Cigarette smoking is well recognized for its contribution to perioperative morbidity via effects on the cardiovascular system, mucus secretion and clearance, and small airway narrowing. Although patients are invariably counseled to stop smoking prior to elective surgery, data from coronary artery bypass patients suggests this should occur at least 8 weeks prior to surgery, because smoking cessation just prior to surgery may actually increase the risk of postoperative pulmonary complications (7), probably due to transient increases in sputum volume. In the case of cancer, however, surgery is rarely delayed and aggressive pulmonary toilet including liberal use of bronchoscopy may be employed.
Major operations such as lobectomy and many pleural procedures are now accomplished without thoracotomy and the use of rib retraction. Video-assisted thoracic surgery (VATS) has become a more standard approach in major lung resection. The physiologic insult created using this technique has changed the acceptable risk profile for patients undergoing thoracic procedures. VATS lobectomy has been shown to have decreased morbidity, lower narcotic requirement, and quicker recovery time, as well as less delirium in the elderly and an earlier return to ambulation (8–12). This decrease in postoperative morbidity has allowed for these operations to be performed on patients with preoperative functional status that would be unacceptable for thoracotomy.
TABLE 61.2 Respiratory Failure Risk Index | |
![]() |
Expectations as to the duration of postoperative respiratory failure allow the caregiver to heighten his or her awareness if the patient behaves in unanticipated ways. A very large patient population from the Veterans Affairs Medical Centers provided a database for researchers to learn what factors play a role in predicting postoperative respiratory failure (13). Factors negatively influencing outcome included the type of surgery, emergency surgery, low preoperative albumin, high preoperative BUN, partial or full dependent status, COPD, and age greater than 60 years (7). These factors are all assigned a point status (Table 61.2); more points increase the probability of postoperative respiratory failure (Table 61.3). It is important to realize women were excluded from data collection in this study but the factors noted do not have gender specificity.
OPERATING ROOM EVENTS IMPACTING ICU CARE
The pace of postoperative recovery depends on the amount and types of anesthetic agents given as premedication and during the operative procedure. Anesthetic delivery is constrained by patient factors. For example, the need for high FiO2, particularly during one-lung anesthesia, limits the ability to use nitrous oxide (N2O). In general, the FiO2 should be minimized due to concerns for oxygen-induced pulmonary toxicity, particularly in patients who have received neoadjuvant radiation and/or chemotherapy (14). A goal of early extubation limits the use of opioids. Regional techniques—spinal, epidural—can supplement general anesthesia but are generally not applicable to operative anesthesia for open thoracic procedures because of the difficulty in providing a high-enough spinal level. Controlled ventilation is necessary to sustain respiration during open-thorax procedures. For most procedures, the plan is to have the patient awake, comfortable, and extubated at the end of the procedure, thus avoiding the potential stress on fresh suture lines from positive-pressure ventilation and coughing or bucking on the endotracheal tube.
TABLE 61.3 Respiratory Failure Risk Index Scores and Outcomes |
![]() |
Selective endobronchial intubation with isolation of the right and left lungs permits the surgeon to operate on a quiet, collapsed lung, while the contralateral side is ventilated. Disposable polyvinyl double-lumen tubes are available in odd sizes between 35 and 41 French in both right-sided and left-sided configurations. The nonoperative bronchus is usually chosen for selective intubation in lobectomies and pneumonectomies, so that surgical manipulation does not displace the tube, and to allow resection of the mainstem bronchus if necessary. When selective endobronchial intubation is impossible—as in pediatric patients, very small adults, laryngectomy patients—a bronchial blocker can be placed under fiberoptic guidance to selectively occlude a bronchus.
One-lung ventilation alters the ventilation–perfusion relationship as blood passing through the unventilated lung effectively causes a right-to-left shunt, reducing arterial saturation. Perfusion of the unventilated lung will be reduced somewhat by physical collapse of the lung and hypoxic pulmonary vasoconstriction. The double-lumen endotracheal tube (DLETT) is large and has the potential to cause airway trauma and edema, may shift its position, and is a more challenging suction portal. The DLETT is generally removed at the end of the operation and replaced by a single-lumen tube when continued mechanical ventilation is required. Specific indications for continued postoperative selective endobronchial intubation include the need to protect the lung against soilage—pus or blood—and provision of different levels of positive end-expiratory pressure (PEEP) to differentially compliant lungs in emphysematous patients undergoing single-lung transplantation. A fiberglass resin tube-changer and a pediatric (small diameter) fiberoptic bronchoscope are essential tools, which should be available in the ICU for placement and adjustment of double-lumen tubes.
Minimally invasive techniques have been used with increasing frequency in thoracic surgery. These include VATS for major anatomic lung resection, pleural space procedures, and minimally invasive esophagectomy; robotic-assisted surgical procedures are performed through similar incisions. The benefit to smaller incisions, with no retraction placed on ribs or abdominal musculature, has improved the postoperative complication and recovery profile for these operations. The use of VATS in the resection of early-stage lung cancer has resulted in a decrease in overall complications, pulmonary complications, atrial dysrhythmias, and need for transfusion (15).
The choice of location for postoperative recovery depends on the degree of patient illness, and the ability of a particular nursing unit to deal with postoperative ventilation and/or hemodynamic monitoring. At many hospitals, patients undergoing bronchoscopy, mediastinoscopy, esophageal dilatation, esophagoscopy, gastrostomy, jejunostomy, laryngoscopy, pleuroscopy, or scalene node biopsy can spend a short time in the postanesthesia recovery unit (PACU) and then be transferred to a step-down or general nursing floor, or even sent home. Patients undergoing lobectomy, segmental or wedge pulmonary resections, hiatal hernia repairs, or Heller myotomy can generally be recovered in PACU and then sent to a step-down unit if there are no complications. Patients undergoing esophagectomy, esophagogastrectomy, and pneumonectomy are likely to have ongoing monitoring or postoperative ventilation needs, and generally are managed in an ICU.
IMMEDIATE POSTOPERATIVE ISSUES
Usual postoperative monitoring includes intermittent blood pressure determinations, continuous electrocardiography, and pulse oximetry. In selected patients, assessing intravascular volume status and cardiopulmonary function may be facilitated with central venous pressure or pulmonary artery catheters but is rarely necessary.
Chest tubes are usually inserted to drain the surgical site at the end of the procedure, except with pneumonectomy patients where standard practice is to avoid a chest tube unless there is the need to monitor the pneumonectomy space postoperatively. Chest tubes should never be clamped during patient transport, because of the dangers of unrecognized bleeding and tension pneumothorax. Chest tubes, except for those in pneumonectomy spaces, are usually connected to a vacuum regulator to provide negative 20 cm H2O suction. A chest radiograph will confirm endotracheal, nasogastric, and chest tube placement, and identify any pneumothorax, mediastinal shift, or significant atelectasis. Routine chest x-rays are not necessary after uncomplicated removal of chest tubes and the decision to reinsert a chest tube is usually based on clinical appearance rather than radiologic findings (16).
Commercially available chest tube systems vary in their appearance, but all provide calibrated drainage chambers, a method to release excess positive pressure, and regulated amounts of negative pressure. Air bubbles are normally expected in the chamber that limits the amount of applied suction; air bubbles in the water seal chamber represent an active leak.
Thoracic drain devices have been developed to quantitatively measure air flow through the system as a measurement of the size of an air leak. This allows for greater confidence in the determination of when a leak has sealed. Data is collected and stored locally which can be visualized in a graph format allowing visual assessment of the trend of air flow (Thopaz drain, Medela Switzerland). Use of this system has allowed for earlier chest tube removal (17).
Hourly output from chest tubes should be recorded, and the operative team notified if drainage is greater than 100 mL/hr for more than 4 hours, or if greater than 200 mL of drainage is recorded in any 1-hour observation period. Expected chest tube drainage from major procedures in the first 24 hours is roughly 300 to 600 mL, tapering to less than 200 mL by the second day. Daily chest radiographs are usually obtained while chest tubes are in place. The level of fluid in the water seal chamber should fluctuate with each respiration (assuming no air leak) and serves as confirmation of chest tube patency. Most pulmonary resection patients will return with mild-to-moderate air leaks, which become problematic only if the underlying lung parenchyma does not completely expand to fill the pleural space, or if a significant percentage of tidal volume is lost through the chest tubes with mechanical ventilation. Additional pleural drainage may then be required, or changes in ventilation made to minimize the air leak and optimize ventilation. Leaks may occur only above a given inflation pressure, and ventilation techniques such as smaller volumes at higher rates, pressure-controlled inverse ratio ventilation (IRV), or high-frequency oscillation (HFO) can minimize leaks and allow a seal to develop. Once all air leaks resolve and drainage is minimal—less than 100 mL/24 hr—chest tubes may be removed during the expiratory phase of ventilation or while the patient performs a Valsalva maneuver.
Prophylactic PEEP is sometimes used in an effort to decrease postoperative drain output, especially from mediastinal drains in cardiac surgery patients. The evidence, however, suggests higher PEEP levels do not affect chest tube output or transfusion requirements (18).
Extubation and Airway Concerns
Extubation can often be accomplished in the operating room, but continued ventilation may be necessary with concurrent cardiac illness, inability to protect airway, malnutrition, or coexisting lung disease. Silent aspiration of gastric contents is an important complication following pulmonary resections, and maintenance of endotracheal intubation for 24 hours postoperatively has been shown to decrease the occurrence of pneumonia and the operative mortality rate (19) in high-risk patients.
Measurement of maximal inspiratory pressure (MIP)—often called negative inspiratory force (NIF)—is helpful in determining respiratory muscle strength, particularly in patients recovering from thymectomy for myasthenia gravis, and in those who received long-acting neuromuscular blocking agents in the operating room. Residual neuromuscular blockade can be assessed using a train-of-four monitor and reversed, if necessary, with small doses of neostigmine plus vagolytic agents such as atropine or glycopyrrolate. Ideally, the patient should be awake and following instructions, and have an adequate gag reflex—signifying airway protection—and cough—for secretion clearance. Measured parameters suggesting readiness for extubation include a respiratory rate to tidal volume (f/Vt) ratio of <100, an MIP of greater than 25 cm H2O and adequate oxygen saturation (≥92%) on FiO2 ≤50% at PEEP ≤5 cm H2O. Although many patients will not strictly meet these criteria for extubation, it is usually best to attempt weaning and extubation rather than risk of the complications of continued ventilation. Specific indications to delay extubation are in Table 61.4.
TABLE 61.4 Selected Indications for Continued Postoperative Ventilation |
![]() |
Laryngeal and glottic edema frequently occurs after airway manipulation or intubation with a large DLETT. The presence of serious laryngeal edema can be detected—after first suctioning the posterior pharynx—by deflating the endotracheal tube cuff, and occluding the endotracheal tube and watching for evidence of airway obstruction. Endotracheal intubation may need to be maintained while edema resolves; racemic epinephrine and corticosteroids are traditionally used, although the literature support for this is sparse. If there is any doubt about airway patency, the endotracheal tube should be removed only under direct laryngoscopic or fiberoptic observation, with a percutaneous tracheostomy set immediately at hand to provide airway access should re-intubation be impossible because of airway swelling.
Only a minority of thoracic surgery patients require postoperative ventilation and, in these, reduction of barotrauma becomes an additional consideration. Mechanical ventilation is associated with cytokine production, and even conventional tidal volumes may contribute to lung injury in critically ill patients (20). Low tidal volumes (6 mL/kg) are recommended in patients at risk for ARDS (21), but more research is needed to conclude whether a lung-protective strategy benefits all patients (22). The normal inspiratory to expiratory ratio is about 1:2, and inspiratory times longer than 1 second are poorly tolerated in awake patients. Longer inspiratory times reduce peak airway pressure, but often require addition of sedative agents and in patients with significant airway obstruction may not allow sufficient time for exhalation, resulting in auto-PEEP with consequent hemodynamic compromise.
Assist control, intermittent mandatory ventilation, or continuous positive airway pressure with pressure support can be employed. Evidence is insufficient to conclude whether pressure-controlled ventilation is superior or inferior to volume-controlled ventilation with acute lung injury (23). The FiO2 in the early postoperative period is generally set at 0.5 to 0.6, and then reduced as clinically appropriate. The combination of pulse oximetry and end-tidal carbon dioxide monitoring will reduce the need for frequent arterial blood gas sampling. Controversy still exists as to the optimal level of PEEP in the thoracic surgery patient. Low levels of PEEP (3 to 5 cm H2O) may be helpful in restoring FRC and substituting for the “physiologic PEEP” of the glottis.
Airway pressure release ventilation (APRV) is a pressure-controlled mode of ventilation; exhalation occurs when the inflating pressure is released from a sustained high level. With the majority of time spent in the high-pressure phase, it is thus a form of IRV. Because it allows spontaneous breathing, it is often better tolerated, with less sedation, than pressure-controlled IRV (PC-IRV). The higher mean airway pressures may be a concern with air leaks, but APRV has been shown to improve oxygenation, particularly in thoracic trauma patients (24).
Neurally adjusted ventilator assist (NAVA) is emerging as a method to better match ventilator assistance to patient needs, by assessing diaphragmatic electrical activity via an esophageal probe (25). As diaphragmatic activity occurs before airway flow or pressure changes, synchrony between the patient and the ventilator may be improved using NAVA, although more clinical studies are presently needed.
HFO ventilation is the logic extension of the “open lung” concept; cyclic exchange of tidal volume is replaced by sustained inflation with air exchange taking place by a variety of mechanisms including convection and diffusion stimulated by oscillations in the range of 3 to 7 Hz (equivalent to 180 to 420 breaths/min). While long employed in neonatal critical care, HFO has been widely adopted for adults with ARDS; recent literature suggests no advantage and possible harm in a subset of patients where HFO was applied early in adults with moderate to severe ARDS (26). HFO requires a specialized ventilator (Sensormedics 3100B, Viasys Healthcare, Yorba Linda, CA). High-frequency jet ventilation (HFJV), which involves rapid pulses of air entraining additional volume, has a role in the operating room during “shared airway” procedures (i.e., laryngoscopy, bronchoscopy, microlaryngeal procedures, and airway surgery). The role of HFJV in the ICU, particularly for management of hypoxemic respiratory failure, is poorly defined; the one exception being ventilation of a patient with a bronchopleural fistula. In theory, HFJV allows ventilation at lower airway pressures than conventional ventilation. The reduction in ventilation pressure will minimize the amount of air passing through the fistula and may promote healing by allowing adjacent tissues to approximate and possibly seal the fistula. In the face of decreased pulmonary compliance, the beneficial effect of HFJV in lowering airway pressure may be lost (27).
Noninvasive ventilation (NIV) has been shown to improve survival in acute care patients, particularly when applied early rather than as a rescue treatment. A recent review summarizes 178 studies, including the use of NIV for prevention and treatment of postextubation and postoperative acute respiratory failure (28).
Postoperative Fluid Management
Thoracic surgery patients present unique issues in terms of fluid management in the postoperative period due to the heightened potential for pulmonary edema. Postpneumonectomy pulmonary edema occurs in approximately 4% to 27% of patients (29,30) and pulmonary edema from all causes in 27% of pneumonectomy patients (14). Understanding the contribution of insensible—600 to 1200 mL/d in a 70-kg adult—and measured fluid loss during the surgical procedure provides valuable information when anticipating the patient’s needs in the postoperative period. Thoracic surgery patients may also have operative losses of 6 to 8 mL/kg/hr of “third-space” fluid to the interstitial space and intracavitary areas (31). The choice of fluid for resuscitation is left to the discretion of the caregiver as there is no known difference in outcome with the use of either isotonic crystalloid or colloid.
During their procedures, patients are exposed to intraoperative handling of the lung, fresh frozen plasma (FFP), prolonged one-lung ventilation, collapse and re-expansion of the lung, as well as increases in postresection pulmonary artery pressures (32). These factors all contribute to the lung parenchyma being primed for a more profound inflammatory response and potential fluid accumulation. Patients undergoing procedures involving the mediastinum, such as esophagectomies or tumor excision, experience even more profound fluid shifts and pose a greater management challenge in the postoperative period.
There is no formula applicable across the broad spectrum of patient types seen in this population to adequately predict fluid needs. Traditional markers of perfusion help determine if a patient is adequately volume resuscitated. These include urine output—usually over 0.5 mL/kg/hr—mental status, blood pressure, heart rate, blood lactate level, capillary refill time, venous oxygen saturation, and filling pressures and cardiac performance. Pulse pressure and stroke volume variation (SVV) can be helpful in assessing preload in mechanically ventilated patients; PPV and SVV are less useful in the operating room when the chest is open (33).
Resection patients, especially if a right-sided pneumonectomy and experiencing high ventilatory pressures during surgery (14), require greater scrutiny when determining fluid needs due to the increased risk for postpneumonectomy pulmonary edema. Ideally, the clinician will limit crystalloid infusion to 20 mL/kg for the first 24 hours in this cohort (13). If a state of poor perfusion persists, then invasive devices allowing for precise hemodynamic monitoring and oxygen consumption need consideration in an effort to accurately establish goals of therapy.
Pain Management
The pain associated with thoracotomy is considered one of the most intense of any surgical procedures (34); adequate pain control is important not only to ensure patient comfort but also avoid potential cardiac and pulmonary complications. Early pain management is also important in an effort to reduce the chances of developing long-term postthoracotomy pain (35). The reasons for pain in this setting are many and include the skin incision, dissection of the intercostal muscles and pleura, pleural irritation, chest tube insertion, and prolonged rib retraction leading to ligamentous and muscle injury (15). Without satisfactory pain relief the patient is exposed to adverse effects, including an inability to breathe deeply which decreases vital capacity and FRC. Splinting also occurs making it more difficult to clear secretions. These factors increase the likelihood of developing respiratory failure in the postoperative period. The cardiovascular system is at risk, as pain is associated with elevated circulating levels of catecholamines, which act on the myocardium to increase oxygen consumption.
A variety of options exist for pain management, including systemic analgesics, neuraxial opioids, and local anesthetics via the epidural or intrathecal route, regional anesthesia such as intercostal and paravertebral nerve blocks, and adjuvant therapies such as transcutaneous electrical nerve stimulation (TENS) or applied heat.
The mainstay of postoperative pain control is systemic analgesics in the form of opioids. Agents such as morphine, fentanyl, and hydromorphone are frequently used and can be administered intravenously, subcutaneously, or intramuscularly, with the intravenous route providing the most predictable responses; barring an emergency, intramuscular injections would be considered less than optimal. Opioid side effects remain the greatest issue with respiratory depression, nausea, vomiting, and ileus being a few examples. Nonopiate medications such as nonsteroidal anti-inflammatory agents, including the parenteral prostaglandin inhibitor ketorolac (36), are reasonable adjuncts to the opioids. It is necessary to exercise caution when using NSAIDs in the face of underlying renal insufficiency as their use may exacerbate the renal dysfunction. Additionally, NSAIDs may pose a risk with postoperative healing; one animal model demonstrated less effective pleural adhesions following pleurodesis (37).
Neuraxial opioids and local anesthetics via the epidural or intrathecal route provide excellent regional pain control. Epidural catheters have been the preferred route, and when local anesthetics, either with or without opioids, are infused in this manner the incidence of pulmonary complications decrease relative to systemic opioids (38). The initiation of epidural catheters prior to the operation appears to be the ideal approach as it allows for better management of pain in the postoperative setting (39). Hypotension due to sympathetic blockade is a potential side effect when local anesthetics, such as bupivicaine, are administered. Therefore, it may be necessary to either decrease the dose or eliminate the local anesthetic completely from the infusion and utilize opioids exclusively.
Intercostal and paravertebral nerve blocks provide for regional pain control; these may be performed either intraoperatively or postoperatively, provide relief lasting up to 12 hours, may need repetitive dosing, and can even be accomplished with cryoablation of the intercostal nerves during the surgery (40). Chest tube insertion sites are potential sites of discomfort and may be blocked either directly or proximally. Intercostal nerve blocks are relatively contraindicated in postpneumonectomy patients due to the risks of entering and contaminating the empty chest cavity.
ON-Q Pain Relief System
The On-Q® system (On-Q, Lake Forest, CA) consists of an elastomeric ball reservoir for local anesthetic such as bupivacaine or ropivacaine, connected to a long fine catheter. The catheter can be tunneled to provide a multilevel paraspinal block and may be placed at the bedside or in the operating room at thoracotomy (41). These long paraspinal blocks are a viable alternative to an epidural catheter for the management of both rib fracture and postthoracotomy pain; the local anesthetics provide an opioid-sparing effect (42,43).
The described methods are some of the traditional modalities utilized when controlling pain in the thoracic surgery patient. TENS, heat and cold application, music therapy, and relaxation techniques are additional means of providing a comfortable setting (23). In addition, patients requiring prolonged postoperative mechanical ventilation may benefit from the centrally acting alpha adrenergic agonist dexmedetomidine, which reduces the amount of narcotic needed for pain control (44) and is associated with less delirium than other agents.
SPECIFIC PATIENT POPULATIONS
Thoracic Trauma
Trauma patients are typically evaluated and treated for acute, life-threatening injuries prior to ICU arrival. The critical care physicians’ role is to understand the nature of the injuries, whether blunt or penetrating, and the anticipated clinical course. In addition, maintaining a high degree of vigilance is paramount for diagnosing potential missed injuries.
Typical blunt injuries to the chest include rib fractures, flail chest, hemothorax, pneumothorax, tension pneumothorax, pulmonary contusion, cardiac contusion, and aortic disruption. Penetrating traumas such as gunshots and stabbings are less predictable in terms of the injuries generated and therefore require a case-by-case assessment in terms of management issues. Uncontrolled hemoptysis or cavitary lesions following penetrating injury require emergent surgical intervention.
Mortality increases in thoracic trauma with increasing age, lower Glasgow Coma Scale scores, liver injury, splenic injury, >5 rib fractures, and long-bone fractures. Mortality rates typically are between 9% and 20% in the United States (45). If the patient suffers an out-of-hospital cardiac arrest in relation to their trauma, the chances of survival diminish even further, with less than 10% of patients in this group surviving to hospital discharge (46).
Rib fractures are the most common type of chest trauma with ribs five through nine the most susceptible; while this injury is rarely life-threatening, rib fractures may serve as indicators for more severe intrathoracic or intra-abdominal injuries. Pain may be significant, leading to splinting, hypoventilation, atelectasis, and potentially pneumonia as pulmonary toilet is compromised. First and/or second rib fractures indicate a large transfer of energy to the thoracic cage and should raise further suspicion for other intrathoracic problems, such as aortic rupture or tear (47). Patients aged 65 and older pose a particular problem when faced with these types of injuries as, with each rib fracture, mortality increases by 19% and the risk of pneumonia by 27% (48). The implications of age begin at 45 years as those with four or more rib fractures in this group show more in-hospital complications, such as increased ventilator and ICU days (49).
Flail chest (Fig. 61.1) is defined as three or more adjacent ribs fractured at two or more sites creating a segment of paradoxical wall motion; any large number of rib fractures can similarly impair respiratory mechanics, especially, as is often the case, there is associated pulmonary contusion. Mainstays of management include aggressive pain control (including the use of either an epidural catheter or On-Q device), aggressive pulmonary toilet, and both invasive and noninvasive mechanical ventilation. Stabilization of rib fractures through surgical internal fixation has demonstrated both reduced pain and increased pulmonary function with subsequent earlier separation from mechanical ventilation. In some patients at risk for respiratory decompensation, mechanical ventilation may be avoided altogether (50).

FIGURE 61.1 There are multiple fractured ribs on the right with several fractured in more than one place. This leads to a flail segment with inspiration.

Full access? Get Clinical Tree
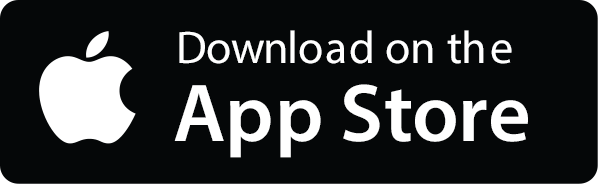
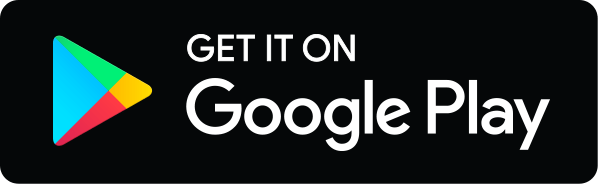