Mechanical—acute
Laceration
Stretch
Intraneural injection
Vascular
Acute ischemia
Hemorrhage
Pressure
Extraneural
Intraneural
Compartment syndrome
Chemical
Injection of neurotoxic solutions
Severity of Acute Nerve Injuries
Classification of acute nerve injuries is useful when considering the physical and functional state of damaged nerves. In his classification, Seddon introduced the terms neurapraxia, axonotmesis, and neurotmesis (Table 5.2).
Table 5.2
Classification systems for nerve injury
Classification | Pathology | Prognosis |
---|---|---|
Neurapraxia | Myelin injury or ischemia | Excellent recovery in weeks to months |
Axonotmesis | Axon loss Variable stromal disruption | Good to poor Depending upon the integrity of supporting structures and distance to muscle |
Endoneurial tubes intact Perineurium intact Epineurium intact | Good Depending upon distance to muscle | |
Endoneurial tubes disrupted Perineurium intact Epineurium intact | Poor Axonal misdirection Surgery may be required | |
Endoneurial tubes disrupted Perineurium disrupted Epineurium intact | Poor Axonal misdirection Surgery usually required | |
Neurotmesis | Axon loss Endoneurial tubes severed Perineurium severed Epineurium severed | No spontaneous recovery Surgery required Prognosis after surgery guarded |
Neurapraxia refers to nerve dysfunction lasting several hours to 6 months after a blunt injury to the nerve. In neurapraxia, the nerve axons and connective tissue structures remain intact. The nerve dysfunction probably results from several factors, of which focal demyelination is the most important abnormality. Intraneural hemorrhage, changes in the vasa nervora, disruption of the blood–nerve barrier and axon membranes, and electrolyte disturbances all may add to the impairment of nerve function. Because the nerve dysfunction is rarely complete, clinical deficits are partial and recovery usually occurs within 6–8 weeks, although some neurapraxic lesions (with minimal or no axonal degeneration) may take several months to recover. Axonotmesis consists of physical interruption of the axons but within intact Schwann cell tubes and intact connective tissue structures of the nerve (i.e., the endoneurium, perineurium, and epineurium). Sunderland subdivided this group, depending on which of the three structures were involved. With axonotmesis, the nerve sheath remains intact, enabling regenerating nerve fibers to find their way into the distal segment. Consequently, efficient axonal regeneration can take 2–18 months. Neurotmesis refers to a complete interruption of the entire nerve including the axons and all connective tissue structures (epineurium included). Clinically, there is total nerve dysfunction. With both axonotmesis and neurotmesis, axonal disruption leads to Wallerian degeneration, from which recovery occurs through the slow process of axonal regeneration. However, with neurotmesis, the two nerve ends may be completely separated, and the regenerating axons may not be able to find the distal stump. For these reasons, effective recovery does not occur unless the severed ends are sutured or joined by a nerve graft.
Mechanical Nerve Injury
Intraneural Injection
Intraneural injection has the potential to create structural damage to the fascicle(s) that is more extensive and less likely to heal as compared to a relatively clear injury caused by a needle. Indeed, the devastating sequelae of sensory and motor loss after injection of various agents into peripheral nerves has been well documented [5, 6]. Nearly all experimental studies on this subject have demonstrated that the site of injection is critical in determining the degree and nature of injury. In order for peripheral nerve injury to occur with an intraneural injection, local anesthetic probably need be injected intrafascicularly; even intentionally, intraneural injections may not invariably lead to neurologic injury [7].
In clinical practice of peripheral nerve blockade (PNB), injection of local anesthetic is typically followed by a latency of 10–30 min for the blockade to develop. In contrast, injections of the same LA for the same PNBs occasionally result in nearly instantaneous, dense, and unusually long-lasting nerve blockade. It is almost certain that such blocks are the result of intraneural injections and the consequent intimate exposure of neural tissue to even low concentration and small volume of local anesthetics [7, 8]. However, intraneural injections can be extrafascicular or intrafascicular. The intraneural–extrafascicular injections are characterized by a diffuse spread of the injectate within the epineurium with escape of the fluid into the extraneural space (Figs. 5.1 and 5.2). Such injections do not necessarily result in nerve injury [5–10]. In contrast, intrafascicular injections almost invariably lead to some degree of neurologic impairment [5] and possibly a substantial proximal spread of the injectate toward the neuraxis [11, 12].
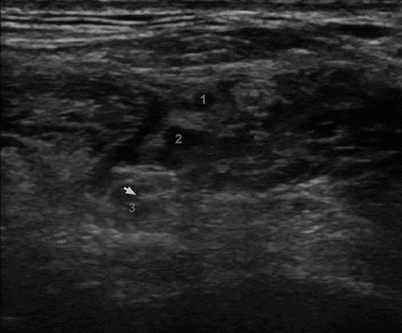
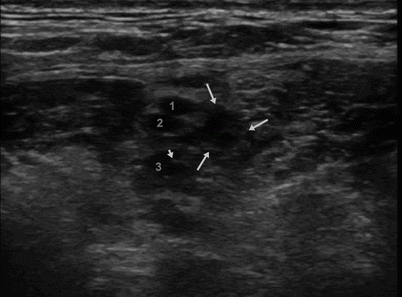
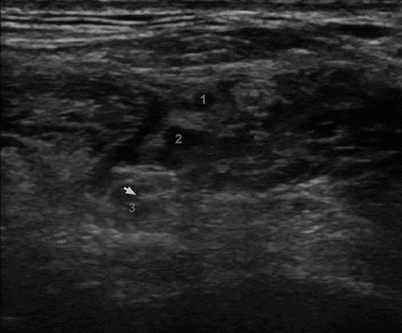
Fig. 5.1
Ultrasonographic image of the brachial plexus during interscalene blockade. Shown are the superior (1), middle (2), and inferior (3) cords of the brachial plexus. The off-line review of the image demonstrated needle insertion in the inferior cord of the brachial plexus (arrowhead)
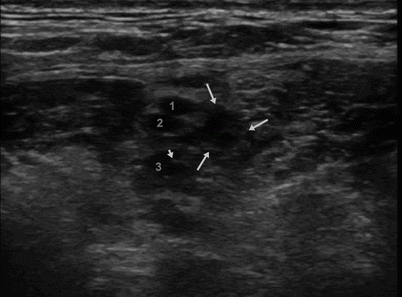
Fig. 5.2
Inadvertent intraneural injection. Shown are the superior (1), middle (2), and inferior (3) cords of the brachial plexus. The tip of the needle is seen within the inferior cord of the brachial plexus (arrowhead), while the injection of local anesthetic resulted in escape of the local anesthetic into the interscalene groove (arrows)
Histologic features of injury after intraneural injection are rather nonspecific and range from simple mechanical disruption and delamination to fragmentation of the myelin sheath and marked cellular infiltration (Fig. 5.3). Using a variety of animal models of nerve injury, a vast array of cellular changes following peripheral nerve trauma have been documented [8]. The extent of actual neurological damage after an intrafascicular injection can range from neurapraxia with minimal structural damage to neurotmesis with severe axonal and myelin degeneration, depending upon the needle–nerve relationship, agent injected, and dose of the drug. Injury to primary sensory neurons may cause a shift in membrane channel expression, sensitivity to algogenic substances, neuropeptide production, and intracellular signal transduction, both at the injury site and in the cell body in the dorsal root ganglion. These events may lead to increased excitability and the occurrence of acute or chronic pain or dysesthesia often experienced by patients with neurologic injury.
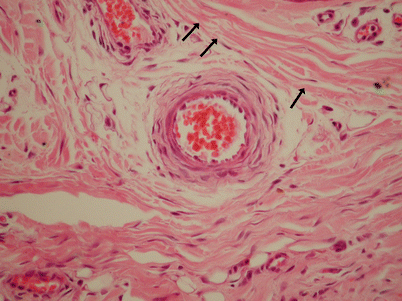
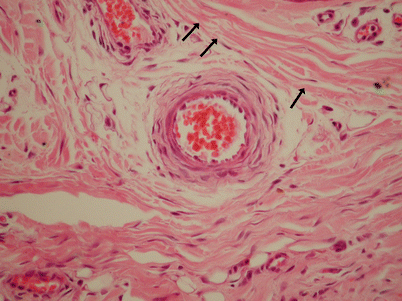
Fig. 5.3
Pathohistologic changes in the sciatic nerve of the dog after an intraneural injection of 2 % lidocaine. Shown are perineurial delamination (arrows) and fascicular and epineurial cellular (inflammatory) infiltration
Signs, Symptoms, and Methods to Reduce the Risk of Intraneural Injection
Our ability to monitor and avoid intrafascicular injection during PNBs has been limited. The discussion below focuses on methods commonly used in clinical practice to reduce the risk of intraneural injection.
Pain on Injection
Pain with injection has long been thought of as the cardinal sign of intraneuronal injection; consequently, it is commonly suggested that blocks be avoided in heavily premedicated or anesthetized patients. However, numerous case reports have suggested that pain may not be reliable as a sole warning sign of impending nerve injury, and it may present in only a minority of cases [13]. Fanelli and colleagues have reported unintended paresthesia in 14 % of patients in their study; however, univariate analysis of potential risk factors for postoperative neurologic dysfunction failed to demonstrate paresthesia as a risk factor. In addition, the sensory nature of the pain or paresthesia can be difficult to interpret in clinical practice. For instance, a certain degree of discomfort on injection (“pressure paresthesia”) is considered normal and affirmative of impending successful blockade because it is thought that this symptom indicates that injection of local anesthetic has been made in the vicinity of the targeted nerve [14]. In clinical practice, however, it can be difficult to discern when pain–paresthesia on injection is “normal” and when it is the ominous sign of an intraneural injection. Moreover, it is unclear how pain or paresthesia on injection, even when present, can be used clinically to prevent the development of neurologic injury. For instance, in a prospective study on neurological complications of regional anesthesia by Auroy and colleagues, although the participating anesthesiologists did not continue to inject local anesthetic when pain on injection occurred, neurologic injuries after paresthesia still ensued [1].
Minimal Intensity of the Stimulating Current
The optimal current intensity resulting in accurate localization of a nerve has been a topic of controversy. Methods in most recently published reports have reported obtaining nerve stimulation with currents of 0.2–0.5 mA (100 μs) prior to injecting local anesthetics, believing that motor response with current intensities lower than 0.2 mA may be associated with intraneural needle placement. A recent study in an animal model suggested that the ability to obtain motor response to nerve stimulation using current intensity of <0.2 mA may result in an intraneural injection and inflammation of the nerve [15].
Paresthesia Versus Nerve Stimulation
While it is clear that needle trauma can result in nerve damage, it is uncertain whether block techniques that seek to elicit mechanical contact paresthesias during block needle insertion increase the risk of lasting injury. One study demonstrates that seeking paresthesias may increase postoperative lesions [16], but a contrasting study [17] shows only a 0.36 % rate of neuropathy from brachial plexus blocks done with intentional production of paresthesias. It is unresolved whether using electrical stimulation through the needle reduces the incidence of nerve damage. However, advancement of a needle beyond the depth that produces a motor response by current stimulation will typically cause a mechanical paresthesia by contact [18], indicating that electrical nerve localization occurs at a somewhat greater distance than mechanical paresthesia [19]. Nonetheless, the stimulator technique cannot guarantee safety, since it has been shown that the needle may enter the nerve without producing a detectable motor response [1, 20, 21].
Ultrasound-Guided Nerve Blocks
Real-time monitoring of needle placement by US is useful, but of inadequate resolution to avoid intrafascicular injection [10]. During ultrasound-guided nerve blocks, some clinicians first inject a small volume (2–3 ml) of local anesthetic as a precautionary measure to avoid an intrafascicular injection. However, fascicles are small structures, and injury may occur even with minute volumes of LA (≤0.5 mL) [5, 8, 11, 22]. Injections into fascicles are characterized by high opening injection pressure (≥20 psi), followed by a rapid decrease of injection pressure to normal as the perineurium ruptures and local anesthetic leaks out perineurally (Fig. 5.4) [8, 11, 22]. Therefore, intraneural injection of even small volumes of local anesthetic may be hazardous. For success and safety of PNBs, a combination of real-time US needle guidance along with in-line injection pressure monitoring [23] and avoidance of injection with stimulation of <0.2 mA [15] may prove to be the ultimate monitoring during PNBs (Fig. 5.5) [8, 23, 24].
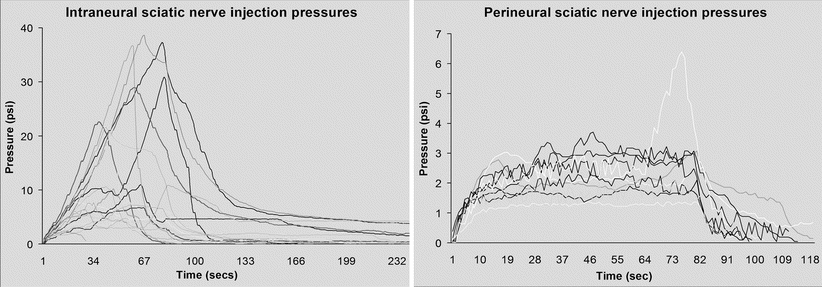
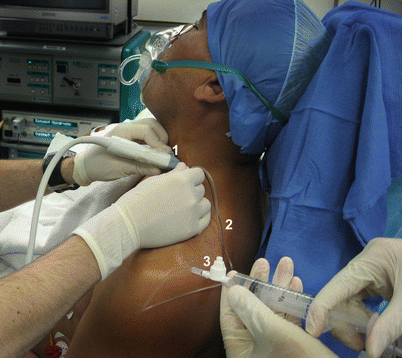
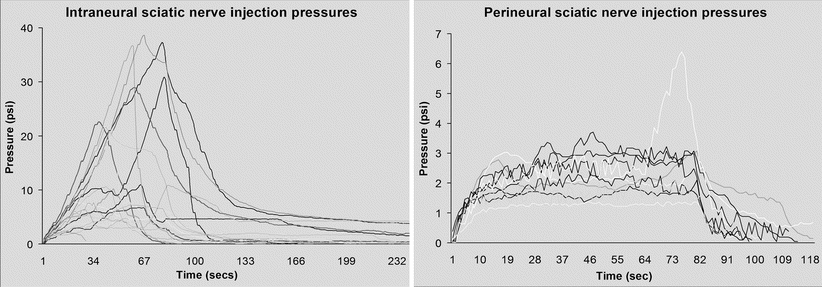
Fig. 5.4
Injection pressure during intraneural (intrafascicular) and perineural application of local anesthetics.Intraneural injection of local anesthetic results in significantly higher injection pressures (Kapur E et al. [8]. With permission of Blackwell publishing)
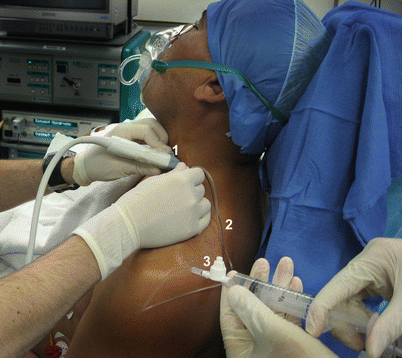
Fig. 5.5
Administration of interscalene brachial plexus block with ultrasound guidance (1), electrical nerve stimulation (2), and in-line injection pressure monitoring (3) to avoid injection pressure >20 psi which may be indicative of an intraneural intrafascicular injection
Needle Design and Direct Needle Trauma
Needle tip design and risk of neurologic injury have been matters of considerable debate. Nearly 30 years ago, Selander and colleagues suggested that the risk of perforating a nerve fascicle was significantly lower when a short-bevel (e.g., 45°) needle was used instead of a long-bevel (12–15°) needle [25]. The result of their work is largely responsible for the current trend of using short-bevel needles (i.e., angles 30–45°) for the majority of major peripheral nerve conduction blocks. However, the more recent work of Rice and McMahon suggested that when placed intraneurally, short-beveled needles cause more mechanical damage than the long-beveled needles [26]. In a rat model, where deliberate penetration of the largest fascicle of the sciatic nerve with 12- to 27°-beveled needles was studied, short-beveled needles resulted in the greatest degree of neural trauma. Their work suggests that sharp needles produce clean, more-likely-to-heal cuts, whereas blunt needles produced noncongruent cuts and more extensive damage on microscopic images. In addition, the cuts produced by the sharper needles were more likely to recover faster and more completely than were the irregular; more traumatic injuries caused by the blunter, short-beveled needles. Although the data on needle design and nerve injury have not been clinically substantiated, the theoretical advantage of short-beveled needles in reducing the risk of nerve penetration has influenced both practitioners and needle manufacturers. Consequently, whenever practical, most clinicians today prefer to use short-beveled needles for major conduction blocks of the peripheral nerves and plexuses. Long-bevel, small-gauge needles, however, continue to be used routinely for many nerve block procedures, such as axillary transarterial brachial plexus block, wrist and ankle blocks, cutaneous nerve block, and others.
Toxicity of Injected Solutions
Local anesthetics produce a variety of cytotoxic effects in cell cultures, including inhibition of cell growth, motility, and survival, and they may also produce morphologic changes [27]. The extent of these effects is proportionate to the duration of exposure to the local anesthetic solution, and they can occur using local anesthetic concentrations in the range used clinically. Within this range, the cytotoxic changes are greater as concentrations increase. Relevant to the clinical setting, the exact site of the local anesthetic deposition plays a critical role in determining the pathogenic potential. After application of local anesthetics outside a fascicle, the regulatory function of the perineurial and endothelial blood–nerve barrier is only minimally compromised. The normally hypertonic endoneural fluid becomes hypotonic, with the accumulation of edema, increased perineural permeability, and increased fluid pressures within the fascicles [28]. Inflammatory changes, as well as myelin and Schwann cell injury, have been identified [28–30].
High concentrations of extrafascicular anesthetics produce axonal injury independent of edema formation and elevated endoneurial fluid pressure [31]. The duration of exposure and concentration of local anesthetic determine the degree and incidence of local anesthetic-induced residual paralysis [32–34]. The importance of these changes after extrafascicular injections in contributing to clinical cases of nerve injury has not been determined, but it is prudent to use the minimum necessary local anesthetic concentrations. Since small-fiber neurons are more sensitive to chemical damage, the manifestations of local anesthetic nerve damage may include spontaneous paresthesias and deficits in pain and temperature perception but not loss of motor, touch, or proprioceptive function [35].
Injection of local anesthetic within a nerve fascicle has been invariably neurotoxic in numerous animal models [5]. Intrafascicular injection of saline alone, lidocaine 1 %, and bupivacaine 0.5 % results in evidence of axonal degeneration and barrier changes. Findings are progressively worse with increasing concentrations of both agents, especially in concentrations above the clinically used range [36, 37].
In addition to local anesthetics themselves, various adjuvant agents injected together with the local anesthetics for neural blockade may play a role in causing nerve damage. Epinephrine amplifies the vasocompression effects of local anesthetics [38]. The addition of epinephrine has been shown to increase the neurotoxicity of bisulfite-containing chloroprocaine solutions [29] and to increase the axonal degeneration that follows intrafascicular bupivacaine injection [36, 37]. Nerve blood flow, especially in the large epineural feeding arteries, is sensitive to the vasoconstrictive effect of injected epinephrine at usual concentrations [39]. Regardless of these laboratory findings, however, the clinical use of epinephrine is common, and its potential to cause clinical neurotoxicity remains controversial.
Nerve Ischemia
The earliest response of the peripheral sensory neuron to ischemia is depolarization and generation of spontaneous activity, perceived by the subject as paresthesias. This is followed by blockade of slow-conducting myelinated fibers and eventually all neurons [21], possibly through accumulation of excess intracellular calcium [40], which accounts for the loss of sensation with initiation of limb ischemia.
Nerve function returns within six hours if ischemic times are less than two hours [41], and ischemic periods of up to 6 h may fail to produce permanent structural changes in the nerves [42]. However, more detailed pathological examination after 3 h of reperfusion can show edema and fiber degeneration that lasts for 1–2 weeks, followed by a phase of regeneration lasting 6 weeks [43]. In addition to neuronal damage, oxidative injury associated with ischemia and reperfusion also affects the Schwann cells, initiating apoptosis [44]. Recently, sensory testing of rats 2–4 h after a 3-h period of hind paw ischemia demonstrated hypersensitivity to cold and innocuous or nociceptive mechanical stimuli reminiscent of human hyperalgesic syndromes [45].
Tourniquets may cause nerve damage either by ischemia or mechanical deformation. The initial effect of direct compression of the nerve by the tourniquet is failure of transmission by fast conducting myelinated fibers [46]. Prolonged nerve dysfunction results from damage to the portion of the nerve under the edge of the pneumatic cuff, where the mechanical distortion of the nerve is maximal. Irreversible damage, including substantial distortion of myelin lamellae and axonal shrinkage, may ensue as early as 2–4 h after tourniquet inflation [47] and predominantly affects large diameter neurons [48]. Thus, the main findings of tourniquet-induced neuropathy are motor loss and diminished touch, vibration, and position sense, with preserved senses of heat, cold, and pain and the absence of spontaneous paresthesias [49]. One may minimize nerve damage by using wide cuffs and inflation pressures just adequate for arterial occlusion [50], but periodic deflation of the cuff (even as much as 10 min down every hour) has no beneficial effects on the compression trauma [51]. Alternating between the two cuffs of a double-cuff tourniquet may allow prolonged blood flow interruption with diminished mechanical damage to the nerves, since each site is compressed for only half the total duration [52].

Full access? Get Clinical Tree
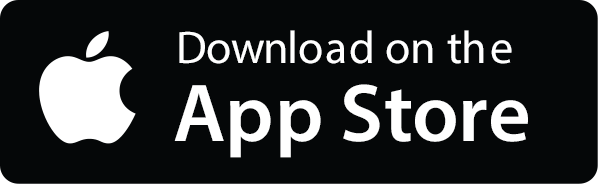
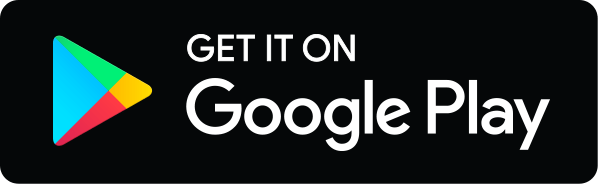