Chapter 17
Clinical Monitoring II
Respiratory and Metabolic Systems
It is important to note that the modalities chosen to obtain physiologic feedback may or may not lead to a reduction in patient morbidity and mortality.2 Research provides rationale to support the standardization of minimal monitoring modalities. Standards are available to the anesthesia provider from both the American Association of Nurse Anesthetists (AANA) and the American Society of Anesthesiologists (ASA).3,4 It is a matter of clinical judgment by the anesthetist that determines what modalities beyond those minimally required will provide useful information when added to the anesthetic plan of care. It is through this evidence-based approach that best practices are developed.
It is important to emphasize that despite advancements in technology, human vigilance must be maintained and considered primary in assessing patient responses to anesthesia. Human error is always possible and often associated with adverse outcomes. Monitoring alone cannot prevent adverse outcomes, but a timely response to warnings can have a positive impact.5 It cannot be emphasized enough that reliance on technology must never be allowed to lull the clinician into complacency. Although accuracy and reliability of monitors continue to improve, the potential for machine malfunction and artifact is ever present. Timely human response to intraoperative events remains the key to predicting, avoiding, and managing untoward responses to anesthesia and surgery. The basic human senses of sight, hearing, touch, and even smell remain the primary tools in clinical monitoring. The patient is the primary source of assessment data.
Monitoring Standards
Monitoring standards are published and reviewed by anesthesia professional associations so that minimal standards in the provision and monitoring of care can be recommended. Such standards are intended to provide guidance to anesthesia providers and healthcare facilities in evaluating quality care and developing and improving the safety of practice while educating the public regarding patient rights and expectations. Standard V of the AANA Scope and Standards for Nurse Anesthesia Practice outlines specific monitoring requirements necessary for compliance (Table 17-1).4 The standards acknowledge the importance of vigilant monitoring as the basis of safety in anesthesia practice. In addition, the standards point out that the CRNA must constantly be in attendance of the patient until the responsibility for care can be safely transferred to another qualified healthcare provider. They further state that these are intended to be minimum requirements and should be exceeded as deemed necessary in the judgment of the anesthetist. In most cases, when certain monitoring modalities are not used, they must be at least immediately available. The AANA interpretation specifically indicates that alarm limit parameters and audible warning systems should be used, and a specific statement is made recommending the use of variable pitch alarms. The value of variable pitch commonly used in pulse oximetry equipment has been widely appreciated for its ability to provide information regarding subtle changes in oxygen saturation using the sense of hearing, prior to visualizing the monitor. Alarms are designed to protect the patient by alerting the practitioner that the individual is at increased risk and needs immediate assistance.
TABLE 17-1
Scope and Standards for Nurse Anesthesia Practice
Adapted from the American Association of Nurse Anesthetists. Scope and Standards for Nurse Anesthesia Practice; 2010. Available at http://www.aana.com.
An area of concern related to electronic monitoring devices is a phenomenon referred to as alarm fatigue. Alarm fatigue can be defined as a type of human error occurring when a practitioner is desensitized to alarms or alerts.5,6 The ideal alarm would be easy to localize and recognize. It would be evident despite other noises and alarms, yet would allow for effective communication between care providers. The ideal monitoring device would also elicit a minimal number of false alarms. Alarms in many medical devices fall short of the ideal and as a result are subject to being ignored or even disabled by the practitioner—a very dangerous practice. Biomedical specialists continue to develop and perfect alarms to improve their usefulness in alerting the clinician to significant changes in patient status.7
The ever changing and developing technologic capabilities warrant that these standards also allow for consideration of new monitoring modalities as they are introduced. It is important to note that the type of anesthetic given may influence the specific monitoring needed but should not be a factor in considering the level of vigilance or accessibility of advanced monitoring and interventional equipment.
Systematic Approach to Monitoring
A systematic, evidence-based process shown to be effective in reducing anesthetic morbidity and mortality should be the goal when planning appropriate monitoring to be used. As monitoring standards guide practice by prescribing minimums to be adhered to, other processes can be helpful in defining how monitoring should occur and which modalities should be used. In the spirit of learning from previous errors or untoward events, closed-claim studies can provide valuable insight into monitoring techniques and habits that could be useful in preventing future anesthesia mishaps. Incident monitoring systems and practices can be used to collect data for practitioners to use to develop safer processes as in the often referenced analogies made to the aviation industry.8 In a manner of speaking, clinical monitoring can be thought of as a means to avoid critical incidents.
Many algorithms and protocols have been written to guide the practitioner through the monitoring process and ensure thorough, vigilant observation. Simple, systematic approaches to crisis management are often focused on or derived from the common algorithm “ABC,” representing airway, breathing, and circulation. In an anesthesia context, additional foci for monitoring can include color (i.e., cyanosis), oxygen and oxygen analyzers, ventilation, vaporizer or pump settings and status, endotracheal tube patency and placement, monitors and equipment, and drug effects, thereby expanding the crises response algorithm to COVERABCD (Table 17-2).9 There is an enormous value in developing a systematic approach to monitoring. The habit sometimes referred to as “sweeping” the anesthesia field to visualize the patient, with the eyes following a path to the anesthesia machine via the airway and breathing circuit and progressing to the monitoring modalities used, has long been taught as a means of increasing vigilance and attention to detail.
TABLE 17-2
Algorithm | Descriptor |
C—Circulation, Color | Determine adequacy of circulation, check pulse, blood pressure; ECG notes oxygenation through assessment and oximetry |
O—Oxygen, Oxygen analyzer | Check oxygen delivery system, hypoxic guard |
V—Ventilation, Vaporizer | Ventilate by hand to assess breathing circuit and airway patency, assess chest excursion and auscultation; assess ETco2; check vaporizer function |
E—Endotracheal tube | Systematic assessment of ETT if used, including patency, seal, etc |
R—Review monitors, Review equipment | Review all monitors in use, assure appropriate calibration and maintenance, review any and all equipment in contact with the patient |
A—Airway | Check patency of the unintubated airway, assess for laryngospasm, foreign body, etc |
B—Breathing | Assess pattern, rate, and depth of respirations; examine, auscultate, and review ETco2 and pulse oximeter monitors |
C—Circulation | Repeat assessment of circulation |
D—Drugs | Review drugs given; consider needed pharmacologic intervention; consider possibility of medication administration error |
ECG, Electrocardiogram; ETco2, end-tidal carbon dioxide concentration; ETT, endotracheal tube.
Adapted from Runciman WB, Merry AF. Crises in clinical care: an approach to management. Qual Safe Health Care. 2005; 14(3):156-163.
Review of critical incidents has provided insight into how clinicians can enhance safety and prevent mishaps through their use of monitors with alarms. Closed-claims review of various databases supports the notion that vigilance remains a key point, and respiratory events are of particular concern.10 It has been suggested that in cases in which anesthesia is provided outside of the operating room, (e.g., a rapidly growing practice in which monitoring may not be as focused as in a standard anesthetizing area), the number and severity of liability claims may be increased.11 The well known mantra of the ABCs—observing the Airway, Breathing (respiration), and Circulation—remains foremost and critical in anesthesia clinical monitoring. Monitoring drug effects completes the basic monitoring approach. The monitoring of cardiovascular circulation is addressed in Chapter 16. The monitoring of the neurologic system can be found in Chapter 18.
Airway Monitoring
Monitoring the airway includes the observance of gas exchange from the upper to lower airways. Assessment of airway patency is performed in very subtle yet essential ways. Ventilatory movement of the chest must be observed and the presence of any sign of airway obstruction such as retractions, or seesaw motion of the chest and abdomen, is noted. Seeing the presence of condensation in an airway device or clear mask can serve to indicate the presence of gas exchange. The sense of touch can be used to perceive subtle movement of air exchange felt on the hand of the anesthetist. The sense of smell can be the first aid in detecting a disconnected circuit or airway device when volatile agents are being used. Listening for the presence of abnormal airway sounds such as stridor is crucial in noting airway obstruction. The precordial stethoscope is a valuable tool in auscultating the presence or absence of airway exchange during all phases of the anesthetic, regardless of the type administered. Ensuring adequacy of ventilation, whether an endotracheal tube or laryngeal mask airway is in use, must include verification of placement by assessing breath sounds and chest expansion, as well as verification of the presence of carbon dioxide (CO2) in the expired gas.3 While failure to successfully intubate is problematic, failure to recognize misplacement is catastrophic.
Respiratory Monitoring: Ventilation
Skin and nail bed color should be observed as part of the whole patient-assessment picture; however, skin color changes alone are not a reliable measure of whether ventilation and oxygenation are adequate. Cyanosis is a late sign of anemia or hypoxia and can be difficult to assess accurately, given such variables as differences in lighting during certain procedures and lack of controls in comparisons of patients’ coloring throughout the perioperative period to name a few. Besides physical assessment skills, monitoring the adequacy of ventilation must be done throughout the perioperative period, using multiple parameters. Several themes are evident that relate to safety and vigilance in monitoring when reviewing closed-claims studies for anesthesia incidents. The most prominent of these is the value of certain specific monitors and their alarms. These include pulse oximetry, end-tidal carbon dioxide measurement, oxygen analyzers, and disconnect alarms. Of equal importance is the users’ ability to assure the proper function of these prior to administering an anesthetic.10
Carbon Dioxide Monitoring
The measurement of arterial blood gases to determine level of carbon dioxide provides direct measurement of ventilation and metabolic status, and the necessity of this action may be indicated at times. The means of measuring the carbon dioxide tension in the blood (Paco2) is based on hydrogen ion concentration because CO2 reacts with water to produce hydrogen ions through a reversible reaction. This reaction yields carbonic acid, which dissociates to yield hydrogen and bicarbonate ions,12 as shown in the following equation:
The production of carbonic acid also allows for the qualitative—and to a limited extent, quantitative—detection of the presence of carbon dioxide through the use of disposable end-tidal carbon dioxide (ETco2) detector devices. These colorimetric devices react to changes in pH and display it as a color change. They are widely used in emergency settings to verify proper placement of an endotracheal tube.13 Although these devices are sensitive enough to detect carbon dioxide quickly, a minimum of six breaths has been suggested to avoid misinterpretation. False positives may result from the detection of CO2 from air forced into the stomach during mask ventilation or the presence of carbonated beverages or antacids in the stomach.14
The means to measure CO2 level must be based on the patient’s condition, the type of anesthetic administered, and the complexity of the surgical procedure. The continuous measurement of carbon dioxide in expired gas provides a practical, noninvasive, and accurate reflection of arterial blood carbon dioxide and is the most common means of monitoring carbon dioxide levels in the anesthesia setting. It is also a monitoring standard of care during general anesthesia, for the patient being ventilated or whose ventilations are being assisted. In addition, the ASA monitoring standards state that “during moderate or deep sedation the adequacy of ventilation shall be evaluated by continual observation of qualitative clinical signs and monitoring for the presence of exhaled carbon dioxide unless precluded or invalidated by the nature of the patient, procedure, or equipment.”3 Accuracy of ETco2 as a correlation with arterial carbon dioxide has been well documented. End-tidal CO2 is said to be approximately 2 to 5 torr lower than arterial CO2 in patients who have no cardiac or pulmonary abnormalities.14 Capnometry is a term that encompasses all means of measuring carbon dioxide, whereas capnography refers to the recording of the measurement. The term capnogram is used to describe a continuous display of carbon dioxide during the phases of ventilation. The continuous measurement of ETco2 is accomplished through the use of infrared analysis. When a gas mixture containing more than one substance, (e.g., an exhaled gas sample) is analyzed, a quantitative measurement can be made to determine the proportional contents. Each gas in the mixture absorbs infrared radiation at a different wavelength. The amount of CO2 is measured by detecting its absorbance at specific wavelengths and filtering the absorbance related to other component gases. Older monitors had difficulty distinguishing between nitrous oxide and CO2, but this fault is corrected in current models.12
Sampling of ETco2 can be accomplished through either a nondiverting (also known as mainstream) or diverting (also known as sidestream) monitor. A nondiverting monitor measures gas directly within the breathing system. Gas passes through a wide-chambered sensor that fits over a connector between the anesthesia circuit and mask adapter. The sensor is connected to the monitor by a cable. Nondiverting monitors offer several advantages. They have minimal sampling-time delays, use few disposable items, and do not require scavenging because gas is not removed from the system. Disadvantages include the inability to measure gases other than CO2 and nitrous oxide, an increase in circuit deadspace by the adapter, and greater risk of interference by condensation and secretions. Also, because the sensor and cable are attached in proximity to the patient, the added weight may cause traction on the tube, increase the risk of circuit disconnect, and make sampling in a nonintubated patient difficult.
The other more commonly used CO2 monitor type is the diverting monitor. The diverting monitor extracts gas from sample tubing attached near the patient end of the circuit and pumps it to the monitor. Disadvantages include the need for scavenging of sampled gases because they are removed from the circuit and some risk of contamination by condensation or secretions exists. Advantages of the diverting system include minimal increase in deadspace and versatility in gas analysis because the sample can also be sent to anesthetic agent monitors. In addition, the small, lightweight tubing can be adapted to sample awake, spontaneously breathing patients through the mouth, nares, or simple mask.14
It has become common to adapt the sampling line of a diverting ETco2 monitor to trace a capnogram in awake or sedated, spontaneously breathing patients receiving O2 via simple mask or nasal cannula. Nasal cannula tubing already equipped with a sampling line is commercially available. It has been shown that sampling ETco2 in the spontaneously breathing patient’s hypopharynx is reliable and accurate and that the exact position and use of supplemental O2 flow will not affect reliability.15 The use of ETco2 monitoring as a warning of hypoventilation or excess sedation has gained attention outside of the anesthesia setting. For example, ETco2 monitoring has been shown to be a more sensitive indicator of hypoventilation than clinical observation or pulse oximetry when used during sedation in the emergency department setting.16 Other studies note the value of ETco2 monitoring during sedation as well and note the advantage over pulse oximetry alone in detecting hypoventilation in patients receiving supplemental O2. It has been suggested that like pulse oximetry, ETco2 monitoring should be considered a standard of care for patients receiving sedation outside the operating room.17
End-Tidal Carbon Dioxide Capnography
The normal time capnogram can be recorded at varying speeds. A fast speed setting of approximately 12.5 mm/second allows interpretation of individual respiratory components and short-term changes, whereas a slow speed of approximately 25 mm/minute displays long-term changes.18 Although no standard descriptions exist for the components of the capnogram, it is typically described as encompassing four basic phases (Figure 17-1). These phases are often displayed in a classic waveform shape best recorded during mechanical ventilation in an intubated patient. It is important to note that the basic shape of the wave form will vary depending on the mode of airway management used (endotracheal tube, laryngeal mask airway, simple mask, nasal cannula, etc.) as well as in comparison to the spontaneously breathing patient.
Although the shapes may vary, the represented phases apply universally. The first phase is the end of inspiration and very beginning of expiration. Gas sampled at this point identifies the baseline. This sample comes from the anatomic deadspace and contains no CO2. This portion in a normal capnogram should approximate zero. The second phase is the expiratory upstroke. Gas sampled represents a mix of deadspace and alveolar gas and thus records measurable CO2. This phase represents the rapid passing of initial expired gas through the upper airways. The third phase represents the plateau and records alveolar emptying of CO2. In the normal pulmonary measurement, the plateau is very nearly flat. This phase represents the longest duration of the measurement. ETco2 is measured at the end of the plateau just prior to the beginning of phase four. The fourth phase is displayed as the rapid decrease in CO2 concentration of sampled gas as a result of inspiration of air or O2. This downstroke returns the recorded CO2 measurement to or very near to zero. Variations in the capnograph tracing can be very subtle and represent specific alterations in the ventilatory process. Some common deviations are worth noting. In the process of evaluating the capnogram, the anesthetist should note the respiratory rate, whether ventilation is spontaneous or mechanical, the value of measured end-expired CO2, the shape of the recorded waveform, and the presence of additional respiratory efforts. Deviations from what is normally a close approximation of arterial CO2 can occur. End-tidal CO2 measurements may be inaccurate in the presence of significant ventilation and perfusion mismatching. When ventilation-to-perfusion ratio is large, the resultant increase in deadspace causes a low concentration of ETco2 overall.19 In addition, small tidal volumes—reflecting inadequate alveolar ventilation—may produce ETco2 recordings that significantly underestimate arterial CO2 levels. Some controversy exists as to the relative accuracy of capnography during laparoscopic procedures.20
After esophageal intubation, an initial, slight upstroke of carbon dioxide may be seen in those rare circumstances when carbon dioxide may be sampled from the stomach as mentioned previously in reference to disposable ETco2 detector devices. These CO2 measurements are the result of excess air blown into the stomach during overzealous ventilation or a partially obstructed airway. Such a waveform will display for a very brief period and be followed by a measurement of zero. A waveform that fails to return to baseline during phases one and four indicates that rebreathing of carbon dioxide is occurring. This can be the result of inadequate fresh gas flow in the nonrebreathing system or a depleted or ineffective carbon dioxide absorber (Figure 17-2). Although this may be detected over time, it can be difficult to distinguish.
FIGURE 17-2 Elevation of the baseline indicates rebreathing. (Courtesy Novametrix Medical Systems, Wallingford, Conn.)
Sloping of the plateau phase represents a progressive prolongation of expiration. It is typically the result of either an obstruction of expired gas flow at some point along the airway or ventilation-perfusion mismatch (Figure 17-3). It also can be indicative of chronic obstructive lung disease because CO2

Full access? Get Clinical Tree
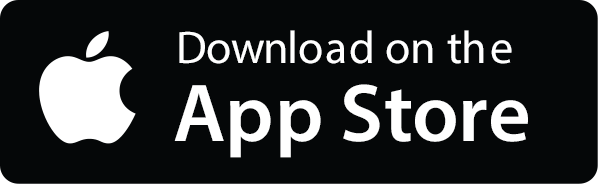
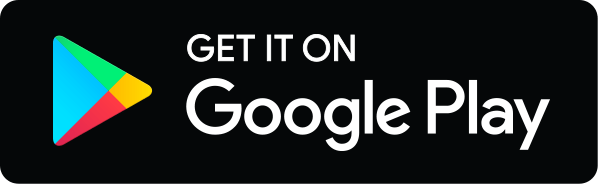
