Fig. 1
Changes in the nociceptive system during pathophysiologic nociceptive pain and neuropathic pain. Spinal sensitization and increased hyperexcitability at the supraspinal level form the process of central sensitization
At the peripheral level distinct processes were observed which characterize pathophysiologic nociceptive and neuropathic pain. The hallmark of pathophysiologic nociceptive pain, e.g., pain during inflammation or after tissue injury, is peripheral sensitization. Nociceptive nerve fibers exhibit a lowering of their excitation threshold for the response to mechanical and/or thermal stimuli and increased firing frequencies during the application of stimuli of noxious intensities. Such processes were characterized in the skin, muscle, joint, and visceral organs (Schaible and Richter 2004). Molecular mechanisms of peripheral sensitization are addressed in Sect. 1.4 (see below). More recently, the concept of hyperalgesic priming was introduced (see Kandasamy and Price 2015). Priming arises from an initial injury and results in the development of a remarkable susceptibility to normally subthreshold noxious inputs causing a prolonged pain state in primed animals. Priming increases the sensitization process which is evoked by sensitizing mediators. As an example, application of prostaglandin E2 to normal tissue causes a short-lasting sensitization of nociceptors if applied before injury or priming. However, if the neurons were primed, e.g., by interleukin-6, NGF, and other priming stimuli, prostaglandin E2 will cause a long-lasting sensitization (see Kandasamy and Price 2015).
A frequent process of neuropathic pain at the peripheral level is the generation of ectopic discharges. These action potentials can be elicited at the lesion site of the nerve fibers, but they can also be generated in the soma of the lesioned neurons (Devor 2009). Underlying mechanisms are changes in the expression of ion channels, actions of inflammatory mediators on lesioned fibers, and effects of the sympathetic nervous system on lesioned nerve fibers. In the latter case the neuropathic pain may be sympathetically maintained (Schaible and Richter 2004).
Peripheral nociceptive processes often trigger changes in the spinal cord which are called central sensitization. The changes in the spinal cord provide a gain of the nociceptive processing at the spinal site (Cervero 2009; Woolf and Salter 2000). Nociceptive spinal cord neurons which receive increased input from inflamed regions show the following phenomena: a lowering of threshold, increased responses to innocuous and noxious stimuli, and an expansion of the receptive fields (Schaible et al. 2009). In the sensitized state, more spinal cord neurons show responses to a stimulus applied to a specific peripheral site. These changes reflect an increase of the synaptic processing including the suprathreshold activation of synapses which may be too weak in the normal state to depolarize the neuron sufficiently. In many aspects these processes are similar to the long-term potentiation which was characterized as a major process of memory formation in the hippocampus (Sandkühler 2000). Central sensitization is also thought to occur in neuropathic pain states.
Several cell types may contribute to the spinal sensitization. First, the sensitization of peripheral nociceptors increases the sensory input into the spinal cord, thus providing a stronger presynaptic component of synaptic activation. Second, postsynaptic spinal cord neurons are rendered hyperexcitable by the activation of NMDA and other receptors (Sandkühler 2000; Woolf and Salter 2000). Third, glial cells may be activated and produce cytokines and other mediators which facilitate the spinal processing. Glial cells are strongly activated in neuropathic pain states, but they may also contribute to inflammatory pain (McMahon and Malcangio 2009). The involvement of glial cells in pain states is addressed by Old et al. (2015). Fourth, the activity of inhibitory interneurons may be reduced. The inhibitory neurons in the spinal cord and the mechanisms by which the inhibitory control is decreased or lost are addressed by Todd (2015). The spinal sensitization and the resulting thalamocortical processing are thought to underlie the observation that in many pain states the pain becomes widespread (Phillips and Clauw 2013). The significance of central sensitization in humans under clinically relevant conditions, and the experimental methods to test central sensitization in humans, will be addressed by Arendt-Nielsen (2015).
Ascending nociceptive information activates the thalamocortical system. During chronic pain states significant changes of this system were observed in patients using fMRI. Remarkably, many chronic pain states such as chronic osteoarthritic pain are associated with a so-called atrophy of the regions in which pain is processed. The underlying cellular mechanisms have not been identified. Interestingly, this atrophy seems to be reversible because after successful treatment of pain, the brain structures show a normalization (Bushnell et al. 2013; Gwilym et al. 2010; Rodriguez-Raecke et al. 2009). Under neuropathic conditions the cortex may show a reorganization with significant changes in the cortical maps. Such changes were, e.g., observed during phantom limb pain.
As already mentioned, ascending tracts not only activate the thalamocortical system. They also activate the amygdala via the parabrachial nucleus. Further input to the amygdala is provided by the nerve fibers from the thalamus and from the cortex (Duvarci and Pare 2014). The amygdala is key nuclei in the generation of fear, and they can be activated in pain conditions (Kulkarni et al. 2007). In this volume, the role of the amygdala and their connections to the medial prefrontal cortex (mPFC) in pain states will be addressed by Neugebauer (2015). Pain-related mPFC deactivation results in cognitive deficits and the failure of inhibitory control of amygdala processing. Impaired cortical control allows the uncontrolled persistence of amygdala pain mechanisms.
Neural pathways descending from the brain stem mediate inhibition and facilitation of nociceptive spinal cord neurons (Ossipov et al. 2010; Vanegas and Schaible 2004). During severe chronic pain, a reduction of descending inhibition, in particular the diffuse inhibitory noxious control (DNIC), was reported (Kosek and Ordeberg 2000; Lewis et al. 2012). In addition, descending facilitation may contribute to pain, in particular during neuropathic pain (Vanegas and Schaible 2004). Thus, descending inhibitory systems from the brain stem may be less effective and/or descending excitatory systems from the brain stem may be overactive during chronic pain. These changes may be (partly) reversible after successful pain treatment (Kosek and Ordeberg 2000).
Effects of the nervous system on inflammation. It must be noted that the importance of the nociceptive nervous system extends beyond the generation of pain. The nervous system is able to influence inflammatory processes in the organs. Such influences are mediated by the efferent effects of nociceptive sensory afferents which produce neurogenic inflammation, by fibers of the sympathetic and parasympathetic nervous system, and by neuroendocrine influences (Schaible and Straub 2014). Spinal hyperexcitability is not only important for pain generation (see above). It plays also a role in the regulation of joint inflammation (Waldburger and Firestein 2010). In this volume this topic will be addressed by Sorkin (2015). Both pro- and anti-inflammatory feedback loops can involve just the peripheral nerves and the spinal cord or can also include more complex, supraspinal structures such as the vagal nuclei and the hypothalamic–pituitary axis.
1.4 Molecular Mechanisms of Pain
Molecular mechanisms of nociception are of considerable interest for pharmacologic approaches, and therefore, they are particularly addressed in this volume. The peripheral nociceptor as well as the spinal cord and the amygdala are in the focus.
Nociception in the periphery consists of two elementary processes, the transduction of stimuli (the generation of a sensor potential by the impact of a noxious stimulus) and the transformation of the sensor potential into a series of action potentials. Noxious stimuli are mechanical or thermal (heat and cold), and also some chemical mediators (e.g., bradykinin or H+) cause pain. The chemosensitivity of nociceptors is particularly important for the process of sensitization (and priming).
For the transduction of thermal stimuli into sensor potentials, ion channels of the transient receptor potential (TRP) family are responsible. While the involvement of TRPV1, TRPV2, and TRPM8 in the sensation of noxious heat (TRPV1 and TRPV2) and innocuous cold (TRPM8) has been established, the significance of other TRP channels in thermo(noci)ception is not that clear. For two TRP channels (TRPA1 and TRPV4), a role in mechanical hyperalgesia is being discussed (Kwan et al. 2009; Levine and Alessandri-Haber 2007; Malsch et al. 2014; Segond von Banchet et al. 2013) although these channels may not be the transduction molecules involved in the “normal mechanonociception.” The current knowledge on the involvement of TRP ion channels in the sensation of noxious heat and noxious cold and of the involvement of these ion channels in the generation of thermal hyperalgesia has been summarized (Basbaum et al. 2009; Julius 2013; Stein et al. 2009) and is not the topic of this volume.
Some chemicals can also open ion channels. For example, H+ triggers the opening of acid-sensing ion channels (ASICs), and capsaicin opens TRPV1. Most mediators, however, activate membrane receptors and are thereby involved in the sensitization of nociceptive neurons (see below).
The sensor potential triggers the generation of action potentials. For action potentials voltage-gated sodium channels are essential. In nociceptive neurons, mainly the sodium channels Nav1.7, Nav1.8, and Nav1.9, and under neuropathic conditions Nav1.3, are expressed (Waxman and Zamponi 2014). Nav1.7 is activated by slow, subtle depolarization close to the resting potential, and it thus sets the gain on nociceptors. Nav1.8, which shows depolarized voltage dependence, produces most of the current responsible for the action potential upstroke, and it supports repetitive firing. Nav1.9 does not contribute to the action potential upstroke but depolarizes the cells and prolongs and enhances small depolarization thus enhancing excitability (Waxman and Zamponi 2014). In this volume Habib et al. (2015) will address the role of these ion channels in different inflammatory and neuropathic pain states. They show that particular Nav ion channels are involved in different pathophysiologic states. Because Na+ channel blockers are thought to be promising targets for new analgesics (Gold 2008), such knowledge is important for the understanding of which blocker might be suitable under the particular conditions.
When neurons are sensitized both the channels of transduction and the voltage-gated ion channels, in particular the Na+ channels, show changes such that the excitability is enhanced (Linley et al. 2010; Schaible et al. 2011). Some mediators such as prostaglandin E2 change the opening properties of TRPV1 and of sodium channels such that weaker stimuli are sufficient to open the ion channels. The effect of prostaglandin E2 is mediated by G protein-coupled receptors which activate second messengers in the nociceptors (Hucho and Levine 2007), and these second messenger systems change the opening properties of the ion channels.
While prostaglandins are known for a long time as sensitizing molecules, more recent research revealed a number of other receptor types in nociceptive sensory neurons which are of great importance for the sensitization. It was shown that proinflammatory cytokines such as TNF-α, interleukin-6, and interleukin-17 induce a persistent state of sensitization in C-fibers (Brenn et al. 2007; Richter et al. 2010, 2012). Cytokines are thought to play a significant role in the generation of inflammatory and neuropathic role (Schaible et al. 2010; Sommer and Kress 2004; Üceyler et al. 2009). Interleukin-6 is thought to be an important molecule of hyperalgesic priming (see Kandasamy and Price 2015).
NGF and its receptor trkA were discovered as suitable targets for pain treatment. A single application of an antibody to NGF was shown to provide significant pain relief in osteoarthritis for several weeks (Lane et al. 2010). NGF has a variety of actions on nonneuronal cells and sensory neurons which regulate the excitability in the long term (Bennett 2007). In this volume Mizumura and Murase (2015) address the hyperalgesic effects of NGF in different tissues and in inflammatory and neuropathic pain states, and they address the mechanisms involved.
Proteinase-activated receptors (PARs) are a family of G protein-coupled receptor that is activated by extracellular cleavage of the receptor in the N-terminal domain. This slicing of the receptor exposes a tethered ligand which binds to a specific docking point on the receptor surface to initiate intracellular signaling. McDougall and Muley summarize how serine proteinases activate PARs leading to the development of pain in several chronic pain conditions. The potential of PARs as a drug target for pain relief is discussed (McDougall and Muley 2015).
Excitatory synaptic transmission in the spinal cord under basal conditions is mediated by the transmitter glutamate, the transmitter of nociceptive sensory neurons. Central sensitization is also dependent on glutamate, in particular acting on NMDA receptors. However, numerous other transmitters and mediators are involved in the complex signaling in the spinal cord (e.g., NK1 receptors for substance and CGRP receptors) (Woolf and Salter 2000). Other mediators such as spinal prostaglandins contribute to spinal sensitization (Bär et al. 2004). The particular role of NO to nociceptive spinal cord signaling will be addressed by Schmidtko (2015). The role of mediators involved in glial cell activation and functions will be addressed by Old et al. (2015).
Under normal conditions, excitatory and inhibitory synaptic mechanisms are presumably in a balanced activity state. Such inhibition is provided by specific local inhibitory interneurons (see Todd 2015), but it may also be provided by mediators which act in a feedback manner from activated neurons. Such inhibitory control is, e.g., provided by endocannabinoids which are addressed in this volume by Woodhams et al. (2015). Cannabinoid 1 (CB1) receptors are found at presynaptic sites throughout the peripheral and central nervous systems, while the CB2 receptor is found principally (but not exclusively) on immune cells. The endocannabinoid (EC) system is now known to be one of the key endogenous systems regulating pain sensation, with modulatory actions at all stages of pain processing pathways. As already discussed, pain states may involve a reduction of inhibitory mechanisms.

Full access? Get Clinical Tree
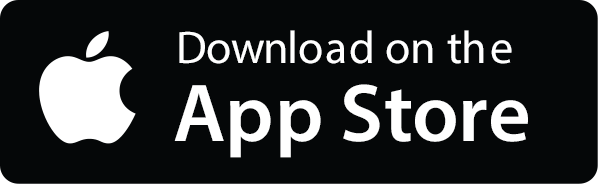
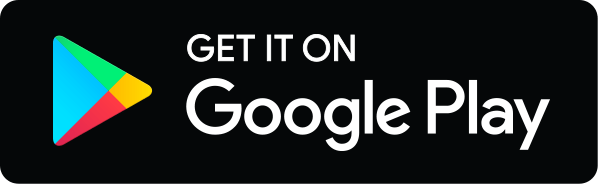