Diabetes Mellitus
Carol Green-Hernandez PhD, FNP-C
Pavel Fort MD
INTRODUCTION
Diabetes in childhood is a devastating diagnosis for the patient and family, and carries the potential for an altered quality of life and a decreased life span. However, such outcomes need not always occur. This chapter provides information about the diagnosis and management of several variants of diabetes, including type 1 and nonautoimmune variants, type 2 of adolescence, and maturity-onset diabetes of the young (MODY, or type 3). Strategies are emphasized for developing and maintaining a successful partnership with the patient and family in all aspects of primary care. The essentials for successful comanagement of the patient with a pediatric endocrinology team specializing in diabetes are highlighted.
ANATOMY, PHYSIOLOGY, AND PATHOLOGY
Type 1 diabetes mellitus has several variants, including autoimmune type 1 diabetes, nonautoimmune diabetes of infancy, and idiopathic forms of the disease. These each are physiologically different from type 2 diabetes of adolescence, and each of these in turn differs from the autosomal dominant MODY (type 3).
Type 1 Diabetes Mellitus
Autoimmune Type 1 Diabetes Mellitus
Type 1 diabetes is highly correlated with absence of insulin secretory reserve, secondary to beta cell destruction. Type 1 disease is an autoimmune process that is associated with genetic polymorphism in the HLA class II region and also involves component regions of classes I and III (Chervonsky et al., 1997; Ma et al., 1997; Dorman, 1997; Lan et al., 1998; Juneja, 1999). Type 1 diabetes is characterized by destruction of beta cells by glutamic acid decarboxylase antibodies and is therefore classified as immune-mediated diabetes. Autoimmune type 1 diabetes eventually leads to significant infiltration of islet cells by T cells, leading to their scarring and, ultimately, destruction of functioning beta cells for months to years.
Other factors are interwoven with the autoimmune component of type 1 pathogenesis. These factors include the child’s genetic background, the perinatal experience, birth weight (both high and low) (Minior et al., 1998), and early exposure to environmental triggers for disease (Klemetti et al., 1998). In addition, the genes and alleles for type 1 appear to differ from population to population (Akerblom, 1998; Dorman, 1997).
The thymus appears to play a dual role: Insulin-like growth factor II (IGF-II) is involved in T-cell development and T-cell negative selection. It is conceivable that defective thymic censorship of the antigen-antibody to insulin may be an important factor in the pathogenesis of type 1 diabetes (Geenen et al., 1998; Like et al., 1982).
The role of environmental triggers in type 1 development is similarly confusing. It is not yet clear whether only one significant exposure to a trigger is enough for ensuing pathogenesis or whether several exposures to a variety of triggers are required (Greenbaum et al., 1999). The question begs to be asked: Do different populations require different triggers, depending on genetic predeterminants? What is clear is that type 1 diabetes is multigenic, its pathogenesis being an outcome of environmental triggers interfacing with a genetically susceptible host.
Clearly, environmental chemical toxins can play an important role in beta cell destruction. Nitrosourea compounds, rodenticides, and streptozocin have all been demonstrated to cause immune-mediated type 1 diabetes (Kaufman, 1997). Fetal exposure to nitrites and nitrates in food and water also have been hypothesized as causative agents of beta-cell destruction (Dorman, 1997).
In recent years, one oft-debated environmental trigger is nutrition. Both cow’s milk and meat proteins have been implicated. Several studies have examined the role of cow’s milk exposure and type 1 pathogenesis in young children (Dorman, 1997; Saukkonen et al., 1998; Dahlquist, 1998). Saukkonen and colleagues found that Finnish children who express type 1 disease have higher levels of cow’s milk antibodies and decreased IgM antibodies to cow’s milk formula than sibling controls matched for HLA-DQB1 genotype. The researchers’ findings suggest that genetically susceptible children who are exposed to cow’s milk itself in their first year of life are at greater risk for later type 1 diabetes.
Population-based studies in diverse cultures and ethnic groups suggest that an environmental trigger may be operant in the growing worldwide incidence of type 1 diabetes (Diabetes Epidemiology Research International Group, 1988, 1990; Green et al., 1992; Karvonen et al., 1993; Dahlquist & Mustonen, 1994; Dorman et al. 1996; Perez-Bravo et al., 1996; Dahlquist, 1998). For example, there is growing speculation that the increasing incidence of type 1 disease among Japanese children may involve an increased propensity for including meat products in the diet. This dietary change is a marked one, compared with the traditional eating habits of previous generations (Dahlquist, 1998). Nevertheless, more work needs to be done in different population groups before a more definitive statement regarding type 1 triggers and disease incidence can be made.
Nonautoimmune Diabetes of Infancy
Because nonautoimmune diabetes of infancy does not differ from type 1 in clinical presentation, the infant presenting in a ketoacidotic state may be misdiagnosed as having type 1 disease. In fact, they are two different and distinct entities. Nonautoimmune diabetes of infancy does not include destruction of beta cells among its sequelae.
Several forms of nonautoimmune diabetes of infancy have been described, including diabetes secondary to congenital agenesis of the pancreas and diabetes occurring as part of the autosomal recessive syndrome of polycystic kidney disease (Zerres et al., 1994). The most frequently seen variant is transient neonatal diabetes mellitus (TNDM). TNDM is still a rare occurrence. The pathogenesis of TNDM is not known, although it may be a logical result of immature beta cell function. This is the reason it is termed transient. In transient disease, the infant will mature out of the temporarily diabetic state (Attia et al., 1998).
Transient neonatal diabetes mellitus has been described as developing spontaneously in normal and in low birth weight infants. TNDM has also been observed to occur in infants from the same nuclear family (Attia et al., 1998; Karl & Heiner, 1995). Common features in TNDM presentation include very young age (typically between 1 and 2 months) and diabetic ketoacidosis. Obviously, the latter occurrence may lead the provider to assign a preliminary diagnosis of type 1 diabetes to the child. Unlike in true type 1 disease, insulin therapy usually can be discontinued after a couple of months, although Karl and Heiner reported a case that required insulin therapy for 18 months. Insulin treatment is no longer necessary when the infant’s innate beta cell function has matured enough to take on the task of endogenous insulin production. TNDM generally has no further consequences once endogenous beta cell function ensues. However, diabetes recurrence in previously stabilized children has been reported (Attia et al., 1998; Karl & Heiner, 1995).

On the off-chance that the diabetes recurs later in childhood, infants treated for TNDM should continue to be monitored for diabetes.
• Clinical Pearl
Adequate diabetes prevention strategies should be taught to these children and their families for lifelong prevention and delay of later diabetes (Hahl et al., 1998; Knip, 1998).
Idiopathic Type 1 Diabetes Mellitus
Like immune-mediated type 1 disease, idiopathic diabetes is characterized by absence of insulin secretory reserve. As in TNDM and autoimmune type 1 diabetes, patients with idiopathic type 1 diabetes are subject to ketoacidosis (American Diabetes Association [ADA], 1997b). Permanent insulinopenia that is not associated with an autoimmune disease process is rare (Kaufman, 1997).
Diabetes that results from cystic fibrosis (CF) exemplifies nonimmune-mediated type 1 disease, yet it also shares features with type 2 diabetes. Ketoacidosis is a rare event in diabetes of CF, although it may be present at the time of diagnosis in cases in which prolonged hyperglycemia was unrecognized (Moran et al., 1998).
Type 2 Diabetes Mellitus
Traditional models of diabetes classification place type 2 disease firmly in adulthood. This is no longer true; diagnosis of adolescents and occasionally younger children with type 2 disease is becoming increasingly commonplace. Type 2 diabetes is a polygenic disease that occurs as an outcome of long-term insulin excess and concomitant insulin resistance. Sometimes this scenario ends with insulin deficiency, because compensatory hyperinsulinemia cannot override insulin resistance. At levels of 140 mg/dL or more, glucose is toxic to beta cells and to target tissues where insulin works. Unlike type 1 disease, the insulin deficiency of type 2 disease is not absolute. Exogenous insulin administration cannot override the body’s resistance to endogenous insulin at this point in type 2 pathogenesis.
Type 2 disease of adolescence is more common in obese children of color than in their Caucasian peers who are overweight. It is also seen in children of mothers who had gestational diabetes, regardless of race or ethnicity (ADA, 2000a). Obesity plays a central role in the pathogenesis of type 2 diabetes in part because of the metabolic derangements, including excessive insulin secretion, that can accompany obesity (Kaufman, 1997; Scott et al., 1997; Glaser & Jones, 1998; Hanson et al., 1998; Slyper, 1998). The extent of hyperinsulinism and insulin resistance that occur secondary to obesity seems to vary from one ethnic group to another.
Otherwise healthy adolescents with no first-degree family history of diabetes were studied by Arslanian and Suprasongsin (1996). They found that African American adolescents exhibited increased insulin production and decreased insulin sensitivity compared with young Caucasian subjects. Their findings correspond with similar population-based studies of North American adolescents of African, Pima, Ojibwa-Cree, Hispanic, and Japanese heritage (Pettitt et al., 1993; Islam et al., 1995; Haffner et al., 1996; Glaser & Jones, 1998; Dean et al., 1998; Libman et al., 1998; Boyle et al., 1999), as well as Central American youth in Veracruz, Mexico (Aude Rueda et al., 1998).
• Clinical Pearl
The outcome of delayed glycolysis and glycogenolysis in infants of very low birth weight (ie, lacking in subcutaneous tissue) means that they face increased risk of insulin resistance and hence type 2 disease in adulthood. The provider needs to be aware of this risk in the patient whose birth weight was very low so as to apprise parents of health-promoting activities that may help their child to delay or avoid type 2 diabetes.
Maturity-Onset Diabetes of the Young
Sometimes referred to as type 3 diabetes, MODY is an autosomal dominant inherited disorder often mistakenly diagnosed as either type 1 or 2 disease. MODY is actually neither, although it is more correctly type 2 than type 1. MODY is a heterogenic disorder of impaired insulin secretion without its absolute absence, characterized by some degree of the insulin resistance syndrome seen in type 2 disease. Mutations of four different genes have been identified in the pathogenesis of MODY (Hattersley, 1998). Further, patients are not predictably from those ethnic groups with a high incidence of type 2 diabetes. Rather, patients with MODY typically are Caucasian, although the subtype for MODY 5 has been well demonstrated in Japan. Hattersley states that there may be a sixth subtype as well (MODY 6).
• Clinical Pearl
More subphenotypic variants for MODY will certainly be discovered in coming years. This means that treatment methods will need to be individualized in keeping with each patient’s genetic permutation.
Regardless of phenotype, patients with MODY have strong family histories for type 2 disease and sometimes for type 1 disease or even for MODY. They do not demonstrate morphologic evidence of the glutamic decarboxylase antibody of type 1 diabetes. Other characteristics, at least of MODY 1, 2, and 3, include onset before age 25 years and absence of ketosis (Cameron & Batch, 1997). Cortisol,
glucagon, and growth hormone levels tend to be normal, as are serum lipids.
glucagon, and growth hormone levels tend to be normal, as are serum lipids.
There are currently four well-described subphenotypic classifications of MODY (MODY 1, 2, 3, and 4) (Stoffers et al., 1997; Green-Hernandez, 1999a). At this point, MODY 1 and 3 appear to have a central deficiency in insulin secretion. Insulin levels may range from normal or slightly decreased to altogether absent after glucose challenge. Conversely, when faced with a glucose load, the patient with MODY 2 demonstrates a diminished response to signaling for beta cell secretion of insulin, resulting in normal to mild decreases in insulin levels, although hyperglycemia becomes more severe in later life. Microvascular complications are rare in MODY 2. It more typically occurs in early childhood, perhaps even from birth. MODY 2 can thus be confused with early type 1 disease (Cameron & Batch, 1997; Hattersley, 1998).
MODY 3 is more likely to occur in adolescence or early adulthood. Disease presentation is one of mild to moderate hyperglycemia, with the potential to become progressively more severe as the young person grows older. Microvascular complications are an expected outcome if normoglycemia is not achieved in disease management.
MODY 4 appears to occur later than 1, 2, or 3. Stoffers and colleagues (1997) state that in their experience, the average age of onset of MODY 4 is 35 years. Hyperglycemia is variable, depending on patient participation in the diabetic regimen. Thus, microvascular complications are also variable, depending on degree and consistency of patient normoglycemia. Like the other variants, MODY 4 is nonketotic and occurs in families with high diabetes prevalence rates.
Children and adolescents who develop MODY (whether 1, 2, or 3) are generally asymptomatic until after several weeks to months of glucose elevation. Serum glucose can range from impaired glucose tolerance (IGT) to frank fasting hyperglycemia. These youngsters are generally not obese. Just as the incidence of obesity is increasing in the general pediatric population (Slyper, 1998), however, so too is it in the MODY group (Cameron & Batch, 1997; Tattersal, 1982). Unlike in type 1 and type 2 diabetes, environmental factors such as obesity appear to play little role in the pathogenesis of MODY (Hattersley, 1998).

Consider MODY when a nonobese adolescent presents with elevated blood glucose but without ketosis and has a family history of type 2 diabetes.
Gestational Diabetes Mellitus
Gestational diabetes mellitus (GDM) can occur in the young pregnant adolescent with or without a prior history of obesity. GDM is expressed in response to carbohydrate intolerance in 1% to 5% of pregnant women (Spong et al., 1998). Infants of these pregnancies tend to have higher birth weights and higher incidences of macrosomia, thus placing them at greater risk for subsequent diabetes development. Hispanic women are at increased risk for GDM. The earlier its occurrence in a pregnancy, the greater the risk for GDM in subsequent pregnancies. This is especially true for women who need insulin and who have elevated third trimester glucose values.
Gestational diabetes is not a focus for this text. The reader whose young patient is pregnant and is diagnosed with GDM is directed to examine other resources for the diagnosis and management of GDM, including the works of Coustan & Carpenter (1998) and Langer (1998). The ADA position statement on gestational diabetes outlines current management guidelines for preconception and prenatal care (2000a).
Pathology of Complications of Diabetes
Ketoacidosis
Many children first present with diabetes as a diagnostic outcome of emergency treatment for ketoacidosis. This holds true for both type 1 and type 2 disease. Ketoacidosis is rare in MODY, though it can occur in MODY 2. Insulin deficit underscores ketoacidosis, whether this deficit is relative (as in type 2 and insulin resistance syndrome) or absolute (type 1). Insulin inhibits gluconeogenesis and glycogenolysis. It is needed for ATP synthesis and protein synthesis. Lipogenesis requires insulin bioavailability; its absence leads inexorably to lipolysis. In short, without insulin, glucose could not be taken up by peripheral tissues. Insulin deficiency leads to hyperglycemia. The final outcomes of insulin absence include depletion of hepatic stores of glycogen, muscle breakdown, and release of free fatty acids.
Ketoacidosis results when continued glycogenolysis produces ketone bodies. Dysregulation of the endocrine system’s regulatory hormones ensues, including increased cortisol, catecholamine, and growth hormone production. These hormones exacerbate the hyperglycemia. Persistent, profound hyperglycemia leads to osmotic diuresis. Nausea and vomiting may accompany this hormonal dysregulation. As the hyperglycemia deepens, consciousness shifts toward increasing confusion. In a worst-case scenario, profound dehydration and coma occur and, if left untreated or treated too late, lead to brain damage or death (Silink, 1998). Refer to Chapter 47 for an in-depth discussion of the diagnosis and management of ketoacidosis.
Chronic Complications of Diabetes Mellitus
All complications, including nephropathy, neuropathy, and retinopathy, can be prevented or significantly delayed for decades if diabetes is well controlled (Diabetes Control and Complications Trial [DCCT] Research Group, 1993; 1995; Lekakis et al., 1997; Author, 1998; Cooper, 1998; Lovestam-Adrian et al., 1998; Shaw et al., 1998; Aielo, Gardner, King, et al., 1998). Total costs associated with treating the complications of diabetes are astronomical. Direct medical costs in the United States in 1992 alone totaled $44.1 billion. Indirect costs, including work loss, disability, and premature death, totaled $54.1 billion (ADA, 1997a). Because these complications typically occur years and even decades after the diagnosis of diabetes, it may be difficult to explain why the patient should want to participate in a prescribed regimen that is not noted for being much fun.
Vascular changes, including renal and large vessel disease, occur only after many years of poorly controlled diabetes. The average child with diabetes will not demonstrate such changes during early or middle childhood. However, by adolescence, signs of renal complications especially may become apparent in the patient with poorly controlled diabetes. Similarly, retinopathy may begin to be seen in adolescence if chronic hyperglycemia has been a part of the child’s management pattern.
Histopathologic changes lead to thickening of glomerular basement membrane. This change is seen in all patients with diabetes mellitus and can lead to persistent microalbuminuria. Left unchecked, microalbuminuria will eventually evolve into albuminuria and nephropathy. In keeping with this phenomenon, nearly all individuals with type 1 diabetes eventually develop nonproliferative retinopathy. Of these, approximately 30% of patients go on to develop vision-threatening retinal diseases (VTRDs), including neovascularization
and maculopathies, as outcomes of their disease (Gilbert et al., 1998). These findings are increasingly being seen in members of the adolescent diabetic population who, in an effort to be model-slim, are not as active in coparticipating in their insulin regimen.
and maculopathies, as outcomes of their disease (Gilbert et al., 1998). These findings are increasingly being seen in members of the adolescent diabetic population who, in an effort to be model-slim, are not as active in coparticipating in their insulin regimen.
The recent work of Gilbert et al. (1998) points toward a connection between evolving nephropathy and increased risk for VTRDs in patients with type 1 disease. The actual timing of stages at which nephropathy and proliferative retinopathy coexist is not yet clear (Lovestam-Adrian et al., 1998). It is reasonable to believe that patients with persistent hyperglycemia who go on to develop nephropathy and VTRDs have a genetic predisposition to do so. Further, the fact that some patients in poor glycemic control will present with VTRDs but not nephropathic findings points to the multifactorial influences of differing genetic and environmental influences in the pathologic consequences of poorly controlled, long-term disease.
EPIDEMIOLOGY
Type 1 diabetes is found in every country and among most ethnic groups. In the United States alone, 123,000 children have type 1 disease (National Diabetes Data Group, 1995). Of these, about 75% are younger than 18 years at the time of diagnosis (ADA, 2000c). Among adolescents, type 1 prevalence is approximately 1 in 500 (Sperling, 1994; Draznin & Patel, 1998). Whether type 1 or 2, diabetes is on the increase in children worldwide. A change in genetics is not operant, because risk genes are not increasing in children born today, compared with previous generations.
Clearly, lifestyle variables have changed in the past generation and, coupled with other environmental factors, seem to be at the core of the diabetes epidemic. The rushed lifestyle of the adult world is no less obvious than that of children. Hurried lives mean more fast food consumption, with increased simple sugar and fat calories. Many empty calories are consumed in soda and other sugared drinks, including juices, sports drinks, and bottled tea and coffee beverages. Adolescents consume these and other covert sugar in their coffee bar beverages, including lattes and other espresso drinks. Children today are also less active than those of earlier generations, with television and computer games replacing bike-riding and pick-up sports activities for many. Snack food consumption and passive activities are well linked in the literature (Strong et al., 1999). Whether or not the child is at genetic risk, these factors are the antecedents to obesity and, ultimately, type 2 diabetes. The youngest recorded child diagnosed with type 2 diabetes was an obese 5 year-old Pima Indian (Hanson et al., 1998).
For children at risk for type 1 disease, the foregoing factors act as triggers to its genetics, at earlier ages and in a wider population and ethnic base than in earlier generations. First-degree relatives of Caucasian patients share a genetic risk for diabetes development that exceeds that of the general population (6% versus 1%), although more than 20% of children with type 1 diabetes exhibit a positive family history (Dorman, 1997; Altobelli et al., 1998).
As population-based epidemiologic studies increase, more will be learned about variations in the epidemiology of type 1 diabetes in different ethnic groups. It is reasonable to say that type 1 diabetes occurs in different genetically determined individuals as an outcome of different combinations of both genetic and nongenetic risk factors. These combine differently from one child to the next in producing type 1 disease. Beta cell destruction is accelerated by insulin demand as an outcome of different environmental triggers in a genetically susceptible individual. These triggers include the perinatal environment, living in a cold region, infections experienced, nutritional influences (perinatal and in infancy), and a rapid growth rate during pubescence.
Perinatal triggers may include maternal ingestion of nitrites during pregnancy, such as those found in preserved meats and in some drinking water sources. Maternal smoking can be considered a significant environmental trigger in genetically susceptible fetuses (Dahlquist, 1998). Antepartal stress, as well as later psychological stress, has been implicated in triggering of disease (Thernlund et al., 1995). Neonatal birth weight has also been linked to disease onset, with large-for-gestational age babies being at greater risk for type 1 diabetes, and premature and very low birth weight infants facing a risk for type 2 in adulthood (Dahlquist, 1998; Thompson et al., 1997).
Incidence of type 1 disease is greatest in children of northern European heritage, with Finnish people having the world’s highest incidence and Japanese the lowest. Seasonal variations have been identified, with more cases presenting in winter than in warm-weather months (Dahlquist, 1998). Infectious exposure antenatum as well as during the clinical year preceding diabetes onset is also a feasible trigger of disease. Rubella was at one time a significant antecedent to type 1 onset, but it is now rare in westernized countries. Coxsackie B virus and other enteroviral exposures have been found to be associated with triggering of type 1 diabetes (Dahlquist, 1998).
Pubescence is a time of significant growth, including increased growth hormone release with concomitant impact on serum cortisol levels and insulin secretion and tissue uptake. Several epidemiologic studies conducted in different regions of the world all demonstrate a peak in the incidence of type 1 diabetes during this period. Rapid growth, with sudden and significant growth rate, including being taller than their peers, has been identified in the later pathogenesis of type 1 in genetically susceptible youth (Blom et al., 1992; Dahlquist, 1998; Tolis et al., 1997).
HISTORY AND PHYSICAL EXAMINATION
Questions specific to screening for diabetes can be found in Display 61-1. Information related to the physical examination is presented in Display 61-2.
DISPLAY 61–1 • History Questions in Screening for Diabetes
Do you have a family history of diabetes?
If yes, then is it type 1 or type 2, and in whom?
Do you have classic symptoms (ie, polydipsia, polyphagia, unexplained weight loss)?
Are you a member of an ethnic group having a high incidence of diabetes (Native North American, African American, Asian, Hispanic)?
What was your birth weight and, if known, approximate gestational age?
In female of childbearing age: do you have a history of gestational diabetes; delivering an infant over 9 lb; or toxemia, stillbirth, polyhydramnios, or other complications associated with a pregnancy?
Do you have lifestyle stress?
Have you had a recent stressful event (positive or negative)?
What is your weight history (loss or gain)?
Do you have a history of diabetes treatment? If yes, diet prescription, medications, self-management training, glucose self-monitoring?
Do you have a history of complications, including ketoacidosis and hypoglycemia?
List your diet (including fluids) history for food types, amounts, and how prepared in a typical day. Include condiments and all snacks consumed.
List aerobic and anaerobic exercise, noting type, amount of time, and frequency.
What is your sleep–rest history (insomnia, nightmares)?
Do you have visual changes (blurring, diplopia, floaters)?
Do you have numbness, tingling, or burning in the hands or feet? This is a bilateral rather than unilateral neuropathy in diabetes and, although primarily sensory, motor nerve fibers may be involved. In more extreme cases polyneuropathy may progress from severe pain to anaesthesia, and:
Are motor manifestations, including muscle weakness progressing to atrophy, with poor hand grasp or footdrop evident?
Do you have unexplained indigestion or abdominal pain?
Do you have unexplained diarrhea?
Have you had infections, including urinary tract infections and candidiasis?
Have you had intertriginous infections/irritations (in skinfold areas of the obese; under the female breasts, crural areas in men)?
Have you had vaginitis?
Do you have a history of chronic disease, including hypertension, endocrine, or autoimmune disease?
Do you have atherosclerosis risks, including diet, obesity, smoking, dyslipidemia, family history?
What medications do you take, prescribed and over the counter?
List your recreational drug use, including tobacco and alcohol (amounts and frequency).
Do you have any other ethnic, psychosocial, socioeconomic, educational, or lifestyle considerations that could affect the provider and patient in their management of diabetes?
DISPLAY 61–2 • Physical Examination for the Patient With Diabetes
Length/height and weight, with comparison to norms
Sexual maturation staging (during prepubertal stage of development)
Blood pressure (may need to obtain orthostatic measurements as well) and comparison to age-related norms
Eyeground examination (preferably dilated, done by an ophthalmologist or licensed optometrist skilled in diabetes assessment) for signs of maculopathies and retinopathies:
Retinal hemorrhages
Scattered hard exudates
Cotton-wool exudates (generally in concert with severe hypertension)
Oral examination (eg, Candida)
Thyroid auscultatin and palpation
Cardiac examination (eg, S4)
Abdominal examination (eg, hepatomegaly)
Examination of pulses by palpation and auscultation
Hand and finger examination
Thorough skin examination (including insulin injection sites if applicable)
Neurologic examination, including assessing for a positive Romberg sign (sometimes presents as swaying, destabilized pattern; type 2, adolescent and older)
Sensory stimulation changes (peripheral neuropathy and, if indicated by history, abdominal; type 2, adolescent and older)
Vibratory sense changes (peripheral neuropathy and, if indicated by history, abdominal; type 2, adolescent and older)
Foot examination, looking for evidence of foot ulcers or infection not otherwise explained (type 2, adolescent and older)
Deep tendon reflex measurement (may be decreased, especially in presence of PN); absence of ankle reflexes early sign
Gait analysis (may be unstable, especially in presence of peripheral neuropathy)
If primary or differential diagnosis includes another endocrine disorder, such as pheochromocytoma, Cushing syndrome, acromegaly, or hemochromatosis or pancreatic disease, secondary diabetes possible in these patients (ADA, 2000b)
PN = peripheral neuropathy.
DIAGNOSTIC CRITERIA
A suspected diagnosis of diabetes mandates further investigation by the primary care provider. The clinician should consider a diagnosis of diabetes when a patient presents with any of the findings outlined in Display 61-3.
DISPLAY 61–3 • Diagnostic Criteria for Diabetes Mellitus
Fasting plasma glucose (FPG) should be measured after >8 hours of caloric fasting; elevation ≥126 mg/dL (7 mmol/L).
Patients in the higher FPG ranges usually present with the classic symptoms of polydipsia, polyuria, and unexplained weight loss.
Casual plasma glucose (ie, taken at any time of day, regardless of meal timing) is ≥200 mg/dL (11.1 mmol/L) plus symptoms.
Oral glucose tolerance test (OGTT) is not recommended for routine use. If these data are obtained, then a level ≥200 mg/dL at 2 hours should be investigated using an equivalent 75-g anhydrous glucose/water load.
Weight loss is common in type 1 but less so in type 2 disease, especially in people with preexisting obesity.
A patient who presents with FPG between 110 and 126 mg/dL can be classified as having impaired glucose tolerance (IGT). The OGTT should not be routinely used in the average clinical setting. If this value is obtained, its level at 2 hours continues to be classified as IGT if it is between 140 and 200 mg/dL.
FPG values:
*FPG < 110 mg/dL = normal
*FPG ≥ 110 and 126 mg/dL = IGT
*FPG ≥ 126 = conditional diagnosis of diabetes
The above should be reconfirmed on a different day through repeat testing in any patient presenting without symptoms of hyperglycemia accompanied by acute metabolic decompensation.
Sources: Expert Committee, 1997; ADA, 2000.
DIAGNOSTIC STUDIES
The following discussion relates to diagnostic testing for type 1 disease and for type 2 diabetes in adolescents. The reader is referred to current endocrine resources or current ADA guidelines for testing in suspected GDM.
The means for diagnosing diabetes have been modified from previous recommendations (ADA, 1997b, 2000c). These include preferential diagnosis through fasting plasma glucose
(FPG), although other blood test analyses can also be used.
(FPG), although other blood test analyses can also be used.
Testing is not recommended for type 1 disease in patients who are healthy and who do not carry autoimmune risk.
Testing should be considered more frequently in all patients, including those who are younger than 45 years and who:
Are obese (at or greater than 120% of desirable weight or with a body mass index at or greater than 27 kg/m2)
Report a first-degree relative who had diabetes
Are members of an ethnic group with high risk for diabetes (Native North American, or African, Asian, or Hispanic North American)
And in adolescents who:
Were diagnosed with GDM or delivered a baby over 9 lb
Have high blood pressure (at or greater than 140/90 mmHg) (Young et al., 1998; ADA, 2000c)
Have low-density lipoprotein cholesterol at or under 135 mg/dL or triglyceride at or exceeding 250 mg/dL
Were found previously to have either IGT or impaired fasting glucose (ADA, 1997b; 2000c)
The clinician should consider for testing any child with a history of:
FTT, especially in the absence of abuse or neglect
Delayed psychomotor development in which no other cause is operant
If information is needed about FTT or delayed psychomotor development, the reader should refer to Chapter 18 and Chapter 23.
MANAGEMENT
Whether type 1 or type 2, diabetes is characterized by consistent elevation of blood glucose; type 2 disease also involves insulin resistance. Clinical diagnosis, appropriate treatment options, and primary care management ideally lead to normalization of blood glucose and, if possible, improved glucose response to endogenous insulin in type 2 diabetes. The goal of treatment is not so much to control the disease but rather to prevent or decrease complications arising from the damaging effects of chronic hyperglycemia. The DCCT Research Group (1993) provided firm evidence that optimal control of blood glucose, defined as HbA1c of 7.4% or less, can delay or prevent long-term complications of diabetes. The ADA 1997 Report from the Expert Committee on the Diagnosis and Classification of Diabetes Mellitus goes a step further, setting an adult target fasting goal of 80 to 120 mg/dL, bedtime glucose level of 100 to 140 mg/dL, and glycosylated hemoglobin (HbA1c) of < 7.0%. Any level of improved control will decrease complication rates. Sound management plans arise from appropriate treatment options; more individualized plans of care can maximize positive outcomes for a patient. Various treatment options are appropriate in different clinical circumstances.
When symptoms of polydipsia, polyuria, and polyphagia occur, often accompanied by weight loss and, acutely, dehydration, the diagnosis of type 1 diabetes must be considered. Because the patient with this symptom array typically presents in ketoacidosis, management is emergent initially. Refer to Chapter 47 for information on the diagnosis and management of ketoacidosis. Once the ketoacidosis is reversed, then workup and management should follow ADA guidelines (2000c), with pediatric endocrinology consultation.
When symptoms of polydipsia, polyuria, and polyphagia present, current diagnostic guidelines support making a clinical diagnosis of type 2 based on a single blood glucose measurement. This is true even in the absence of weight loss, which is more typical in type 1 than in most variants of type 2 disease. The glucose test need not be fasting. Specifics for each kind of glucose measurement should correspond with the values presented in Display 61-4 (ADA, 1997b, 2000c; Limbach, 1998).
If diabetes is not confirmed but is still suspected, then the provider can obtain an oral glucose tolerance test (ADA, 2000c).
If diabetes is not confirmed but is still suspected, then the provider can obtain an oral glucose tolerance test (ADA, 2000c).
DISPLAY 61–4 • Laboratory Evaluation and Criteria for Diagnosing Diabetes
Fasting lipids (total cholesterol, high-density lipoprotein cholesterol, triglycerides, and low-density lipoprotein cholesterol)
Serum creatinine, if proteinuria is present
Urinalysis for glucose, ketones, protein, and sediment
Microalbuminuria (timed specimen, or the albumin to creatinine ratio) in pubertal and postpubertal patients with type 1 diabetes after 5 years of disease and in all patients with type 2 diabetes
Thyroid function tests in all patients with type 1 disease
Fasting plasma glucose (FPG) at 8 or more hours of ≥126 mg/dL, or
2-hour plasma glucose ≥200 mg/dL during a 75-g oral glucose tolerance test (OGTT), following World Health Organization guidelines; OGTT not recommended for routine clinical analysis
Random plasma glucose in an undiagnosed symptomatic child for diagnostic purposes
Unless patient is in metabolic decompensation, repeat the glycemic measure used on a different day to confirm the diagnosis.
Glycohemoglobin (GHB; HbA1c)
Sources: Report of the Expert Committee, 1997; ADA, 2000.
Whether type 1 or type 2 disease is present, the primary care provider must recognize the importance of ethnic and cultural influences when discussing treatment options. This recognition begins before planning interventions. Food customs and eating rituals differ from family to family. Specific ethnic or lifestyle habits greatly influence diet and meal planning. Similarly, cultural beliefs and values surrounding the body and perceived self-harm will influence the child’s and family’s levels of participation in self-monitoring and medical therapies. Presenting realistic diabetes treatment options mandates that the primary care provider inquire about and respect cultural influences, customs, beliefs, and values. This perspective is especially important when a family’s cultural, religious, or ethnic background, or even lifestyle, differs from that of the provider. Chapter 1 and Chapter 2 provides a framework for the clinician to examine personal values and for collecting patient data related to cultural and family assessments.
Goals of therapy and management of young children, especially those of preschool age, differ from goals in older children and adolescents. With their unpredictable physical activity, dietary intake, and increased sensitivity to insulin, young children often steer a course between high and low blood glucose. Overzealous treatment may result in frequent hypoglycemic reactions, even convulsions. Studies have demonstrated the detrimental effects of severe hypoglycemia on cognitive function in children with diabetes, specifically deficits on perceptual, motor, memory, and attention tasks (Rovet & Ehrlich, 1999). Hypoglycemic episodes can go undetected during the night, so-called nocturnal hypoglycemia (Beregszaszi et al., 1997). Therefore, most pediatric endocrinologists aim at preprandial glycemic values between 100 and 200 mg/dL in preschool children and between 90 and 140 mg/dL in older patients (Kiess et al., 1998).

It is important that the parents of a young child with diabetes understand the dangers of hypoglycemia. The provider must ascertain that they are able to monitor their child’s blood glucose at least four times a day or more and at an additional time of once a week at 2:00 AM. Glycosylated Hgb values in young patients may be maintained at higher levels than those recommended for older, school-age children. An HbA1c between 7% and 8.5% is quite acceptable for young children with diabetes.
Newer pharmacologic interventions and insulin delivery systems can be combined to achieve these therapeutic goals. Certified Diabetes Educators (CDEs) have curricula and teaching tools for empowering each person to control their diabetes effectively. As their knowledge base expands, both patient and provider must overcome reluctance to inject insulin and self-test glucose several times per day, while continuing to update their understanding of this disease.
Goals of Diabetes Management for All Treatment Options
The following current standards of practice can frame a plan of care that supports the patient with diabetes in obtaining optimal metabolic control. After young childhood, hyperglycemia must be eliminated to control the progression of diabetes and to delay or prevent complications, including microvascular and macrovascular problems. These two problems are discussed later in this chapter.
The immediate treatment goals of diabetes management include the following:
Achievement of optimal glycemic control based on HbA1c up to 8.5%, with preprandial glycemic values between 100 and 200 mg/dL in preschool-age children (Kiess et al., 1998)
HbA1c of 7.4% or less, with preprandial glycemic values between 90 and 140 mg/dL in older children and adolescents
Absence of hypoglycemic episodes requiring assistance to treat, including nocturnal hypoglycemia
The overall goals of diabetic management for all children and adolescents include the following:
Providing for normal growth and development to the greatest extent possible
Normalizing the activities of childhood, such as sports, camping, and peer group functions
Correction of metabolic irregularities
Achieving the glycemic range for age, as just discussed, to prevent consequent end organ damage due to microvascular and macrovascular complications
Achievement of these goals depends on maintaining the target blood glucose level. If frequent high and low blood sugar levels are encountered in the face of desirable HbA1c, efforts must be made to smooth out glycemic control within the age-related parameters just discussed. Patient self-monitoring of blood glucose (SMBG) is critical to the success of this effort, whether by the parent or caregiver of the young child or by self-monitoring in the older child and adolescent. Lifestyle assessment is an important first step in creating effective interventions for managing blood glucose.
Lifestyle Assessment and Intervention
The importance of understanding the child’s activities of daily life cannot be overstated. Included in this assessment are family meal planning; the child’s hours of sleep, school, and activity; and variations in activities on a day-to-day, weekly, and seasonal basis. The provider also needs to assess the parents’ educational level and employment, composition and involvement of the rest of the household, and potential for community support. Cultural and ethnic practices and religious beliefs may play an important role in health decisions, food choices, times of ritual fasting, and celebrations. Family habits and customs also may affect the child’s eventual self-management of diabetes. Ascertaining insurance status is important, because this often determines patient access to both primary care and specialty providers, support services, medication, and supplies (Songer et al., 1997). Chapter 1 provides a guideline for obtaining cultural and family assessment data.

Full access? Get Clinical Tree
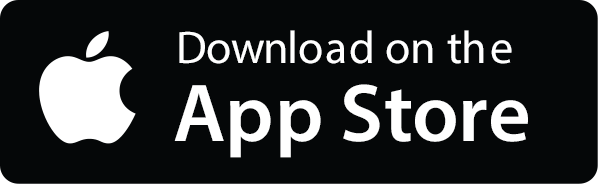
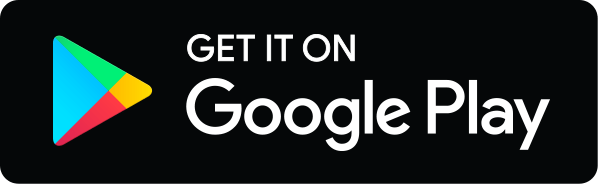