditure in a critically ill population are typically between 30 and 45 kcal per kg per day. Increasing energy above this does not improve nitrogen balance [34]. Patients with a prolonged stay in the ICU may benefit from the more accurate predictions of energy expenditure afforded by indirect calorimetry [35]. Nondialyzed patients with ARF require a protein restriction of less than 0.5 g per kg of essential amino acids or 0.6 to 1.0 g per kg per day of mixed protein sources. However, such severe restrictions should not be imposed for longer than 2 weeks, and importance should be given to adequate energy provision for protein sparing. Patients receiving intermittent HD require 1.2–1.5 g per kg per day of mixed protein sources, whereas those undergoing CRRT can tolerate protein levels of up to 2.5 g per kg per day. Serum electrolytes should be monitored daily with additives adjusted on an individual basis. Standard vitamins and trace minerals can safely be provided to renal failure patients in the ICU. Fluid allowances for nondialyzed or HD patients are based on 24-hour urine output with an additional 500 mL for insensible losses. Those undergoing CRRT should be permitted additional fluid for provision of full nutritional support. A summary of recommendations supported by randomized controlled trials is included in Table 192.3.
Table 192.3 Nutrition Support in Acute Kidney Injury | |
---|---|
|
Liver Failure
As the central regulatory organ of the body, the liver is responsible for the metabolism, storage, activation, transport, and synthesis of many vital nutrients. Biochemical reactions fundamental to carbohydrate metabolism such as glycogenesis and gluconeogenesis are carried out in the liver. Albumin, transferrin, prealbumin, and prothrombin are a few of the major serum proteins generated in the liver. Fatty acid oxidation as well as the production of bile salts, triglycerides, and cholesterol for lipid absorption and transport is part of the normal hepatic function. The liver is also responsible for the catabolism of various potentially toxic substances including ammonia, alcohol, and acetaminophen. Liver damage can lead to the disruption of many of these processes; however, due to the large capacity for hepatic reserve, dysfunction is not usually seen until 80% to 90% of the liver cells have been injured [36].
A number of insults can initiate the cellular degeneration of acute or chronic liver disease. Viral infection, alcohol use, medications or other hepatotoxic agents, cardiac shock, chronic cholestasis, metabolic disorders, and autoimmune diseases are all potential instigators of liver injury. The damage can be so sudden and severe that it results in fulminant hepatic failure (FHF), a rare disease involving extensive liver necrosis and often culminating in death. Complications of FHF include metabolic abnormalities such as hypoglycemia or acidosis, hemodynamic instability, cerebral edema, sepsis or immunosuppression, and the hepatorenal syndrome. The presence of hepatic encephalopathy (HE), manifested by several neurologic, behavioral, and neuromuscular changes, may be able to predict the prognosis of FHF depending on the severity of the impairment [37]. Treatment of FHF often involves nutritional intervention; however, no controlled studies have been done to assess the benefits of nutrition therapy in this population.
Patients with acute hepatitis tend to be highly catabolic in the setting of severe gastrointestinal distress. Nausea, vomiting, and anorexia with occasionally concurrent acute pancreatitis may preclude the ability for oral intake. Short-term nutrition support is often necessary until causes of the acute injury to the liver have been identified and treated.
The end stage of most chronic liver diseases is the development of cirrhosis. Cirrhosis is characterized by repeated episodes of necrosis, followed by regrowth and formation of connective scar tissue. The resulting disruption of normal hepatic structure increases resistance of blood flow to the liver. Portal hypertension, esophageal varices with gastrointestinal bleeding, and ascites often stem from altered hepatic circulation in cirrhotic patients. Clinical evidence of cirrhosis can progress from elevated serum transaminases and jaundice to
hypoalbuminemia and HE. Malnutrition has been documented in up to 100% of hospitalized patients with alcoholic liver cirrhosis [38]. It is important to note that the presence of esophageal varices or ascites does not preclude the use of small bowel nasoenteric tube feeding in malnourished cirrhotic patients [39]. Several controlled trials using enteral nutrition in this population have demonstrated improvements in liver function tests, nutritional status, nitrogen balance, length of hospitalization, and overall prognosis [40,41,42]. The achievement of positive nitrogen balance did not have a negative impact on encephalopathy, azotemia, edema, or ascites among the study groups.
hypoalbuminemia and HE. Malnutrition has been documented in up to 100% of hospitalized patients with alcoholic liver cirrhosis [38]. It is important to note that the presence of esophageal varices or ascites does not preclude the use of small bowel nasoenteric tube feeding in malnourished cirrhotic patients [39]. Several controlled trials using enteral nutrition in this population have demonstrated improvements in liver function tests, nutritional status, nitrogen balance, length of hospitalization, and overall prognosis [40,41,42]. The achievement of positive nitrogen balance did not have a negative impact on encephalopathy, azotemia, edema, or ascites among the study groups.
Malnutrition and Metabolic Alterations
Malnutrition in acute and chronic liver disease is the result of a combination of factors. A decrease in oral intake is common in the patient with prolonged gastrointestinal distress, early satiety secondary to ascites, or excessive alcohol consumption. Maldigestion and malabsorption leading to steatorrhea is often seen with cholestasis or chronic pancreatitis. Malnutrition in liver failure is also closely linked to the presence of severe metabolic derangements characteristic of hypercatabolic states of organ injury. Impaired glycogen synthesis and storage as well as decreased hepatic degradation of stress hormones lead to the preferential use of lipid and protein reserves for gluconeogenesis [43]. Insulin resistance and glucose intolerance are usual complications of early liver failure. Hypoglycemia can occur in decompensated cirrhosis or FHF as a result of hepatic glycogen depletion and impaired gluconeogenesis.
Hepatic steatosis with concurrent depletion of adipose tissue stores is a frequent consequence of the imbalance between lipid uptake, fatty acid oxidation, and the release of lipoproteins by the damaged liver. It is important to note that hepatic steatosis is often preventable by avoiding overfeeding. Lipids are the primary source of energy in enteral nutrition supplements designed for use in liver failure patients. IV lipids are also metabolized well by critically ill patients with hepatic failure when given in amounts not to exceed the energy needs of the individual patient. A recent study by Druml et al. found no significant difference in uptake, hydrolysis, or oxidation of a 20% IV lipid emulsion in septic patients with hepatic failure versus in healthy controls [44].
Altered protein metabolism is by far the most challenging aspect of providing nutrition therapy to the critically ill patient with liver disease. Cirrhosis has long been established as a catabolic disease, with unremitting protein degradation and inadequate resynthesis leading to depletion of visceral protein stores and muscle wasting [45]. Under ordinary circumstances, the skeletal muscle collects circulating branched-chain amino acids (BCAAs) for the synthesis of glutamine and alanine, amino acids that are released into the bloodstream and taken up by the liver for use in hepatic gluconeogenesis. Glutamine is also a carrier for ammonia, a potentially toxic by-product of protein metabolism. Ammonia is normally converted into urea by the liver and excreted by the kidneys. As liver function declines, uptake of serum glutamine is diminished and the degradation of ammonia into urea is impaired. In this case, excess serum glutamine and ammonia is diverted to renal pathways for direct excretion by the kidneys.
Adequate protein intake is therefore essential in the liver patient not only for the provision of energy by gluconeogenesis but also for the preservation of skeletal muscle mass and the prevention of HE. The clinical practice of protein restriction in patients with liver damage is common, for fear of precipitating or worsening central nervous system changes associated with HE. Several protein-related theories have been proposed regarding the development of HE, although it is of significance that the occurrence of encephalopathy has not been observed to directly correlate with protein intake in cirrhotic patients [46]. IV protein solutions with higher concentrations of BCAAs have been developed for use in liver disease based on the following hypothesis. As the use of BCAAs by skeletal muscle increases, serum levels decrease, thereby leaving an imbalance of BCAAs and aromatic amino acids at the blood–brain barrier. With less opposition from BCAA, aromatic amino acids readily cross into the central nervous system to form “false neurotransmitters.” The false neurotransmitters compete with actual neurotransmitters for binding sites and disrupt normal central nervous system function to cause symptoms of HE [47].

Full access? Get Clinical Tree
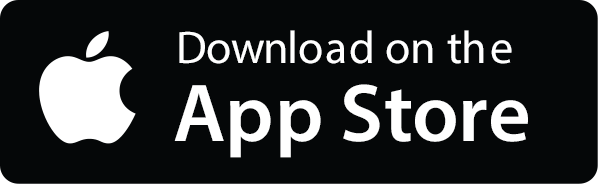
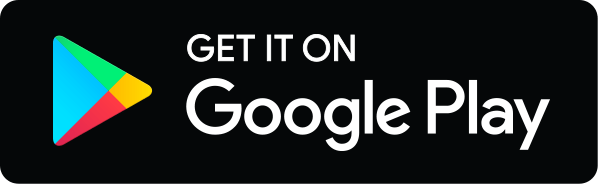