Fig. 14.1
Chemical structures of phencyclidine and (S)-ketamine
Ketamine is increasingly administered as a pure analgesic [7, 8], for induction of rapid sequence intubation performed outside the operating room [9], for bronchodilation in patients with asthma [10], as a pharmacologic restraint for agitated and combative patients in the emergency department as well as in the field [11], and more recently, for treatment of selected mental health disorders [12]. This chapter will review the history, pharmacology, clinical effects, and applications of ketamine. With each clinical effect of ketamine described, we will progress to the logical clinical applications and cautions for ketamine use that have resulted. We will examine the evidence for and against traditional and expanding indications for ketamine administration. Due to ketamine’s significant potential as a drug of abuse, we will explore the nonmedical use of ketamine as well [4, 13].
History
Over 50 years ago, ketamine was initially administered to humans under the original investigation number, CI-581 [4]. It was developed as a short-acting intravenous anesthetic due to the shortcomings of phencyclidine and cyclohexamine which resulted in long-lasting psychotomimetic activity (i.e., emergence delirium or recovery agitation) during the postanesthetic period [1, 4, 5]. Initial human studies of ketamine noted its ability to induce a trance-like, cataleptic state which inspired these investigators to coin the term “dissociative anesthetic” [4, 14, 15]. When compared to phencyclidine, ketamine was found to provide similar anesthetic effect but with a shorter half-life and with less problematic emergence delirium [6]. In addition, ketamine was found to preserve protective airway reflexes with minimal clinical respiratory or circulatory depression [16, 17]. Ketamine was approved by the Food and Drug Administration as an anesthetic and introduced into clinical practice in 1970 [5, 14] and due to its effectiveness and large margin of safety, was administered to injured American soldiers requiring anesthesia during the Vietnam War [1, 4].
In addition to its dissociative effects, ketamine also produces potent analgesia, at sub-anesthetic concentrations, and short-term amnesia [6]. The combination of these three properties (i.e., dissociative sedation/anesthesia, analgesia, and amnesia) makes ketamine a unique drug in clinical practice and, when coupled with its favorable safety profile, explains why ketamine use and indications have expanded so dramatically [1]. Ketamine is currently the single most commonly administered drug to children to facilitate the performance of painful procedures in the emergency department [17, 18]. In addition to prominent clinical use, due to its unique dissociative and psychedelic properties, the potential for abuse of ketamine, like its parent drug phencyclidine, must also be considered [13, 19]. Ketamine was made a Schedule III controlled substance in the USA in 1999 [4, 6], and reports of ketamine abuse in the USA, Europe, and Southeast and East Asia are growing [6, 13].
Pharmacology
The dissociative state produced by ketamine is the result of a functional and electrophysiological dissociation between the thalamo-neocortical and limbic centers of the brain, preventing higher centers from perceiving visual, auditory, or painful stimuli [6, 16, 19]. Limbic system functions such as processing sensory information, regulating emotions, and facilitating the development of long- and short-term memory are affected by ketamine administration resulting in the dissociative state, analgesia, suppression of anxiety and fear, and amnesia [20]. The varied clinical effects of ketamine are believed to be mediated through its binding to multiple sites, primarily N-methyl-d-aspartate (NMDA) but also non-NMDA glutamate, nicotinic and muscarinic cholinergic, and opioid receptors in the central nervous system (CNS) [1, 5, 16, 20, 21]. Blockage of the excitatory neurotransmitter, glutamate, from binding NMDA receptors is the most likely etiology of ketamine dissociation [21]. The unique and diverse mechanisms of action of ketamine in the CNS result in a range of clinical effects and applications that we will discuss in this chapter.
Intravenous (IV) ketamine, 1.5–2 mg/kg, produces peak serum and CNS concentrations within 1 min of administration which reliably induces clinical dissociation [16, 17]. Due to the subsequent rapid, high CNS levels, recommendations for IV ketamine support administration over a 30–60 s time interval to prevent associated respiratory depression or apnea [17]. The duration of action of traditionally administered IV dissociative-dose ketamine is about 10 min with full recovery observed between 1 and 2 h [22]. However, in a recent study of lower dose (0.7–0.8 mg/kg), rapid administration (less than 5 s) of IV ketamine, a shorter period of sedation was produced, and no respiratory depression was noted in this small sample of children who received ketamine for forearm fracture reduction [23]. Using this rapid administration, lower-dose method, the total time of sedation was reduced to about 25 min. While this method of ketamine administration is promising, especially in busy clinical settings where more rapid patient recovery from sedation will allow for quicker patient turn-around times, further study comparing rapid administration, lower-dose ketamine to traditional administration , dissociative-dose ketamine is required to fully elucidate the risks and benefits.
Intramuscular (IM) ketamine is dosed 4–5 mg/kg to induce dissociative sedation [17] and has an onset of action of 3–5 min [24]. The duration of action of IM ketamine is significantly longer than ketamine administered IV with time to full recovery exceeding 2 h [25]. Despite the increased length of sedation, one comparison of IM vs. IV ketamine found similar overall length of stay in the emergency department which suggests that the ease of IM administration, as compared to the time required for IV placement, proves to be a significant time saver [24]. IM ketamine has the distinct advantage of not requiring IV placement which results in more rapid administration of the drug and is important for patients in whom IV access is particularly problematic. A randomized, controlled trial of IV vs. IM ketamine in 225 children found those who received IM ketamine vomited significantly more frequently (26.3 % vs. 11.9 %) and had a longer length of sedation (129 vs. 80 min) than those who received IV ketamine. This study was terminated early because of nursing resistance to IM-administered ketamine based on increased vomiting and the longer recovery times observed in these patients, which resulted in a strong nursing preference for IV ketamine. Importantly, respiratory adverse events experienced by these patients were similar between the two routes of administration (4.0 % IM vs. 8.3 % IV), and IV access was not required to manage any adverse event [25]. In a recent review of the IV vs. IM ketamine literature, the authors conclude that IV ketamine “appears to have a better adverse events profile than IM ketamine…(and) has a shorter recovery.” The authors recommend IV ketamine when intravenous access is readily available, but note that IM ketamine is acceptable for sedation and may be preferable when “it may be difficult to reliably establish IV access with minimal distress to the child.” [26].
Although the bioavailability of intranasal (IN) ketamine has been reported to be poor, about 45 %, the onset of action is rapid and duration of action of 2–3 h makes IN ketamine administration popular among recreational users [6]. Studies describing the use of IN ketamine for pain and sedation have been published in a number of settings including prehospital, emergency department, postoperatively, and in dental practice [27]. IN ketamine has been successfully administered to adults and children for analgesia [28, 29]. Ketamine, 1 mg/kg IN, provided similar pain relief to children with moderate to severe pain from limb injuries as IN fentanyl, 1.5 mcg/kg, in a recent randomized controlled trial. Children in the IN ketamine group were more likely to experience drowsiness and dizziness and more frequently reported a bad taste in the mouth than those who received IN fentanyl [28]. While IN ketamine has been shown to be effective as a premedication or anxiolytic drug , [30, 31] IN ketamine for procedural sedation has not been found to be particularly effective [32]. In a randomized, controlled trial comparing three doses of IN ketamine (3 mg/kg vs. 6 mg/kg vs. 9 mg/kg) for sedation of children receiving laceration repair, only the 9 mg/kg group produced adequate sedation, and the study was suspended due to ineffectiveness of sedation, as per predetermined criteria. It is not clear whether such a high dose of IN ketamine will allow for reliable sedation given the high volume of drug required and degree of subsequent unabsorbed nasopharyngeal runoff which may be expected even with more concentrated ketamine formulations [32].
Orally ingested ketamine is poorly absorbed with bioavailability reported to be as low as 16 % [6]. The onset of action of oral ketamine is much slower than IV, IM, or IN routes with plasma concentrations detected about 30 min after administration. Peak plasma concentrations of oral ketamine are about 20 % of that obtained when a similar dose of ketamine is administered IM [6]. Oral ketamine, 5–10 mg/kg, has been used to augment laceration repair analgesia and sedation with positive results; however, this route is not commonly used for procedural sedation outside the operating room [33, 34]. Table 14.1 provides a summary of ketamine dosing by indication and route of administration.
Table 14.1
Ketamine dosing by indication and route of administration
Indication | Route | Dose |
---|---|---|
Dissociative sedation | IV | 1.5–2 mg/kg |
IM | 4–5 mg/kg | |
Brief sedation—rapid administration <5 s | IV | 0.5–0.8 mg/kg |
Analgesia | IV | 0.2–0.75 mg/kg |
IM | 1–3 mg/kg | |
IN | 0.5–1 mg/kg | |
Induction of rapid sequence intubation | IV | 2 mg/kg |
Moderate to severe asthma | IV | 2 mg/kg |
Pharmacologic restraint | IM | 4–5 mg/kg |
Clinical Effects and Applications
Protective Airway Reflexes and Laryngospasm
As mentioned previously, ketamine has become the most common agent used in the emergency department for children in need of painful procedures due to its desirable effects of sedation, analgesia, and amnesia but also because of its favorable safety profile [16–18]. Foremost, ketamine does not suppress normal upper airway protective reflexes such as coughing, sneezing, and swallowing, reducing the risk for pulmonary aspiration [16, 20]. For unfasted children who require emergent procedural sedation, ketamine may be the safest drug choice available due to the retention of airway protective reflexes [21]. Additionally, respiratory depression with ketamine is rare. In an individual patient data meta-analysis of 8282 children, apnea occurred in 63 (0.8 %) and laryngospasm in 22 (0.3 %), and the overall incidence of airway and respiratory adverse events defined as upper airway obstruction, oxygen desaturation less than or equal to 90 %, apnea, or laryngospasm was 3.9 % [2]. In this study, risk factors for ketamine-associated airway and respiratory adverse events were determined to be high intravenous doses (initial dose ≥2.5 mg/kg or total dose ≥5.0 mg/kg), administration to children younger than 2 years or aged 13 years or older, and the use of coadministered anticholinergics or benzodiazepines [2] (Table 14.2).
Table 14.2
Risk factors for ketamine-associated adverse events
Adverse event | Risk factors | Comments |
---|---|---|
Airway and respiratory adverse eventsa | Independent predictors • Age less than 2 years • High IV dosing (initial dose ≥2.5 mg/kg or total dose ≥5 mg/kg) • Coadministered benzodiazepine • Coadministered anticholinergic | NOT independent predictors • Minor oropharyngeal procedures • Underlying physical illness (ASA class ≥III) • Route of administration IV vs. IM |
Laryngospasm | • No association of age, dose, oropharyngeal procedure, underlying physical illness, route, or coadministered anticholinergics | • Laryngospasm appears to be idiosyncratic • Clinicians must be prepared for its rapid identification and management |
Vomiting | • More common in children 5 years and older • Peak age is early adolescence • Increased when administered IM • Increased with high doses IV | • Incidence may be decreased with coadministered ondansetron |
Unpleasant recovery reactions | • Not age related to a clinically important degree in children • Presence of significant presedation agitation may contribute | • Not decreased with coadministered midazolam in children • Decreased with coadministered midazolam in adults |
Ketamine is a sialagogue; it stimulates salivary and tracheobronchial mucous gland secretions and acts to exaggerate laryngeal reflexes [5, 17]. Anticholinergics such as atropine or glycopyrrolate have been coadministered with ketamine to reduce excessive salivation associated with ketamine which, it has been postulated, may precipitate laryngospasm [16, 21]. Recent study of the effects of anticholinergics on ketamine administered in the emergency department to children has shown that while hypersalivation decreases, anticholinergics have not been proven to provide a clinical benefit, that is, no difference in airway adverse events [35–37]. Coadministration of anticholinergics with ketamine is no longer routinely recommended and has been identified as a risk factor for ketamine-associated airway and respiratory adverse events, in one study, although the causative mechanism of this finding is not known [2, 17].
In one case-controlled study, the risk of laryngospasm in children undergoing general anesthesia was increased with active upper respiratory tract infection and in patients with an airway anomaly [38]. The incidence of ketamine-associated laryngospasm is unknown, although as mentioned previously, laryngospasm occurred in only 0.3 % of children in a meta-analysis of 8282 children [2]. A case-controlled analysis of this large ketamine data set found no evidence of association with laryngospasm based on age, dose, procedure performed (including minor intraoral procedures), the American Society of Anesthesiologists (ASA) risk classification status, route of administration (IV or IM), or the administration of anticholinergics [39]. The impact of an upper respiratory infection on ketamine-associated airway and respiratory adverse events was not studied. The authors conclude that laryngospasm occurrence is idiosyncratic and recommend that clinicians be prepared for laryngospasm anytime they administer ketamine.
Ketamine-associated laryngospasm may occur at any time from induction to emergence from sedation, and it may be brief or recurrent [39]. Cases of laryngospasm have been reported to have occurred with IV and IM ketamine. Laryngospasm in these cases was successfully managed with positive pressure ventilation delivered with simple bag-mask equipment and did not recur [39–42]. However, one report of two cases of recurrent, severe laryngospasm which were resistant to management with bag-mask ventilation has been reported [43]. In this case report, one patient required muscle relaxation with succinylcholine and endotracheal intubation. Although generally very safe, with low rates of airway and respiratory complications, clinicians administering ketamine must be prepared to provide advanced airway management on rare occasions.
Cardiovascular Effects
Unlike most sedative, anesthetic , and analgesic agents, ketamine is effectively a cardiovascular stimulant, producing increases in heart rate, cardiac output, and blood pressure in healthy patients [19, 20, 44, 45]. Although ketamine has a direct myocardial depressive effect, it indirectly stimulates the circulatory system by increasing circulating catecholamine levels [46]. The sympathomimetic effects of ketamine are believed to be primarily due to direct stimulation of CNS structures [5]. Ketamine blocks the reuptake of the catecholaminergic hormones norepinephrine, epinephrine, dopamine, and serotonin resulting in a 10–30 % increase in blood pressure and heart rate [21]. However, in critically ill patients, with severely depleted catecholamine stores, ketamine does not provide sympathomimetic effects [1]. In patients with limited myocardial reserve and increased demand as seen in shock, sepsis, and significant hypovolemia, ketamine may decrease cardiac output and may exacerbate or induce hypotension and bradycardia [21, 45]. In a recent, multicenter, prospective, randomized, controlled trial of critically ill adults, ketamine was compared to etomidate for induction of rapid sequence intubation. Both drugs performed similarly in facilitating rapid sequence intubation which resulted in patients having similar sequential organ failure assessment (SOFA) scores. The authors concluded that ketamine is a safe and valuable alternative to etomidate for endotracheal intubation in critically ill patients and should be considered in those with sepsis [47]. Based on these seemingly conflicting conclusions, it is not absolutely clear how the cardiovascular effects of ketamine will affect critically ill patients, and outcomes may be related to the degree of illness and severity of depletion of catecholamine stores. While ketamine appears to be a good option for induction of rapid sequence intubation for most critically ill patients, these conflicting findings must be taken into consideration when administering ketamine to critically ill patients with depleted catecholamine stores.
Due to its sympathomimetic effects, ketamine use has historically been discouraged for children or adults with known or possible coronary artery disease, congestive heart failure, or hypertension [5, 17, 19]. A recent clinical practice guideline for ketamine use in the emergency setting points out that the evidence suggesting that ketamine sympathomimetic effects may be problematic to patients with these conditions is inconclusive and that ketamine administration is not contraindicated in these patients [17]. However, the cardiovascular effects of ketamine are best tolerated in healthy individuals, and the risks and benefits to patients with coronary artery disease, congestive heart failure, or hypertension must be considered prior to ketamine administration.
Despite concerns for ketamine effects in critically ill patients with depleted catecholamine stores and underlying cardiac conditions, ketamine may prove to be the best option for these patients by providing less cardiovascular suppressive effects as compared to other commonly administered agents such as propofol, benzodiazepines, etomidate , and barbiturates.
Pulmonary Effects
In a study of ketamine, 2 mg/kg IV, administered to healthy (ASA I) children as a bolus, ketamine did not affect resting respiratory rate, tidal volume, end-tidal carbon dioxide (CO2) tension, or minute ventilation [48]. At doses typically administered to patients for brief procedural sedation, ketamine does not produce significant respiratory depression and is a bronchodilator which has been shown to decrease bronchospasm [5, 17, 19]. The mechanism of diminished bronchospasm with ketamine administration is thought to be due to subsequent increase in circulating catecholamines, direct smooth muscle dilatation, and inhibition of vagal outflow [17]. Respiratory adverse events including oxygen desaturation <90 %, apnea, or laryngospasm , associated with ketamine sedation in children, have been shown to occur infrequently in one observational study (121 adverse events with 1791 ketamine administrations, 6.8 %) and a large, individual patient data meta-analysis (319 adverse events with 8282 ketamine administrations, 3.9 %) [2, 41]. In two studies of children, the addition of midazolam to emergency department ketamine sedation was associated with greater respiratory depression and increased respiratory adverse events as compared to ketamine alone [40, 41]. Respiratory adverse events in these two studies were predominantly managed with non-invasive maneuvers such as airway repositioning, verbal cues, tactile stimulation or oxygen administration. Positive pressure ventilation was applied using brief bag-mask ventilation in a few patients (less than 1 %), and no patients received endotracheal intubation [40, 41].
Although ketamine only rarely causes respiratory suppression or compromise, patients who receive dissociative doses of ketamine must be closely monitored and clinicians must be prepared to provide patients with airway repositioning, verbal cues, tactile stimulation, oxygen, and at least brief positive pressure ventilation with bag mask on infrequent occasions (slightly less than 1 % of the time).
Early work by Corssen et al. reported ketamine to be specifically beneficial in anesthesia for the asthmatic patient by diminishing existing bronchospasm and wheezing at time of induction [49]. In a case series of five adults with status asthmaticus in the emergency setting, four patients displayed immediate improvement of respiratory acidosis after receiving ketamine 1–2 mg/kg IV for intubation with succinylcholine. A fifth patient, intubated with diazepam and succinylcholine, experienced a dramatic rise in pCO2 with intubation which was successfully treated with IV ketamine [46]. In a case report of two children with status asthmaticus, ketamine at dissociative doses, 2 mg/kg IV, followed by continuous infusion of 2 mg/kg/h was associated with improvement in bronchospasm which the authors report prevented intubation and mechanical ventilation [50]. Similar results were reported in an adult who received a dissociating dose of ketamine as a temporizing measure to avoid mechanical ventilation due to a severe asthma exacerbation [51]. While dissociative doses of ketamine provide bronchodilatory effects in patients with status asthmaticus and may be effective in assisting with intubation and even prevent mechanical ventilation, sub-dissociative doses of ketamine administered to adults and children have not been shown to be effective in significantly diminishing bronchospasm in asthma exacerbations [52, 53]. Although its bronchodilatory properties appear to make ketamine a promising addition to the treatment of patients with moderate to severe asthma, further study of the effects of dissociative and sub-dissociative doses of ketamine is needed to clarify the role of ketamine in the treatment of patients with asthma.
Effects on Intracranial and Intraocular Pressure
Ketamine increases cerebral blood flow and intracranial pressure in spontaneously breathing patients [1]. However, for ventilated patients, even those with head trauma, ketamine has not been shown to increase, and may actually decrease, intracranial pressure [21]. A large review of the effect of ketamine on intracranial and cerebral perfusion pressure and health outcomes concluded that the use of ketamine in critically ill patients did not appear to adversely affect patient outcomes [54]. Patients with head trauma may receive ketamine for induction of rapid sequence intubation which has the added benefit of providing blood pressure support to patients who may experience cardiovascular instability due to blood loss from associated injuries [17, 55, 56]. Given the recent evidence, patients with intracranial hypertension due to structural barriers to normal cerebrospinal fluid flow (i.e., hydrocephalus) would appear to be the only patients for whom ketamine administration is contraindicated due to concerns arising from intracranial pressure [17, 57].
A range of levels of increased intraocular pressure have been reported in association with ketamine [16]. However, two recent case series of healthy children, without eye injuries, found no significant change in intraocular pressure with ketamine administered for procedural sedation [58, 59]. While ketamine appears to have no effect on intraocular pressure in healthy children, further study of the effects of ketamine on intraocular pressure in patients with eye injuries or glaucoma is warranted before current guidelines which identify increased intraocular pressure as a relative contraindication to ketamine administration for emergency department procedural sedation may be revised [17].
Dissociative Effects
As mentioned previously, the dissociative sedation or anesthesia provided by ketamine is primarily the result of the excitatory effects which result with ketamine inhibition of glutamate binding to NMDA receptors in the CNS [1, 5, 16, 20, 21]. In the resulting state, patients do not respond purposely to external stimuli. Commonly, the patient’s eyes remain open and nystagmus occurs [5]. Patients may make involuntary movements and sounds. It is important to inform patients and their families or care providers of these effects, which are generally harmless, but may be quite alarming to the uninitiated. The dissociative state lends itself very well to facilitating the completion of a wide range of procedures. However, due to ketamine-associated involuntary, non-purposeful movements, sedation for procedures which require the patient to be motionless for any meaningful length of time, such as magnetic resonance imaging (MRI), is better accomplished using other agents.
To reliably achieve the dissociative state, a range of dosing for ketamine has been proposed [5, 16, 17]. A recent emergency department clinical practice guideline recommends 1.5–2.0 mg/kg IV and 4–5 mg/kg IM for children and 1.0 mg/kg IV for adults for dissociative sedation [17]. To achieve analgesia, sub-dissociative, low-dose ketamine is recommended, 0.2–0.75 mg/kg IV, [5, 16, 60–62], 1–3 mg/kg IM [5, 62], and 0.5–1 mg/kg IN [28, 29] in children and adults.
The combination of dissociative sedation and analgesia with a favorable safety profile made ketamine ideal to provide care required for children and adults who experienced severe pain due to treatment of burns. Burn units used ketamine extensively for dressing changes, wound debridement, and skin grafting procedures [5, 63]. In 1971, Corssen et al. reported that ketamine was their drug of choice for the care of burn patients as ketamine “…does not depress respiratory function, while it stimulates the cardiovascular system…the protective laryngeal and pharyngeal reflexes (are maintained)…thereby ensuring an unobstructed airway without the need for endotracheal intubation, regardless of the patient’s position.” [63].
The use of ketamine rapidly progressed from these beginnings. Starting in the 1970s, ketamine administered IM was used by “surgeon-anesthetists” for a variety of minor to major surgical procedures in developing countries [64, 65]. Phillips and Walker each describe their experience in the South Pacific of administering IM ketamine and performing surgery without the need for endotracheal intubation and without significant complication in most cases. Due to the ease of administration and its favorable cardiorespiratory effects, ketamine administration for painful procedures and surgeries spread to the battlefield and in disaster situations where supportive resources were severely limited [19, 66]. Ketamine use continued to expand from painful procedures in burn patients to obstetric procedures and “outpatient anesthesia” administered as an IV bolus or as a continuous IV infusion [5, 19, 67].
Ketamine has now been used successfully outside of the operating room for the sedation of children receiving a wide range of emergent and elective procedures including, but not restricted to, orthopedic reduction and arthrocentesis, incision and drainage of skin abscesses, laceration repair and wound care, lumbar puncture, bone marrow biopsy/aspiration, oropharyngeal procedures, foreign body removal, dental procedures, and diagnostic imaging [2, 3, 41, 68]. The sedative, analgesic, and amnestic properties of ketamine coupled with minimal respiratory depression and maintenance of intact airway protective reflexes make it an ideal agent for facilitating the performance of painful procedures in the emergency department [2, 3, 17].
A number of clinical trials which compare ketamine with other agents and combinations provide further insights into the strengths and weaknesses of sedation with ketamine. In a randomized, controlled trial of ketamine and midazolam vs. fentanyl and midazolam for emergent orthopedic reduction in children aged 5–15 years, Kennedy et al. found ketamine/midazolam to be more effective for pain and anxiety relief and to result in fewer respiratory complications than fentanyl/midazolam [69]. The authors further report that both regimens facilitated orthopedic reduction, produced amnesia, and rarely caused emergence delirium. The ketamine/midazolam group experienced more vomiting (3 % vs. 0 %) but less hypoxia (6 % vs. 25 %), less need for breathing cues (1 % vs. 12 %), and less need for supplemental oxygen (10 % vs. 20 %) than the fentanyl/midazolam group. Two patients (1.5 %) in the ketamine/midazolam group required brief positive pressure ventilation provided by bag mask. Orthopedic surgeons favored the ketamine/midazolam regimen despite the fact that recovery was 14 min shorter in the fentanyl/midazolam group [69].
Adverse events associated with IV ketamine alone, ketamine/midazolam, midazolam/fentanyl, and midazolam alone were compared in an observational case series of 2500 children who received sedation for emergency procedures [41]. Respiratory adverse events occurred less frequently in ketamine alone (6.1 %) and in the ketamine/midazolam (10 %) groups than in the midazolam/fentanyl group (19.3 %). As a single agent, midazolam use is limited as it provides no analgesia; however, patients who received midazolam alone experienced respiratory adverse events only slightly less commonly (5.8 %) than patients who received ketamine alone. As opposed to respiratory adverse events, vomiting was more common in children who received ketamine alone (10.1 %) than those who received ketamine/midazolam (5.4 %), midazolam/fentanyl (1.8 %), or midazolam alone (0.8 %). From the results of this study, it would appear that the addition of midazolam to ketamine increases respiratory adverse events and decreases the incidence of vomiting. These findings are consistent with the known respiratory suppressive and antiemetic properties of midazolam. Based on the results of this study and Kennedy’s, we can conclude that ketamine, with or without midazolam, will provide more consistent sedation and analgesia and result in fewer respiratory adverse events but more vomiting than the commonly administered combination of midazolam/fentanyl when used for procedural sedation of children in the emergency department [41, 69].
Comparison of the longer-term effects of ketamine with or without midazolam vs. fentanyl/midazolam was performed through a prospective observational study of 554 children whose families were contacted to investigate the occurrence of adverse events after discharge [70]. The authors found post-discharge vomiting to be common (18 %) across all groups, and there was a low prevalence of adverse behavioral events in children once they returned to their homes . The fentanyl/midazolam group was associated with higher adverse behavioral scores than children who received ketamine alone or ketamine/midazolam [70].
The ultrashort-acting sedative agent propofol is commonly administered to children and adults for elective and emergent procedural sedation outside the operating room [71–74]. Godambe et al. compared propofol/fentanyl to ketamine/midazolam for sedation of children 3–18 years of age for emergent orthopedic procedures [75]. To assess efficacy of sedation, observers blinded to the agents administered scored patient distress during the procedure using a standardized scale. Distress scores were low in both groups, although recovery time was significantly shorter in the propofol/fentanyl group. Oxygen desaturations were more common in the propofol/fentanyl group as compared to the ketamine/midazolam group (30.5 % vs. 7.4 %). One patient in the propofol/fentanyl group experienced laryngospasm , and no patients had apnea in either group. Emesis occurred in 3.7 % of the ketamine/midazolam group and 0 % in the propofol/fentanyl group. The authors concluded that despite a greater potential of respiratory depression and airway obstruction as compared with ketamine, propofol offers unique advantages of quicker offset and smoother recovery [75].
In a randomized controlled trial of adults who received either propofol or midazolam/ketamine for painful orthopedic manipulation, the effectiveness of sedation outcomes was similar between groups [76]. Patients who received propofol experience a more rapid recovery (7.8 vs. 30.7 min) and shorter duration of sedation (16.2 min vs. 41.6 min) than the midazolam/ketamine group. The overall rate of respiratory and hemodynamic adverse events was 20 % for the propofol group and 10 % for the midazolam/ketamine group [76].
In a prospective, non-randomized study of sedation for oncology procedures (e.g. bone marrow biopsy or aspiration, lumbar puncture) in children, the first 25 patients received midazolam/ketamine and the second 25 propofol with or without morphine [77]. Both regimens were found to be efficacious for procedural sedation, and propofol , with or without morphine, was reported to have a quicker onset of action and a smoother recovery. Despite these advantages, propofol/fentanyl was also found to have significantly more side effects including oxygen desaturations in 36 % vs. 12 % in the midazolam/ketamine group.
From the comparison studies of ketamine, with or without midazolam, vs. propofol with or without an opioid in adults and children for elective and emergent procedures, we can conclude that both regimens have been shown to provide efficacious sedation and analgesia. In addition, patients who receive ketamine, with or without midazolam, may be expected to experience less respiratory adverse events, longer duration of sedation, and more vomiting than those who receive propofol without or without an opioid.
Based on a large body of literature investigating the administration of ketamine and propofol for procedural sedation outside the operating room, both have been shown to be efficacious and safe, with expected, associated adverse events which are readily managed by appropriately trained and experienced providers of sedation [2, 3, 17, 68, 71–74]. Since both have desirable and undesirable clinical effects, it has been proposed that the combination of ketamine and propofol will serve to decrease the total dose required for each drug, potentiate both drugs’ desirable properties, and ameliorate their shortcomings and undesirable qualities [78]. Propofol is an excellent sedative but lacks analgesic properties, while ketamine is a powerful analgesic. The sympathomimetic effects of ketamine will potentially counteract the respiratory depression and hypotension that may occur with propofol. The antiemetic effects of propofol may reduce vomiting commonly seen with ketamine, and emergence phenomena seen with ketamine may be diminished by the hypnotic properties of propofol which has been shown to produce quicker and smoother recovery from sedation. It is also been postulated that the addition of propofol to ketamine will decrease the dose of ketamine required which will result in shorter sedation time and ultimately, shorter lengths of stay [78] (Table 14.3).
Table 14.3
Coadministered adjuncts to ketamine administration
Adjunct drug | Rationale | Evidence of effects |
---|---|---|
Anticholinergics • Atropine • Glycopyrrolate | Believed to decrease hypersalivation associated with ketamine and therefore prevent laryngospasm | • Secretions are decreased with anticholinergics but no clinical benefits have been identified • Increases airway and respiratory adverse events |
Midazolam | Postulated to decrease incidence of ketamine-associated unpleasant recovery reactions | • Decreases unpleasant recovery reactions in adults but not in children • Increases airway and respiratory adverse events • Decreases vomiting |
Ondansetron | Vomiting is common with ketamine and administering an antiemetic will decrease the incidence of vomiting | • Ondansetron decreases vomiting • Most effective in children 5 years of age and older • Also decreases the incidence of post-discharge vomiting |
Propofol | “Synergistic” relationship with ketamine will potentially make sedation more effective and decrease adverse effects | • No difference in objective measures of effectiveness or length of sedation • No difference in airway or respiratory adverse events • Decreases vomiting |
Four observational case series of adults and children who received the propofol/ketamine combination referred to as “ketofol” support its efficacy and low rate of manageable adverse events when administered in the emergency department for painful procedures [79–82]. Three of these studies used a propofol to ketamine ratio of 1:1, while one administered propofol/ketamine at a 2:1 concentration.
The question remains whether ketofol provides clinical benefits over ketamine (with or without midazolam) or propofol (with or without an opioid). David and Shipp performed a randomized controlled trial of ketamine/propofol vs. propofol alone for emergency department procedural sedation of healthy children and adults [83]. Propofol/ketamine ratio in this study was 2:1, and the combination of ketamine and propofol did not reduce the incidence of respiratory depression but resulted in greater provider satisfaction, less propofol administered, and subjectively better sedation quality. Shah et al. performed a blinded, randomized controlled trial of ketamine/propofol vs. ketamine alone for procedural sedation in children [84]. Ketamine/propofol patients received an initial IV bolus dose of ketamine 0.5 mg/kg and propofol 0.5 mg/kg, followed by propofol 0.5 mg/kg and saline solution placebo every 2 min, titrated to deep sedation. Ketamine patients received an initial IV bolus dose of ketamine 1.0 mg/kg and intralipid placebo (to ensure blinding), followed by ketamine 0.25 mg/kg and intralipid placebo every 2 min, as required. Sedation efficacy and airway complications were similar between groups, while the ketamine/propofol combination produced slightly faster recoveries with less vomiting (2 % vs. 12 %) and higher provider satisfaction scores than those in the ketamine alone group [84] (Table 14.3).
Two additional randomized, controlled trials comparing ketofol to propofol for procedural sedation are worth noting. Andolfatto et al. enrolled patients 14 years of age and older comparing a 1:1 mixture of ketofol to propofol alone for emergency department procedural sedation and analgesia [85]. In this study, ketofol was not found to reduce the incidence of adverse respiratory events as compared to propofol alone, and induction time, efficacy, and sedation time were similar in each group. Subjectively, sedation depth appeared to be more consistent with ketofol [85]. Miner et al. administered ketofol at 1:1 and 4:1 propofol/ketamine concentration ratios which were compared to propofol alone for emergency department deep procedural sedation in adults [86]. The authors found a similar frequency of airway and respiratory adverse events leading to intervention between propofol alone and either 1:1 or 4:1 ketofol. The authors conclude that ketofol, at either concentration, and propofol appear to be similarly safe and effective [86].
When compared to ketamine , patients who receive ketofol experience less vomiting and slightly shorter time of sedation with subjectively better sedation. As compared to propofol, ketofol performed very similarly at 1:1, 2:1, and 4:1 propofol to ketamine concentrations with regard to efficacy and complications of sedation. Importantly, ketofol was not found to improve the efficacy of sedation or decrease the incidence or severity of respiratory adverse events when compared to either propofol or ketamine. It is not clear whether ketofol provides any objective benefit over propofol. The objective benefits of ketofol over ketamine alone would appear to be limited to decreased vomiting and length of sedation which must be balanced with the increased risk of respiratory suppression. Additional studies are needed to clarify the advantages and disadvantages of ketofol procedural sedation as compared to ketamine or propofol [87].
As demonstrated in extensive investigations, the dissociative properties of ketamine make it an excellent choice for sedation outside the operating room for a wide variety of painful, elective, and emergent procedures. Patients who receive ketamine at recommended doses and routes of administration will have a predictable length of dissociation with typically manageable associated adverse effects. The serious airway and respiratory adverse events apnea and laryngospasm will occur in less than 1 % of children who receive IV ketamine and are usually managed effectively with brief bag-mask positive pressure ventilation [2].
Psychotomimetic Effects
Domino and Corssen reported the first pharmacologic and clinical experience with the phencyclidine derivative CI-581 (ketamine) in humans [14, 15]. In the pharmacologic study, they report the effects of CI-581 on 20 male volunteers from a prison population. The results of this study were consistent with the ketamine-associated sympathomimetic effects and transient respiratory depression with minimal clinical significance discussed previously. Most of the subjects showed changes in mood, body image and affect, and some reported vivid dreams and/or hallucinations. However, these effects were found to be short lasting and that some of the subjects experienced no undesirable psychotomimetic effects at all [14]. In their clinical study, 130 patients, ranging in age from 6 weeks to 86 years, received CI-581 for a variety of surgical procedures. Again, adverse cardiorespiratory events were minimal, and some of the adult patients had vivid dreams or frank hallucinations during recovery. The dreams lasted 5–15 min and were described by patients as ranging from amusing and pleasant to frightening. Their dreams frequently involved outer space and some patients experience frank hallucinations. Two middle-aged patients were reported to have displayed signs of schizoid behavior , and both experienced “traveling in outer space and thought that they had died and were flying to hell.” In one of these patients, the experience lasted only a few minutes, but the other was affected for 40 min [15].
Adults emerging from the ketamine-induced dissociative state may experience psychotomimetic effects which have been described as schizophrenia-like symptoms and behaviors [5, 21]. Patients describe out-of-body experiences, floating sensations, vivid dreams, hallucinations, delusions, illogical thinking, agitation, disturbances of emotion and affect, decreases in memory, and occasionally, frank delirium [1, 4–6, 14–16, 19–21]. As ketamine acts to inhibit NMDA receptor binding of glutamate and functionally dissociate or disconnect the thalamo-neocortical and limbic centers of the brain, higher centers are prevented from normally perceiving visual, auditory, or painful stimuli [6, 16, 19]. This leads to a misperception or misinterpretation of auditory and visual stimuli which serves to explain many of the dreaming, floating, and out-of-body or flying sensations described by patients [5]. In preverbal and young or developmentally delayed children, who are unable to articulate the feelings they experience due to the psychotomimetic effects of ketamine, agitation may be the primary behavior seen [40].
The transient schizophrenia-like psychotic state that results from NMDA antagonist drugs like phencyclidine and ketamine has inspired Olney and others to investigate the potential role of the NMDA glutamate receptor system in schizophrenia [88]. They postulate that NMDA receptor blockade and subsequent hypofunction result in excessive release of excitatory transmitters (glutamate) and, consequentially, overstimulation of postsynaptic neurons. The result of this work is that pharmacologic models of schizophrenia now rely on glutamatergic rather than dopaminergic dysfunction to explain the symptoms of schizophrenia [89]. The glutamatergic dysfunction mechanism provides an explanation for the associated cognitive and behavioral disturbances seen with NMDA antagonists and in schizophrenia. Due to the similarity of the mechanism of action of ketamine and the pathologic process of schizophrenia, it is recommended to avoid using ketamine in patients with schizophrenia, and caution is advised in patients with other forms of psychosis [17, 21]. Ketamine may be administered to children with attention deficit and hyperactivity disorders (ADHD) as these conditions do not appear to increase the incidence or susceptibility to psychotomimetic effects [21].
Ketamine-induced psychotomimetic effects have been referred to by many names including emergence phenomena [16, 20, 21, 40, 90], emergence reactions [5, 91], emergence delirium [92], and recovery agitation [3, 93–95]. Lack of consistency in terms and definitions has led to a wide range in reporting the incidence of ketamine-induced psychotomimetic effects as some terms and definitions include pleasant dreams and euphoria, while others report only unpleasant reactions. To address the problem of inconsistent definitions and reporting of adverse events associated with procedural sedation, Bhatt led a panel of sedation researchers and experts in developing consensus-based recommendations for standardizing terminology and reporting of adverse events associated with procedural sedation and analgesia for children in the emergency department [96]. Since clinicians and families are most concerned about dysphoria, agitation, and adverse psychotomimetic effects of drugs, the consensus panel proposed the term “unpleasant recovery reactions” which they defined as “abnormal patient affect or behaviors during the recovery phase that requires additional treatment and a change or delay in patient discharge.” The behaviors include one or more of the following:
- (a)
Crying—Inconsolable
- (b)
Agitation—Restless, continuous activity
- (c)
Delirium—State of severe confusion
- (d)
Dysphoria—Inappropriate mood of sadness
- (e)
Nightmares—Unpleasant dreams
- (f)
Hallucinations—Responds to sensory phenomena (i.e., seeing, hearing, or feeling) that are not physically present [96]
Although these guidelines were written to pertain to pediatric sedation research in the emergency department, as one editorial noted, “…despite the pediatric intent, each element applies readily to adults. The approach and the principles put forth also extrapolate to any setting…” [97].
The incidence of psychotomimetic effects associated with ketamine sedation in adults has been reported to have a wide range (partially due to inconsistent definitions and reporting) from less than 5 % to greater than 50 % [5, 16, 91]. In children, the incidence is believed to be significantly lower. One large, individual patient data meta-analysis of 8282 children found the incidence of recovery agitation to be 7.6 % with only 1.4 % of children experiencing a reaction that was felt to be clinically important (i.e., occurrences of abnormal behavioral responses that either led to specific treatment or were specially described by investigators as demonstrating substantial severity) [3].
Original reports found emergence reactions to be more common in patients over 16 year of age, in females than males, in subjects who normally dream, in those who received large doses of ketamine (>2 mg/kg IV) and rapid IV administration (>40 mg/min), and in patients with a history of personality problems [5, 19]. More recent studies have found unpleasant recovery reactions to not be related to age in children [3, 98] and to be infrequent and mild in patients aged 16–21 years [94]. Preprocedural agitation has been shown to increase the incidence of concerning recovery behaviors in children who receive procedural sedation in the emergency department as well as those who receive general anesthesia [93, 99] (Table 14.2). When ketamine was compared to fentanyl/midazolam for emergent procedural sedation, no difference in the incidence of associated unpleasant recovery reactions and post-discharge behavior problems was detected [21, 70, 90].

Full access? Get Clinical Tree
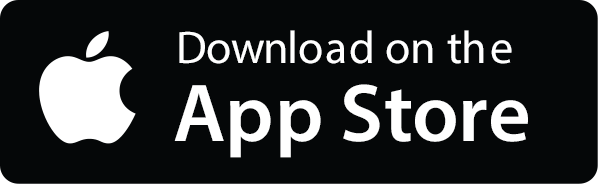
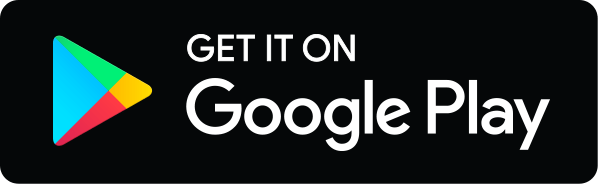