Package
PRBCs
Plasma
Platelets
Cryoprecipitate
Initiation
6 units (UD/TS)
6 units (UD)
1 (0.5 h)
6 units (UD/TS)
6 units (UD)
1 apheresisb
2 (1 h)
6 units (UD/TS)
6 units (TS)
20 units
3 (1.5 h)c
6 units (UD/TS)
6 units (TS)
1 apheresisb
4 (2 h)
6 units (UD/TS)
6 units (TS)
10 units
5 (2.5 h)
6 units (UD/TS)
6 units (TS)
1 apheresisb
6 (3 h)d
6 units (UD/TS)
6 units (TS)
10 units
A modern MTP aims to approximate delivery of a 1:1:1 ratio of RBC/fresh frozen plasma/platelets [10]. By addressing the early coagulopathy of trauma, MTPs have been shown to improve mortality in multiply injured populations [9, 11]. While the specific structure of MTPs varies slightly from center to center [12], they are all approximations of Sheldon’s fresh whole blood resuscitation principles from 1975 [13]. Additional benefits of a formal MTP include earlier administration of blood products during resuscitations, improved blood banking efficiency, decreased total blood product utilization during a hospital stay, and significant economic savings [14]. It must also be noted, however, that reasonable scientific concern remains with regard to the apparent improvement in survival via MTPs [15]. The possibility of a strong survival bias based on survivorship (i.e., surviving long enough to receive the most RBC units) remains [15]. It should also be noted that although the potential benefits of MTPs have been widely publicized, numerous theoretical and observational complications have been linked to MTPs using retrospective registry data. These include, but are not limited to, potentially increased risks of acute lung injury (ALI), acute respiratory distress syndrome (ARDS), hypothermia, and other risks associated with the transfusion of any blood product [16–18]. Further study is clearly warranted and ongoing.
Another major benefit of an MTP is the avoidance of excess crystalloid fluid administration [19]. This reduction in crystalloid volume during the resuscitation period minimizes multiple associated side effects including reperfusion injury, increased leukocyte adhesion and inflammation, associated acidosis, resultant acute respiratory distress syndrome, systemic inflammatory response syndrome, and multi-organ failure [20–22]. On an anecdotal platform, excess crystalloid administration also remains an obstacle to obtaining early definitive fascial closure of the abdominal wall secondary to both visceral and abdominal wall edema.
An adjunct to massive transfusion that should be considered in severely injured trauma patients is tranexamic acid (TXA). One gram is given over 10 min followed by 1 g given over the next 8 h administered within 3 h of injury [23]. TXA has been associated with a lower mortality [23, 24]—most pronounced in the patients undergoing massive transfusion [24]. While the CRASH-2 trial found no adverse events related to TXA administration [23], the MATTERs study found a low, but significantly increased risk of pulmonary embolism (2.7 %) and deep venous thrombosis (2.4 %) [24]. Military data has demonstrated that in massively transfused patients, TXA was independently associated with survival and improved coagulopathy [24]. The mechanism of action is not well understood, but TXA is thought to not only stabilize clots but attenuate the inflammatory response. It is inexpensive and has been deemed relatively safe, and it seems that few patients are needed to treat to achieve a mortality benefit; thus, its use has been growing [24].
Initially, the use of recombinant factor VIIa, a procoagulant used in hemophilia, appeared promising for coagulopathic blunt trauma patients, reducing the amount of transfusions and the need for massive transfusion [25]. Unfortunately, it did not affect mortality or reach statistical significance for penetrating trauma [25]. Subsequently, its safety was questioned when data demonstrated an increased risk of thromboembolic events [26]. The CONTROL trial, a prospective, randomized, double-blinded, multicenter study attempting to determine the efficacy and safety of factor VIIa, was terminated early when it did not demonstrate mortality benefit with questionable enrollment [27]. Ultimately, future studies should be directed at answering these questions. In the interim, factor VIIa is reserved for patients in extremis, usually undergoing massive transfusion, with 200 μg/kg given at hour 0 and 100 μg/kg at post-injury hours 1 and 3.
Permissive Hypotension
Further mention of the concept of permissive hypotension is also prudent and must reference the original randomized controlled trial by Mattox and colleagues in 1994 [28]. More specifically, this trial successfully challenged the dogma of restoring a patient’s blood pressure to physiologic levels in scenarios defined by ongoing hemorrhage. While this study was also heavily criticized due to the dominance of penetrating injuries and the proximity of patients to the trauma center, an updated prospective randomized trial has recently supported this initial observation in a multitude of injured patients [29]. This recent study noted that hypotensive resuscitation (MAP goal of 50 mmHg) is a safe strategy for injured patients that results in less overall blood product and intravenous fluid administration, decreased postoperative coagulopathy, and reduced early postoperative death [29]. Permissive hypotension is not appropriate for all populations, however, and should be avoided in the setting of potential concomitant head injuries and elderly population and if prolonged transport times or delays in definitive interventions are anticipated.
Damage Control Surgery and Its Indications
Once damage control surgery (DCS) was born, it was quickly marketed into other disciplines that included, but were not limited to, neck [30], vascular [31], orthopedic [32], thoracic [33], and military injuries [34]. The conceptual maturation of DCS has led to fundamental tenants that include (1) arresting surgical hemorrhage, (2) containment of gastrointestinal spillage, (3) surgical sponge insertion, and (4) temporary abdominal closure. This sequence is followed by immediate transfer to the intensive care unit with subsequent re-warming, correction of coagulopathy, and hemodynamic stabilization. Return to the operating theater is then pursued 6–48 h later for a planned reexploration that includes definitive repair and primary fascial closure if possible. It is clear that the DCS approach leads to improved survival for both blunt and penetrating injures in patients who are approaching physiologic exhaustion [35].
Despite the clear utility of DCS, its widespread propagation throughout the trauma community has led to a clear over-utilization of this technique. More specifically, multiply injured patients who are not approaching physiologic exhaustion are often exposed to the potential risks associated with open abdomens including infection, gastrointestinal fistula formation, and failure to achieve definitive closure with subsequent massive ventral hernias. As a result, the pertinent question remains: who needs DCS? The succinct response is “patients who are more likely to die from uncorrected shock states than from failure to complete organ repairs.” Depending on the center, these “metabolic cripples” encompass 3–8 % of all severely injured patients (penetrating vs. blunt; military vs. civilian). In essence, they continue to suffer the sequelae of tissue shock that is manifest as persistent hypothermia, persistent metabolic acidosis, and nonmechanical (i.e., nonsurgical) bleeding. More specifically, DCS triggers include core temperature <35 °C, pH < 7.2, base deficit >15, and/or significant coagulopathies [7, 36–38]. It must be emphasized, however, that not even all patients with initial physiologic deficits as significant as these values mandate DCS. With rapid arrest of hemorrhage, as well as ongoing resuscitation, some patients will improve dramatically in all parameters on repeated intraoperative blood gas analyses. These patients stabilize and begin to recover. It should also be stated that patients with multiple intra-abdominal injuries are not always in metabolic failure.
Nonvascular persistent abdominal bleeding is most commonly related to the liver, spleen, pancreas, and/or kidney. Unlike the spleen and kidney, the liver and pancreas cannot generally be resected in a rapid on-demand basis. It should be noted however that prior to the removal of any kidney, palpation for a normal-sized contralateral kidney must be completed.
Technical details surrounding hepatic hemorrhage include leaving the falciform ligament intact to provide a medial wall against which to improve packing pressure (especially in blunt trauma). If hemorrhage continues, an early Pringle maneuver (clamping of the porta hepatis with a vascular clamp) is mandated as both a diagnostic and potentially therapeutic technique. If bleeding continues despite application of a Pringle clamp, a retrohepatic IVC or hepatic venous injury is likely. It should be noted that critically injured patients in physiologic extremis do not tolerate extended Pringle maneuvers to the same extent as patients with hepatic tumors undergoing elective hepatic resection (40 min upper limit). If the liver hemorrhage responds to packing but continues to hemorrhage when unpacking is completed, the patient should be repacked and transferred to the ICU with an open abdomen once damage control of concurrent injuries is complete. Covering the liver with a plastic layer of sterile x-ray cassette material avoids capsular trauma/oozing upon eventual unpacking. In the case of central hepatic gunshot wounds or deep central lacerations where access and exposure are difficult, ongoing hemorrhage can be stopped with balloon occlusion (see below). Return to the operating suite in patients with packed livers should occur in 72 h (assuming hypothermia, coagulopathy, and acidosis are corrected).
It should be noted that although the published history of hepatic trauma is littered with descriptions of various technical maneuvers ordered in a hierarchical scheme, very few are relevant in context of modern trauma care. More specifically, damage control packing of hepatic hemorrhage controls the vast majority of ongoing bleeding in critically ill patients.
Damage control pancreatic maneuvers are extremely limited and revolve around drainage of nearly all pancreatic injuries (either with a closed suction drain and an open abdomen or simply open abdomen alone). The dominant associated life-threatening scenario for peripancreatic injuries involves hemorrhage from the portal and superior mesenteric veins. Although these veins can be ligated, repairs are generally performed with 5-0 or 6-0 Prolene once control is obtained. Clamps above and below the injury are essential for visualization. Alternate damage control options include temporary intravascular shunts (TIVS) (described below) with a small chest tube conduit or ligation (assuming the hepatic artery is intact) [39]. Damage control maneuvers for the splenic vein are generally limited to bulk ligation.
Damage control technique for hollow viscous organs require ligation and subsequent exclusion of injured and/or leaking segments. This is typically performed using gastrointestinal staplers. The supplying mesenteries can be ligated in rapid fashion using clamps or vascular staplers. In the case of gastric division, the proximal stomach should be decompressed by a nasogastric tube. Small bowel and colon can be left in discontinuity for up to 48 h with minimal sequelae.
Vascular Damage Control Techniques
Although it is clear that arresting ongoing hemorrhage is the most crucial of damage control tenets, vascular damage control has been traditionally limited to vessel ligation. More recently, however, balloon catheter tamponade and temporary intravascular shunts (TIVS) have increased in popularity. The impressive utility of balloon catheters for tamponade of exsanguinating hemorrhage has a long history dating back more than 50 years [40]. Although this technique was originally described for esophageal varices [41], it was quickly extended to patients with traumatic vascular and solid organ injuries [42]. Since the initial treatment of an iliac arteriovenous lesion in 1960 [3], balloon catheters have also been used for cardiac [43], aortic [44], pelvic [45], neck (carotid, vertebral, and jugular) [46, 47], abdominal vascular [48], hepatic [49], subclavian [50], vertebral [40], and facial trauma [51]. While this technique was originally intended as an intraoperative endovascular tool [40], it has since been employed as an emergency room maneuver with the balloon being placed outside of the lumen of the injured vessel usually through the wound tract [52, 53].
Modern indications for this damage control technique are limited. This is primarily because routine methods for controlling hemorrhage, such as direct pressure, are typically successful. As a result, indications for catheter tamponade include (1) inaccessible (or difficult to access) major vascular injuries, (2) large cardiac injuries, and (3) deep solid organ parenchymal hemorrhage (liver and lung) [40, 43]. Of interest, the type of balloon catheter (Foley, Fogarty, Blakemore, or Penrose with red rubber Robinson), as well as the duration of indwelling, can vary significantly. In conclusion, balloon catheter tamponade is a valuable tool for damage control of exsanguinating hemorrhage when direct pressure fails or tourniquets are not applicable. It can be employed in multiple anatomic regions and for variable patterns of injury. Prolonged catheter placement for maintenance of hemostasis is particularly useful for central hepatic gunshot injuries [53].
Temporary intravascular shunts (TIVS) are intraluminal synthetic conduits that offer nonpermanent maintenance of arterial inflow and/or venous outflow [54]. As a result, they are frequently life and limb saving when patient physiology is hostile. By bridging a damaged vessel and maintaining blood flow, they address both acute hemorrhage and critical warm ischemia of distal organs and limbs. Although Eger and colleagues are commonly credited for pioneering the use of TIVS in modern vascular trauma [55], this technique was initially employed by Carrel in animal experiments [56]. The first documented use in humans occurred in 1915 when Tuffier employed paraffin-coated silver tubes to bridge injured arteries [57]. This technique evolved from glass to plastic conduits in World War II [58] and continues to vary both in structure and material among today’s surgeons [59].
Modern indications for TIVS include (1) replantation, (2) open extremity fractures with concurrent extensive soft tissue loss and arterial injury (Gustilo IIIC), (3) peripheral vascular damage control, (4) truncal vascular damage control, and (5) temporary stabilization prior to transport [54, 60]. While the understanding of TIVS use for military and civilian settings is increasing [59], the optimal shunt material, dwell time, and anticoagulation requirements remain poorly studied. It can be noted, however, that TIVS are remarkably durable and rarely clot unless they (1) are too small (diameter), (2) kink because of inappropriate length, and/or (3) are placed in an extremity without appropriate (or shunted) venous outflow (venous hypertension leads to arterial thrombosis) [60].
Despite the penetrating mechanism dogma associated with TIVS over the past 40 years, the majority (64 %) of TIVS in a large national database (National Trauma Data Bank—NTDB) were used in patients injured via a blunt mechanism [61]. Although the kinetic force of an motor vehicle crash (MVC) or MVC-pedestrian collision can be tremendous, TIVS is often discussed in the context of extremity damage control for gunshot wounds in patients with hostile physiology [61]. This NTDB analysis however indicated that most extremity TIVS are actually placed for blunt vascular trauma associated with extensive orthopedic and/or soft tissue injuries (74 %). They are also most often used as a temporizing maneuver to provide distal flow to a limb while orthopedic injuries are assessed and fixated. The use of TIVS for this scenario is well recognized and documented to significantly reduce the rate of amputation. In the patients who did not undergo TIVS for fractures and soft tissue defects, it appears that shunting was employed as an extremity damage control technique in those who presented with hemodynamic instability and severe base deficits (26 %) [61]. These patients displayed a much lower level of subsequent amputation when compared to cases of blunt trauma with concurrent fractures and soft tissue trauma. In addition to using TIVS in blunt-injured patients, the NTDB also indicates that this technique is being performed relatively uncommonly across a wide range of hospitals [61]. Of 111 trauma centers employing TIVS, only six used five or more shunts throughout the study period. Additionally, only three centers employed more than ten shunts. TIVS appear to be useful in any scenario with a major vascular injury and hostile patient physiology. This includes cases of blunt MVC trauma with concurrent severe extremity fractures and/or soft tissue injuries. In spite of their simplicity, however, they are underutilized.
Abdominal Compartment Syndrome
Abdominal compartment syndrome (ACS) is defined as sustained intra-abdominal pressure greater than 20 mmHg that is associated with new organ dysfunction/failure [62–67]. ACS differs from intra-abdominal hypertension (IAH) which is a graded (I–IV) and sustained pathological elevation greater than 12 mmHg. Symptoms of ACS are extensive and impact every major system within the human body. These include, but are not limited to, cardiovascular (hypotension), renal (acute kidney injury), and respiratory (failure) elements. It is interesting to note that many of the dominant risk factors for developing ACS mirror the physiologic triggers for engaging in DCS/DCR [62, 63]. This observation supports these physiologic variables (pH, base deficit, core temperature) as clear markers for the absolute “sickest of the sick.” Closing abdomens in patients manifesting physiologic extremis often leads to ACS, as first demonstrated by Morris Jr. and colleagues in 1993 [64]. With closure, these authors described severe abdominal distension in concert with raised peak airway pressures, CO2 retention, and oliguria [64]. Their observed 63 % mortality rate associated with reperfusion injury after unpacking was also dramatic and currently emphasizes the importance of “recurrent” or “tertiary” ACS [65]. While the incidence of primary ACS has decreased dramatically over the past decade [62], continued vigilance is crucial to guard against secondary and recurrent ACS. Despite the increased understanding surrounding this anatomic and physiologic complication, however, it is clear that the actual practice of clinicians requires more education with regard to both monitoring and treating ACS [66]. This reality has led to a recent evidence-based update of both definitions of primary, secondary, and recurrent ACS and an expert society’s therapeutic recommendations [67]. More specifically, in addition to multiple “suggestions,” the World Congress of the Abdominal Compartment Syndrome (WCACS) strongly recommends the following: (1) measuring IAP when any known risk factor for IAH/ACS is present in a critically ill or injured patient using a trans-bladder technique (GRADE 1C), (2) utilizing protocolized monitoring and management of IAP (GRADE 1C), (3) engaging in a decompressive laparotomy for cases of overt ACS (GRADE 1D), (4) attempting to ensure the-same-hospital-stay abdominal fascial closure (GRADE 1D), and (5) utilizing negative suction therapy in patients with open abdominal cavities (GRADE 1D) [67]. It should also be noted that some patients may adequately respond to decompression (nasogastric, colonic, intraperitoneal (i.e., ascites)) and/or increased sedation/paralysis as primary therapeutic maneuvers. Failure to resolve ACS with these medical therapies, however, should lead to rapid surgical decompression. Measuring intra-abdominal pressures can be easily performed at the bedside with a three-way Foley catheter, pressure transducer, and intravenous tubing.

Full access? Get Clinical Tree
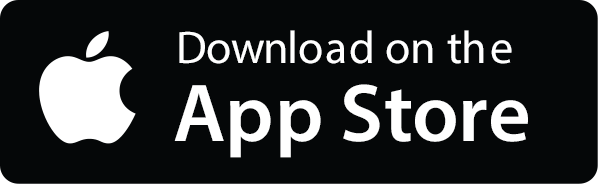
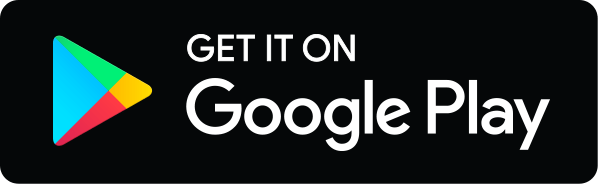