Chest Radiographic Examination
Cynthia B. Umali*
Jerry P. Balikian
*Deceased
Radiographic examination of the critically ill patient in the intensive care unit (ICU) or coronary care unit (CCU) is often necessary to evaluate clinical status. In this setting, the basic role of radiology is to follow the patient’s progress or changes in status after admission or after surgery; the primary diagnosis has been already established. Radiographic examinations are thus requested to evaluate the course of the primary disease and to diagnose complications that may ensue. Henscke et al. [1] studied the diagnostic efficacy of bedside chest radiographs and found that in 65% of the 1,132 consecutive radiographs analyzed, there were new findings or changes affecting patient management. Bekemeyer et al. [2], after analyzing 1,354 radiographs from a respiratory ICU, found a 34.5% incidence of new or increased abnormalities or tube or catheter malpositions. They concluded that routine morning radiographic examinations frequently demonstrated unexpected or changing abnormalities, many of which prompted changes in diagnostic management. The American College of Radiology established the appropriateness criteria for the need of ICU studies [3] and Trotman-Dickenson detailed the role of radiology in the ICU [4,5].
Critically ill patients in the ICU or CCU often cannot take advantage of numerous radiologic modalities that are readily available to mobile patients. Because these patients cannot be transported while their circulatory functions are labile and they are connected to electrocardiogram monitors, ventilators, catheters, and surgical appliances, usually one is left with the portable bedside radiographic examination. Most often, it is a chest examination that is needed; the chest film is especially important because physical examination to determine the presence of a complication such as atelectasis, pneumothorax, pneumonia, or pulmonary edema is difficult in the presence of a ventilator.
Until recently, portable radiographic examinations were restricted by inherent machine limitations in kilovoltage, milliamperage, and radiograph tube currents and by variations in battery charge. The need for adequate penetration to see line and catheter positions necessitated increasing normal exposure time (thereby increasing motion unsharpness) and using a higher kilovoltage (thereby increasing scatter radiation, which increases film fogging). A high kilovoltage also reduces subject contrast. These alterations and limitations cause deterioration of the image, often rendering the film of suboptimal quality for evaluation of subtle changes in the lung parenchyma. During the past few years, most of the above problems have been
practically eliminated with the use of state-of-the-art computed radiography.
practically eliminated with the use of state-of-the-art computed radiography.
Interpretation of portable examinations is fraught with pitfalls. Magnification of the cardiac silhouette cannot be eliminated because of the short tube-film distance and the often supine position of the patient. Signs used to evaluate postcapillary (pulmonary venous) hypertension are not valid on the supine film and may necessitate use of a horizontal beam (cross-table lateral view) to visualize the discrepancy between the dependent and nondependent vessels, which is far more difficult.
Films are often taken after a poor inspiratory effort because of the patient’s inability to cooperate. Unless the type of respirator, phase of cycle, and pressure setting are indicated on the film, the appearance of parenchymal abnormalities is difficult to evaluate. Increased inflation of the lung may cause the opacities to appear less dense, but the apparent improvement secondary to increased aeration does not correspond to a true anatomic improvement. The reverse situation can occur as well.
A portable C-arm fluoroscope is often used at the bedside to monitor catheter placement (especially Swan–Ganz). The fluoroscope also can be used to evaluate alignment of fracture fragments during closed reduction and to visualize diaphragmatic motion. Portable ultrasound equipment is particularly useful for detecting fluid collections, including effusions (pericardial and pleural) and subdiaphragmatic abscesses. Portable gamma cameras are useful for evaluating possible pulmonary embolism in these patients.
With PACCS systems in many ICUs, digital images are now available on ICU monitors immediately after the images are taken.
Evaluation of Tubes and Catheters
Endotracheal Tubes
The location of endotracheal tubes should be checked as soon as possible after insertion (see Chapter 1). To evaluate the position of the tube properly, Goodman et al. [6] showed that one must evaluate the head and neck position simultaneously because tube position can change with flexion and extension of the neck [7] by as much as 4 cm. Thus, to ensure that the tip of the tube is above the carina, one should follow these guidelines:
When the inferior border of the mandible is at or above C4, the tip should be 7 ± 2 cm from the carina.
When the inferior border of the mandible is at the C5-C6 level, the tip of the tube should be 5 ± 2 cm from the carina.
When the inferior border of the mandible is at T1 or below, the tip of the tube should be 3 ± 2 cm from the carina.
When the tube is too high, it may slip into the pharynx. If it is just below the vocal cords, its inflated cuff can cause glottic or subglottic edema, ulceration, and, ultimately, scarring. If it is too low, it can enter a bronchus and cause atelectasis of the lung supplied by the obstructed bronchus (Fig. 63.1).
Ideally, the tube should be one-half to two-thirds the width of the trachea, and the inflated cuff should fill the trachea without causing the lateral walls to bulge. When the ratio of the cuff diameter to the tracheal lumen exceeds 1.5%, tracheal damage is likely to result [8]. Ravin et al. [9] observed that repeated overdistention of the cuff on chest film, despite careful cuff inflation to the minimal leak level, should lead to suspicion of tracheomalacia (Fig. 63.2).
Immediately after intubation, and especially after difficult intubation, a film should be obtained to define the position of the tube. The radiologist should also look for signs of perforation of the pharynx, such as marked subcutaneous emphysema, pneumomediastinum, and pneumothorax. Dislodging of teeth, dental caps, and portions of dentures into the tracheobronchial tree has been reported after intubation. If this is suspected, a foreign body in the tracheobronchial tree should be carefully sought.
Tracheostomy Tubes
The tip of the tracheostomy tube should be located one-half to two-thirds of the way between the stoma and the carina. Unlike the endotracheal tube, the tracheostomy tube does not change position with flexion and extension of the neck. The tracheostomy tube should be evaluated to determine its inner diameter (which should be two-thirds that of the tracheal lumen); its long axis (which should parallel the tracheal lumen); the location of its distal end (Fig. 63.3) (which should not abut the tracheal wall laterally, anteriorly, or posteriorly); and for development of increasing pneumothorax, pneumomediastinum, or subcutaneous emphysema, which may require immediate attention.
Central Venous Catheters
Central venous catheters should be evaluated to ensure that the true central venous pressure is measured. The catheter should be located beyond the venous valves, the most proximal of which is just distal to the junction of the internal jugular vein and the subclavian veins. This is found at approximately the level of the first anterior rib [10] (Fig. 63.4) (see Chapter 2).
Brandt et al. [11] found that the distance to the junction of the superior vena cava and the right atrium is usually the total of the distance from the cutdown site to the suprasternal notch plus one-third the distance from the suprasternal notch to the xiphoid process. Complications of central venous catheter lines include vascular perforation or dissection (Fig. 63.5A,B) and cardiac perforation, leading to cardiac tamponade (Fig. 63.5C), embolization, and infection.
Swan–Ganz Catheters
Swan–Ganz catheters are used to perform right heart catheterizations [12]. Ideally, the tip of the Swan–Ganz catheter should be located in the right or left branch of the pulmonary artery. Occasionally, the tip may be malpositioned (Fig. 63.6); a film should be routinely taken to check its position. If it is more distal to the above location, the catheter may produce pulmonary infarction (Fig. 63.7) by blocking the artery directly or from a clot in or around the tip. Other rare complications include perforation of the pulmonary artery, the resulting focal hemorrhage leading to formation of “traumatic pseudoaneurysm” (Fig. 63.7D), balloon rupture, and pulmonary artery–bronchial tree fistulas.
Intra-Aortic Counterpulsation Balloons
The intra-aortic counterpulsation balloon (IACB) was designed to improve cardiac function in a setting of cardiogenic shock [13], and this remains the major indication for its use. Ideally, the tip of the IACB should be positioned at the level of the aortic arch just distal to the origin of the left subclavian artery to augment coronary perfusion maximally without occluding the subclavian and cerebral vessels (Fig. 63.8). Complications from IACBs are major vessel obstruction, embolization from a clot formed in or around the catheter, and aortic dissection with balloon rupture.
As with endotracheal tubes, the position of the IACB changes with a change in patient position, moving cephalad 1.0 to 4.5 cm when the patient moves from a recumbent to a sitting position [14]. The position, therefore, should be checked periodically.
Chest Tubes
Chest tubes (thoracostomy or pleural drainage tubes) are used to drain either fluid or air from the pleural space (see Chapter 8). If placed for a pneumothorax, the tube should be seen in the anterosuperior position as the air collects beneath the sternum; if placed to drain a pleural effusion, the tube should be seen in the posteroinferior position. To ascertain that the tube is in the pleural space, one must see opaque and nonopaque sides
of the tube. When the nonopaque side is not seen, it is because the subcutaneous tissue, which is similar to the tube in density, has silhouetted this nonopaque border and the tube is outside the pleural space [15]. The side hole of the tube (where there is a break in the opaque marker) also should be seen within the pleural space.
of the tube. When the nonopaque side is not seen, it is because the subcutaneous tissue, which is similar to the tube in density, has silhouetted this nonopaque border and the tube is outside the pleural space [15]. The side hole of the tube (where there is a break in the opaque marker) also should be seen within the pleural space.
Nasogastric Tubes
The tip of the nasogastric tube and the side hole should be visible below the diaphragm within the gastric lumen. A malpositioned NG tube can be identified by its characteristic side hole (Fig. 63.9A,B).
Transvenous Pacemakers
The pacemaker is passed under fluoroscopic guidance to the apex of the right ventricle (see Chapter 5). Films should be checked for breaks or fractures in the wire (Fig. 63.10). A lateral view should be obtained to ascertain that the pacemaker tip is directed anteriorly 3 to 4 mm beneath the epicardial fat stripe [16]. A posteriorly directed tip in the lateral view, coupled with a cephalad direction in the anteroposterior (AP) view,
suggests that the pacer is in the coronary sinus [17]. Projection of the pacemaker tip anterior to the epicardial fat stripe suggests myocardial perforation [16]. Air entrapment in the pulse generator pocket can produce a system malfunction with unipolar pulse generators; this should be kept in mind when examining patients with subcutaneous emphysema [18].
suggests that the pacer is in the coronary sinus [17]. Projection of the pacemaker tip anterior to the epicardial fat stripe suggests myocardial perforation [16]. Air entrapment in the pulse generator pocket can produce a system malfunction with unipolar pulse generators; this should be kept in mind when examining patients with subcutaneous emphysema [18].
Evaluation of the Lung Parenchyma, Pleura, Mediastinum, and Diaphragm
Densities of the Lung Parenchyma
Pulmonary parenchymal densities in the critically ill patient may be caused either by infectious or noninfectious conditions, such as atelectasis, cardiogenic pulmonary edema, acute respiratory distress syndrome (ARDS), pulmonary infarction, or contusion. Radiologic evaluation to determine whether parenchymal densities are secondary to pulmonary edema, other causes, or a combination of edema and other causes is often necessary to complement or initiate a clinical search for pneumonia so that proper therapy can be started.
In 1973, Leeming [19] observed gravitational displacement of edema fluid to the dependent lung. He suggested that pulmonary edema could be differentiated from other causes by a positional shift in the infiltrate. In 1982, Zimmerman et al. [20] evaluated the gravitational shift test and concluded that it is a simple noninvasive method for detecting mobilizable lung water, useful even in the presence of pulmonary damage or an inflammatory process.
After baseline films are obtained, the gravitational shift test is performed, using bedside frontal films. The patient is maintained in a lateral decubitus position for 2 to 3 hours before the films are taken. The hemithorax with fewer parenchymal densities is placed in the dependent position. In 85% of their patients with pulmonary edema, Zimmerman et al. [20] found that the densities in the up lung shifted toward the dependent lung, whereas in 78% of patients with inflammatory disease, no shift was seen.
Evaluation of densities in the retrocardiac area may require an overpenetrated film (Fig. 63.11), a 15- to 30-degree left anterior oblique film, or a right lateral decubitus view. The latter position provides better aeration of the left lung and allows greater visualization of the retrocardiac area. In the presence of pleural effusion, a decubitus view may be necessary to displace the pleural fluid and allow better visualization of the parenchyma.
Congestive Failure and Pulmonary Edema Due to Pulmonary Venous Hypertension
Elevation of pulmonary venous pressure, irrespective of cause, produces a sequence of radiologic findings. When pulmonary venous pressures rise above normal, pulmonary vascular gravitational redistribution occurs [21], producing distention of the upper lobe vessels with a concomitant decrease in caliber of those in the lower lobe in the upright patient. In patients in the supine position, the equivalents of the upper lobe vessels are the anterior or ventral pulmonary vessels and the equivalents of the lower lobe vessels are the posterior or dorsal vessels. The change in caliber of the vessels in the supine position is discernible in a good cross-table lateral film of the chest. These changes are also visible with computed tomography (CT); on a CT, the dorsal vessels become progressively narrower as venous pressure increases.
At pulmonary capillary wedge pressures of 20 to 25 mm Hg, lymphatic drainage is exceeded and the alveolar interstitium, bronchovascular interstitium, interlobular septa, and subpleural tissues become distended with edema fluid. The visible radiologic changes at these pressures are:
Thickening of the interlobular septa (Kerley A and B lines) (Fig. 63.12)
Peribronchial cuffing, in which hairline, well-defined bronchial walls seen on end increase in thickness and lose their sharp definition (Fig. 63.13A,B)
Blurring or haziness of the perivascular outlines (Fig. 63.13A,B)
Thickening of the interlobular fissures (Fig. 63.13A,B)
Widening of the pleural layer over the convexity of the lungs secondary to the presence of fluid in the subpleural space
Pulmonary vascular redistribution (Fig. 63.13C)
Interstitial edema can clear rather rapidly after therapy (Fig. 63.13D). At pulmonary capillary wedge pressures of 25 to 40 mm Hg, edema fluid pours into the alveolar spaces and air space or alveolar edema is seen. The air space consolidation may extend to the subpleural zone, or the more characteristic butterfly or bat-wing edema pattern may be seen (Fig. 63.14).
Unilateral pulmonary edema is probably positional, related primarily to a gravitational shift of mobilizable fluids to the dependent lung [19]. It is postulated that asymmetric edema is often right sided because of cardiac enlargement that impedes
blood flow in the left pulmonary arterial system, thereby reducing capillary volume. Unilateral diminution in pulmonary blood flow, as seen in Swyer–James syndrome, right or left pulmonary artery thromboembolism, and surgical corrections of congenital heart disease (e.g., shunts for tetralogy of Fallot) are other causes of unilateral edema (Fig. 63.15).
blood flow in the left pulmonary arterial system, thereby reducing capillary volume. Unilateral diminution in pulmonary blood flow, as seen in Swyer–James syndrome, right or left pulmonary artery thromboembolism, and surgical corrections of congenital heart disease (e.g., shunts for tetralogy of Fallot) are other causes of unilateral edema (Fig. 63.15).
Atypical patterns of congestive failure and pulmonary edema were described by Hublitz and Shapiro [22] in patients with chronic pulmonary disease. Of the four basic patterns they described, two differ in appearance from pulmonary edema in patients with normal lung compliance and vascularity. An asymmetric regional pattern, in which edema occurs only in zones with adequate vascularity, occurs in these patients. The extent of involvement varies greatly from one segment of the lung to another relative to the state of the vascular bed. Another pattern seen is the miliary nodular pattern. Hublitz and Shapiro [22] postulated that the thick-walled spaces in which thickened fibrous septa replace normal alveolar walls impair collateral ventilation and prevent dispersion of edema fluid throughout the lungs. Fluid is then trapped in relatively larger spaces that have replaced normal alveoli. Shadows produced do not coalesce, and the images are seen on radiographs as miliary nodular patterns. The other two patterns, interstitial and reticular, are also seen without chronic lung disease.
![]() Figure 63.10. Posteroanterior view of the chest in a patient with a malfunctioning pacemaker. A break in the pacer wire (arrow) caused the malfunction. |
Pulmonary edema can be due to cardiac or noncardiac causes. Different radiologic indices distinguish between hydrostatic (cardiac) edema, overhydration pulmonary edema, and edema secondary to increased capillary permeability (see the section Acute Respiratory Distress Syndrome) [23]. In overhydration edema (e.g., edema secondary to renal failure), the cardiac output is large, and, consequently, pulmonary blood flow is large. All vessels are recruited, and no redistribution of flow occurs. Because blood volume is also increased, the
vascular pedicle, azygos vein, and hilar vessels are large. In pure capillary permeability edema, there is no increase in blood volume, and therefore the vascular pedicle and azygos vein remain normal in size; no signs of pulmonary venous hypertension are present, and heart size is also normal. When different types of edema coexist, edema may occur at lower left atrial pressures, and wedge pressure readings may be low or only slightly elevated [24].
vascular pedicle, azygos vein, and hilar vessels are large. In pure capillary permeability edema, there is no increase in blood volume, and therefore the vascular pedicle and azygos vein remain normal in size; no signs of pulmonary venous hypertension are present, and heart size is also normal. When different types of edema coexist, edema may occur at lower left atrial pressures, and wedge pressure readings may be low or only slightly elevated [24].
Acute Respiratory Distress Syndrome
Numerous factors can be responsible for ARDS, but the common denominator is always an acute injury to the alveolocapillary unit. The pathologic alterations with corresponding radiologic changes occur 12 to 24 hours after the first appearance of respiratory symptoms. Insidious accumulation of edema fluid in the extravascular space occurs. This appears to be confined to the true unrestricted interstitial space, in which the basal laminae of the epithelium and endothelium are separated, and does not appear in the restricted interstitial space with fused basal laminae [25].
The corresponding radiologic picture is a perihilar, perivascular haziness with peribronchial cuffing. Only occasionally are Kerley A and B lines seen; in one series, they were noted in only 5 of 75 cases [26]. During the acute stage, the alveoli also become nonhomogeneously filled with a proteinaceous and often hemorrhagic cell-containing fluid. Hyaline membranes form in
the alveoli and sometimes in the alveolar ducts. The radiologic picture is one of patchy, ill-defined, confluent miliary nodular or alveolar densities that are not rapidly reversible (Fig. 63.16).
the alveoli and sometimes in the alveolar ducts. The radiologic picture is one of patchy, ill-defined, confluent miliary nodular or alveolar densities that are not rapidly reversible (Fig. 63.16).
![]() Figure 63.14. Alveolar pulmonary edema. Butterfly pattern of pulmonary edema can be seen in the perihilar areas. |
The course of ARDS is highly variable. In some patients, reabsorption of the exudates is complete within a few days, thereby producing radiologic clearing of the densities. In some, there is a delayed clearing of the exudates, with a corresponding delay in clearing of the radiologic picture. In a third group, progressive fibrosing alveolitis follows. The progression of fibrosis and the degree of tissue derangement do not correlate with the duration of the disease. Radiologically, this phase presents a diffuse, fibrotic pattern.
After the first week, the radiologist’s main concern is the recognition of superimposed complications, such as pulmonary infections, oxygen toxicity, barotrauma, and pulmonary embolism with infarction. When clinical signs and symptoms of infection are present and the radiographic picture deteriorates, pneumonia should be suspected. Development of cavities and a change in the character of the densities should lead to suspicion of superimposed abscess, infarction, or cardiac failure. Unger et al. [27] showed that only direct hemodynamic measurements of the pulmonary capillary wedge pressure provide a dependable means of detecting superimposed failure in cases of
ARDS. Pulmonary embolism, with or without infarction, can be verified with a pulmonary arteriogram using the Swan–Ganz catheter, already in place in most cases, to inject the contrast material.
ARDS. Pulmonary embolism, with or without infarction, can be verified with a pulmonary arteriogram using the Swan–Ganz catheter, already in place in most cases, to inject the contrast material.
Atelectasis and Pneumonia
Atelectasis is easily diagnosed when a characteristic linear density or large densities are seen with accompanying signs suggestive of volume loss (shift of fissures or mediastinal and diaphragmatic elevation, or both). Densities that fall between these categories, however, such as patchy infiltrates, are often indistinguishable from pneumonia on a single study.

Full access? Get Clinical Tree
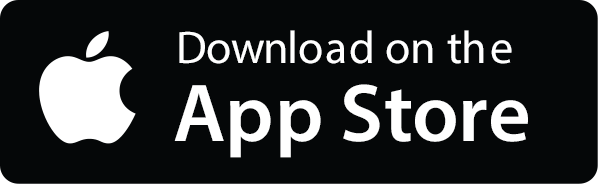
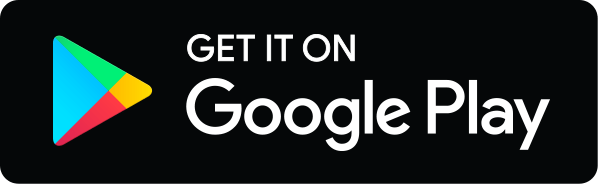