Complex Regional Pain Syndrome, Including Applications of Neural Blockade
Andreas Binder
Ralf Baron
The term complex regional pain syndrome (CRPS) describes a variety of painful conditions following injury that appears regionally with a distal predominance of abnormal findings. The symptoms exceed in both magnitude and duration the expected clinical course of the inciting event and often result in significant impairment of motor function. The disorder shows a variable progression over time. These chronic pain syndromes comprise different additional clinical features including spontaneous pain, allodynia, hyperalgesia, edema, autonomic abnormalities, and trophic signs. In CRPS type I (reflex sympathetic dystrophy) minor injuries or limb fractures precede the onset of symptoms; CRPS type II (causalgia) develops after injury to a major peripheral nerve (1,2,3).
Complex Regional Pain Syndrome Type I (Reflex Sympathetic Dystrophy)
The most common precipitating event is a trauma affecting the distal part of an extremity (65%), especially fractures, postsurgical conditions, contusions, and strain or sprain. Less common occasions are central nervous system (CNS) lesions, such as spinal cord injuries and cerebrovascular accidents, as well as cardiac ischemia (4).
CRPS I patients develop asymmetrical distal extremity pain and edema without presenting an overt nerve lesion (Fig. 46-1). These patients often report a burning, spontaneous pain felt in the distal part of the affected extremity. Characteristically, the pain is disproportionate in intensity to the inciting event. The pain usually increases when the extremity is in a dependent position. Stimulus-evoked pains are a striking clinical feature. These sensory abnormalities often appear early, are most pronounced distally, and have no consistent spatial relationship to individual nerve territories or to the site of the inciting lesion. Typically pain can be elicited by movement of and pressure on the joints (deep somatic allodynia), even if these are not directly affected by the inciting lesion.
Autonomic abnormalities include swelling and changes in sweating and skin blood flow. In the acute stages of CRPS I, the affected limb is often warmer than the contralateral limb (5,6). In chronic phases, vasoconstriction and cold skin is induced by endothelial damage (7). Sweating abnormalities, either hypohidrosis or, more frequently, hyperhidrosis, are present in nearly all CRPS I patients. The acute distal swelling of the affected limb depends very critically on aggravating stimuli. Since it diminishes after sympathetic blocks, it is likely that this swelling is maintained by sympathetic activity.
Trophic changes, such as abnormal nail growth, increased or decreased hair growth, fibrosis, thin glossy skin, and osteoporosis may be present, particularly in chronic stages. Restrictions of passive movement are often present in long-standing cases and may be related to both functional motor disturbances and trophic changes of joints and tendons.
Weakness of all muscles of the affected distal extremity is often present. Small, accurate movements are characteristically impaired. Nerve conduction and electromyography studies are normal, except in patients in very chronic and advanced stages. About half of the patients have a postural or action tremor representing an increased physiologic tremor. In about 10% of cases, dystonia of the affected hand or foot develops.
Complex Regional Pain Syndrome Type II (Causalgia)
The symptoms of CRPS II are similar to those of CRPS I. The only exception is that a lesion of peripheral nerve structures and subsequent focal deficits are mandatory for the diagnosis. The symptoms and signs spread beyond the innervation territory of the injured peripheral nerve and often occur remote from the site of injury, although a restriction to the territory is not in conflict with the current definition.
Epidemiology
A population-based study in the United States on CRPS I calculated an incidence of about 5.5 per 100,000 person years at-risk and a prevalence of about 21 per 100,000 (8). An incidence of 0.8 per 100,000 person years at-risk and a prevalence of about 4 per 100,000 was reported for CRPS II. In contrast, a European population-based study determined a much higher incidence of 26.2 for CRPS in general when using a different diagnostic approach (9). However, CRPS I develops more often than CRPS II. The incidence of CRPS II in peripheral nerve injury varies from 2% to 14% in different series, with a mean of around 4% (10). Estimations suggest an incidence of CRPS I of 1% to 2% after fractures, 12% after brain lesions, and 5% after myocardial infarction. However, the latter data for brain lesions and myocardial infarctions are relatively high and must be interpreted with some caution because of the lack of
uniform diagnostic criteria. Females are more often affected than males, with a female-to-male ratio ranging from 2:1 to 4:1. CRPS shows a distribution over all ages, with a mean age peak at diagnosis of 40 to 50 years and highest incidence rates at 61 to 70 years. Differences in ethnicity, socioeconomic status, and different diagnostic criteria used may contribute to epidemiologic differences (9).
uniform diagnostic criteria. Females are more often affected than males, with a female-to-male ratio ranging from 2:1 to 4:1. CRPS shows a distribution over all ages, with a mean age peak at diagnosis of 40 to 50 years and highest incidence rates at 61 to 70 years. Differences in ethnicity, socioeconomic status, and different diagnostic criteria used may contribute to epidemiologic differences (9).
![]() Figure 46-1. Clinical picture of patient with complex regional pain syndrome type I of the upper left extremity following distortion of the left wrist. |
Predominantly, CRPS occurs in one extremity. Retrospective studies in large cohorts showed a distribution in the upper and lower extremity from 1:1 to 2:1. In 113 retrospectively reviewed cases, the symptoms occurred in 47% on the right, in 51% on the left side, and in 2% bilaterally. Multiple extremities were affected in up to 7% (10).
Are Stages Appropriate?
A sequential progression of untreated CRPS has been repeatedly described, each stage of which (usually three are proposed) differing in patterns of signs and symptoms. Nevertheless, this concept has come into question in the last few years. In 2002, the clinical validity of this concept was tested by Bruehl et al. in 113 patients (11). Using a cluster analysis, three subgroups were identified that could be differentiated by their symptoms and signs regardless of disease duration. The sequential concept relies on the course of untreated CRPS; however, to date, all studies performed to test its clinical validity investigated patients already under treatment. Furthermore, vascular disturbances and skin temperature measurements indicated different thermoregulatory types, depending on time.
In conclusion, it is questionable whether staging of CRPS is appropriate. It is much more practical, with direct implication to therapy, that patients with CRPS be graded according to the intensity of their sensory, autonomic, motor, and trophic changes as having mild, moderate, or severe impairment.
Psychology
Most patients with CRPS exhibit significant psychological distress, most commonly depression and anxiety. Many patients become overwhelmed by the pain and associated symptoms and, without adequate psychosocial support, may develop maladaptive coping skills. Based on these symptoms, there is a tendency to ascribe the etiology of CRPS to emotional causes, and it has been proposed that CRPS is a psychiatric illness. In fact, it is sometimes difficult to recognize the organic nature of the symptoms. However, when describing the clinical picture, Livingston was convinced: “The ultimate source of this dysfunction is not known but its organic nature is obvious and no one seems to doubt that these classical pain syndromes are real.” Covington (12) drew several conclusions on psychological factors in CRPS: (a) No evidence was found to support that CRPS is a psychogenic condition; (b) because anxiety and stress increases nociception, relaxation and antidepressive treatment is helpful; (c) the pain in CRPS is the cause of psychiatric problems, and not the converse; (d) maladaptive behavior by the patients, such as volitional or inadvertent actions, are mostly due to fear, regression, or misinformation and do not indicate psychopathology; and (e) some patients with conversion disorders and factitious diseases have been diagnosed incorrectly with CRPS. In summary, the author concludes that the widely proposed “CRPS personality” is clearly unsubstantiated. This assumption was further strengthened when no differences in psychological patterns were found in those patients with radius fracture who developed CRPS I in comparison to patients who recovered without developing CRPS (13).
According to this view, an even distribution of childhood trauma, pain intensity, and psychological distress was confirmed in patients with CRPS in comparison to patients with other neuropathic pain syndromes and chronic back pain (14). Further studies demonstrated a high psychiatric comorbidity, especially depression, anxiety, and personality disorders in CRPS patients. These findings are also present in other chronic pain patients and are more likely a result of the long and severe pain disease (15). In comparison with patients with low back pain, CRPS patients showed a higher tendency to somatization but did not show any other psychological differences (16). In 145 patients, 42% reported stressful life events in close relationship to the onset of CRPS, and 41% had a history of chronic pain before CRPS (17). Thus, stressful life events could be risk factors for the development of CRPS.
Important Issues Unique to Complex Regional Pain Syndrome
Motor Abnormalities
About 50% of the patients with CRPS show motor abnormalities (18,19). It is unlikely that these motor changes are related to a peripheral process (e.g., influence of the sympathetic nervous system on neuromuscular transmission and/or contractility of skeletal muscle). These somatomotor changes are more likely generated by central changes of activity in the motoneurons. In a recent study, the motor dysfunction in CRPS patients was analyzed using kinematic analysis and functional imaging investigations to study the cerebral representation of finger movements. First, patients were investigated in a kinematic analysis assessing possible changes of movement patterns during target reaching and grasping. Compared with controls, CRPS patients particularly showed a significant prolongation of the target phase in this paradigm. The pattern of motor
impairment was consistent with a disturbed integration of visual and proprioceptive inputs in the posterior parietal cortex. Second, functional magnetic resonance imaging (fMRI) was used to investigate cortical activations during tapping movements of the CRPS-affected hand. During finger tapping of the affected extremity, CRPS patients showed a significant reorganization of central motor circuits, with an increased activation of primary motor and supplementary motor cortices (SMA) (Fig. 46-2). Furthermore, the ipsilateral motor cortex showed a markedly increased activation. When the individual amount of motor impairment was introduced as regressor in the fMRI analysis, we were able to demonstrate that activations of the posterior parietal cortices (i.e., areas within the intraparietal sulcus), SMA, and primary motor cortex were correlated with the extent of motor dysfunction. Thus, substantial adaptive changes within the CNS may contribute to motor symptoms in CRPS (20). According to the bilateral cortical changes, the motor performance was also slightly impaired on the contralateral unaffected side (21).
impairment was consistent with a disturbed integration of visual and proprioceptive inputs in the posterior parietal cortex. Second, functional magnetic resonance imaging (fMRI) was used to investigate cortical activations during tapping movements of the CRPS-affected hand. During finger tapping of the affected extremity, CRPS patients showed a significant reorganization of central motor circuits, with an increased activation of primary motor and supplementary motor cortices (SMA) (Fig. 46-2). Furthermore, the ipsilateral motor cortex showed a markedly increased activation. When the individual amount of motor impairment was introduced as regressor in the fMRI analysis, we were able to demonstrate that activations of the posterior parietal cortices (i.e., areas within the intraparietal sulcus), SMA, and primary motor cortex were correlated with the extent of motor dysfunction. Thus, substantial adaptive changes within the CNS may contribute to motor symptoms in CRPS (20). According to the bilateral cortical changes, the motor performance was also slightly impaired on the contralateral unaffected side (21).
Moreover, a neglect-like syndrome was clinically described to be involved in the disuse of the extremity (22). Recent controlled studies also support an incongruence between central motor output and sensory input as underlying mechanism in CRPS. Using the method of mirror visual feedback, the visual input from a moving unaffected limb to the brain was able to reestablish the pain-free relationship between sensory feedback and motor execution. After 6 weeks of therapy, pain and function were improved, as compared with the control group (23,24). Furthermore, a sustained inhibition of the motor cortex was found in CRPS patients on the contralateral as well as ipsilateral hemisphere (25,26).
Immune Cell–mediated Inflammation and Cytokine Release
Several studies in CRPS patients address the question to what extent an immune cell–mediated inflammation is involved
(Fig. 46-3). Skin biopsies in these patients showed a striking increase in the number of Langerhans cells, which can release immune cell chemoattractants and proinflammatory cytokines (27). In accordance, in the fluid of artificially produced skin blisters, significantly higher levels of interleukin (IL)-6 and tumor necrosis factor (TNF)-α, as well as tryptase (a measure of mast cell activity), were observed in the involved extremity as compared with the uninvolved extremity (28,29). In CRPS patients with hyperalgesia, higher levels of the soluble TNFα receptor type I were found (30). Accordingly, significant increases in IL-1β and IL-6, but not TNF-α, were demonstrated in the cerebrospinal fluid of individuals afflicted with CRPS as compared with controls (31).
(Fig. 46-3). Skin biopsies in these patients showed a striking increase in the number of Langerhans cells, which can release immune cell chemoattractants and proinflammatory cytokines (27). In accordance, in the fluid of artificially produced skin blisters, significantly higher levels of interleukin (IL)-6 and tumor necrosis factor (TNF)-α, as well as tryptase (a measure of mast cell activity), were observed in the involved extremity as compared with the uninvolved extremity (28,29). In CRPS patients with hyperalgesia, higher levels of the soluble TNFα receptor type I were found (30). Accordingly, significant increases in IL-1β and IL-6, but not TNF-α, were demonstrated in the cerebrospinal fluid of individuals afflicted with CRPS as compared with controls (31).
In this context, it is of particular interest that cutaneous denervation can cause rapid activation and proliferation of Langerhans cells that continues until reinnervation occurs. Thus, one might speculate that such cell proliferation may reflect also a functional denervation of cutaneous sympathetic outflow that occurs in CRPS (5,32).
The patchy osteoporosis that is found in more advanced CRPS cases may also be consistent with a regional inflammatory process in deep somatic tissues. Both, IL-1 and IL-6 cause proliferation and activation of osteoclasts and suppress the activity of osteoblasts.
Changes in hair growth can also be created by proinflammatory cytokines. TNF and IL-1 directly inhibit hair growth. Keratocyte-derived TNF and IL-6 cause retarded hair growth, signs of fibrosis, and, in turn, immune infiltration of the dermis, all present in CRPS patients.
Autoimmune Etiology
Several studies focus on an autoimmune response in patients with CRPS. Autoantibodies against rat sympathetic neurons were detected in 40% of CRPS patients, whereas only 5% of other neuropathy patients showed these autoantibodies (33,34). Immunoreactivity to Campylobacter was found in many early CRPS patients, in particular associated with minimal trauma, thus indicating a postinfectious autoimmune basis in some patients (35).
Sensitization to Adrenergic Stimuli: Sympathetically Maintained Pain
Sympathetically Maintained Pain
On the basis of experience and clinical studies, the term sympathetically maintained pain (SMP) was redefined: Neuropathic pain patients presenting with similar clinical signs and symptoms can clearly be divided into two groups by the negative or positive effect of selective sympathetic blockade or blockade of α-adrenoceptors (36,37). The pain component that is relieved by specific sympatholytic procedures is considered “sympathetically maintained pain.” Thus, SMP is now defined to be a symptom in a subset of patients with neuropathic disorders and not a clinical entity. The positive or negative effect of a sympathetic blockade is not essential for the diagnosis of CRPS I. On the other hand, the only possibility to differentiate between SMP and sympathetically independent pain (SIP) is the efficacy of a correctly applied sympatholytic intervention (38,39).
Influence of Sympathetic Activity and Catecholamines on Primary Afferents
Clinical studies in humans support the idea that cutaneous nociceptors develop catecholamine sensitivity after partial nerve lesions (CRPS II). Intracutaneous application of norepinephrine into a symptomatic area rekindles spontaneous pain and dynamic mechanical hyperalgesia/allodynia that had been relieved by sympathetic blockade (40,41). Intracutaneous injection of norepinephrine in control subjects does not elicit pain.
The question arises whether, in CRPS I, similar mechanisms of SMP exist, although no major nerve lesion is present. To address this issue, we performed a study in patients with CRPS I using physiologic stimuli to excite sympathetic neurons (42). Cutaneous sympathetic vasoconstrictor outflow to the painful extremity was experimentally activated to the highest possible physiologic degree by whole-body cooling. During the thermal challenge, the affected extremity was clamped to 35°C to avoid thermal effects at the nociceptor level. The intensity of spontaneous pain and mechanical hyperalgesia (dynamic and punctate) increased significantly in patients who had been classified as having SMP by positive sympathetic blocks but not in SIP patients (Fig. 46-4).
The experimental intervention used in the present study selectively alters sympathetic cutaneous vasoconstrictor activity without influencing other sympathetic systems innervating the deep somatic tissues (e.g., muscle vasoconstrictor neurons [43,44]). Therefore, the interaction of sympathetic and afferent neurons measured here is likely to be located within the skin, as predicted by the pain-enhancing effect of intracutaneous norepinephrine injections in CRPS II. Interestingly, the relief of spontaneous and evoked pain after sympathetic blockade was more pronounced than changes in spontaneous and evoked pain that could be induced experimentally by sympathetic activation. One explanation for this discrepancy might be that a complete sympathetic block affects all sympathetic outflow channels projecting to the affected extremity. It is very likely that, in addition to a coupling in the skin, a sympathetic–afferent interaction may also occur in other tissues, in particular in the deep somatic domain such as bone, muscle, or joint. Supporting this view, these structures in particular are extremely painful in some patients with CRPS (45). Furthermore, patients may exist who are characterized by a selective or predominant sympathetic–afferent interaction in deep somatic tissues, sparing the skin (32) (Fig. 46-5).
Mechanisms Involved in Sympathetically Maintained Pain
The quantitative measurements shown in Figure 46-6 on patients with CRPS I and with SMP clearly demonstrate that the underlying mechanism of SMP must be a coupling between sympathetic noradrenergic neurons and primary afferent neurons in the periphery of the body. The mechanisms of these couplings are not revealed by the experiments performed on human patients. However, it must be inferred from experiments on human patients that these are different for SMP in CRPS II compared with SMP in CRPS I.
Following nerve lesions, afferent nociceptive and non-nociceptive neurons, as well as sympathetic postganglionic neurons, undergo dramatic changes leading to sprouting, shrinkage, and eventually even death of the lesioned neurons, as well as to up- or downregulation of transmitters (including neuropeptides), ionic channels, transduction molecules, and other entities, one such change being upregulation of
α-adrenoceptors (46,47). Noradrenaline released by the sympathetic nerve fibers may activate and/or sensitize the afferent neurons. The sympathetic–afferent coupling may occur at the lesion site, along the lesioned nerve, or even in the dorsal root ganglion, although the latter, as attractive as it may be, appears to be unlikely. Overall animal models clearly support these
peripheral mechanisms of SMP occurring in CRPS II (48,49,50,51,52). It should be kept in mind that not only coupling of sympathetic neurons to nociceptive afferent neurons but also to non-nociceptive afferent (e.g., mechanosensitive, cold) neurons may emerge as important. Sympathetic activation of these afferent neurons may excite sensitized or hyperexcitable central neurons of the somatosensory system (e.g., in the dorsal horn) and contribute to mechanical or cold allodynia in CRPS II patients.
α-adrenoceptors (46,47). Noradrenaline released by the sympathetic nerve fibers may activate and/or sensitize the afferent neurons. The sympathetic–afferent coupling may occur at the lesion site, along the lesioned nerve, or even in the dorsal root ganglion, although the latter, as attractive as it may be, appears to be unlikely. Overall animal models clearly support these
peripheral mechanisms of SMP occurring in CRPS II (48,49,50,51,52). It should be kept in mind that not only coupling of sympathetic neurons to nociceptive afferent neurons but also to non-nociceptive afferent (e.g., mechanosensitive, cold) neurons may emerge as important. Sympathetic activation of these afferent neurons may excite sensitized or hyperexcitable central neurons of the somatosensory system (e.g., in the dorsal horn) and contribute to mechanical or cold allodynia in CRPS II patients.
Unfortunately, we have no animal models for SMP in CRPS I. It is unlikely that mechanisms of SMP occurring in CRPS II (i.e., after trauma with nerve lesion) can explain SMP in CRPS I. For the mechanisms underlying SMP in CRPS I patients only a minor component of the coupling occurs in the skin. We suggest that an important sympathetic–afferent coupling also occurs in the deep somatic tissues, and that the mechanism of this coupling is indirect, involving the vascular bed and possibly other non-neural components. This mode of coupling has been repeatedly postulated (48,50,51,52). However, it has never been explored experimentally using animal models.
Other potential ways of coupling may exist between sympathetic neurons and afferent nociceptive neurons, which have been developed from experiments using animals but which have not been explored in patients. These modes of coupling do not involve activity in the sympathetic nerve fibers, but the sympathetic fibers may mediate the effects of inflammatory (e.g., bradykinin) or other compounds (e.g., nerve growth factor) to nociceptive fibers in the peripheral tissue. This sympathetic–afferent coupling may emerge as important in inflammatory pain and in CRPS I (51,53,54,55).
Finally, the sympathetic nervous system may be involved in coupling to nociceptive neurons via the adrenal medulla. This mechanism, which has been shown to exist in rats (using behavioral experiments), implies that epinephrine released by the adrenal medulla (during its activation by preganglionic neurons) leads to sensitization of nociceptors for mechanical stimulation. The process of sensitization has a slow time course of days to 2 weeks to develop fully. We do not know the mechanism leading to nociceptor sensitization in this model or whether it is important in understanding SMP in patients with CRPS I (51,56,57,58).
Pain-relieving Effect of Sympathetic Blocks
The pain-relieving effect of sympathetic blocks using local anesthetics applied to the sympathetic chain in CRPS patients with SMP, as documented by quantitative measurements (59), is puzzling. Pain relief outlasts the conduction block of sympathetic neurons by at least one order of magnitude. Sometimes only a few (in the extreme, only one!) temporary sympathetic blocks are necessary to produce permanent pain relief. The long-lasting pain-relieving effects of sympathetic blocks clearly argue that activity in sympathetic neurons, which is of central origin, maintains a positive feedback circuit via the primary afferent neurons. Animal models for positive feedback circuits are absent. We postulate that activity in sympathetic neurons maintains a central state of hyperexcitability (e.g., of neurons in the spinal dorsal horn), via excitation of afferent neurons initiated by an intense noxious event. The persistent afferent activity necessary to maintain such a central state of hyperexcitability is probably low in rate. This central state of hyperexcitability is switched off during a temporary block of conduction in the sympathetic chain lasting only for a few hours, and it cannot be switched on again when the block wears off and the sympathetic activity (and therefore also the sympathetically induced activity in afferent neurons) returns. Finally, the tacit assumption made is that cutaneous (sympathetic and afferent) systems are primarily involved. However, whether sympathetic systems and afferent systems innervating deep somatic tissues are more important in this hypothetical positive feedback circuit has never been tested and needs to be investigated experimentally (42).

Full access? Get Clinical Tree
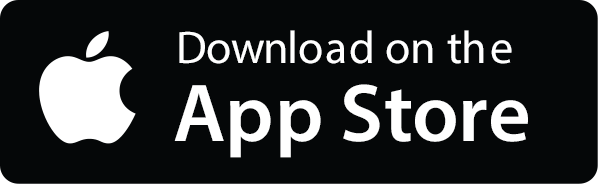
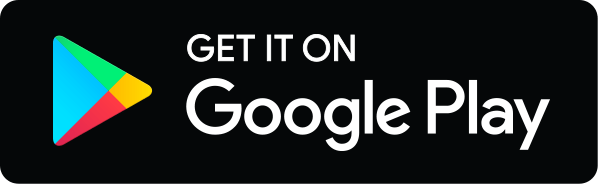
