Introduction
Surgery has become one of the most important medical interventions available, with 200 million patients undergoing surgery with anesthesia each year; this represents an increase of 30% over the last 10 years (1). Approximately one-third of all hospital admissions are for emergency or elective surgery and, with increasing life-span and growing populations, the number of surgical procedures is likely to continue to increase. The practice of modern surgery relies heavily upon anesthesia, enabling patients to tolerate surgical interventions that would, in normal circumstances, result in unbearable agony, potentiate extreme physiological exacerbations, and inflict unpleasant memories and perhaps a form of posttraumatic stress disorder (PTSD).
Despite the dramatic improvements in modern surgery and anesthesia in recent years, our understanding of common procedures such as coronary artery bypass grafts, which offer remarkable health benefits for the population, continues to evolve. Reports of alarming postsurgical neurodegenerative complications including postoperative cognitive decline (POCD), Alzheimer’s disease (AD) and Parkinson’s disease have been described in the recent literature. It has been hypothesized that these complications are due to a malignant systemic inflammatory response following surgery that ultimately results in neuroinflammation, synaptic impairment, widespread neurodegeneration, and ultimately cognitive dysfunction (2). The pathogenesis of these complications remains unclear and further studies are urgently needed to identify the precise pathological processes and etiological factors responsible in these complications. This may provide the basis for the development of interventions to prevent patients from suffering harmful postsurgical neurodegenerative sequelae.
Surgical Trauma and Inflammation
Patients subjected to major surgery often exhibit an acute-phase inflammatory response that is clinically characterized by fever, drowsiness, and anorexia. Mechanical injury is an inevitable product of any surgical procedure, resulting in activation of the innate immune system and a subsequent inflammatory response. This process occurs as part of the body’s inherent protective reaction to trauma in any part of the body and is responsible for the clinical features seen in many patients following major surgery.
Following surgical trauma, a variety of leukocytes including macrophages and monocytes are activated, mobilized, and migrate to sites of tissue injury. This response occurs even in the absence of surgical site infection and intensifies the local inflammatory processes via the release of signaling molecules, such as prostaglandins and nitric oxide (NO), and the generation of pro-inflammatory cytokines including interleukin-6 (IL-6), tumor necrosis factor (TNF)-α, and interferon (IFN)-γ. Further biochemical and cellular features include complement activation, leukocytosis, and lymphopenia, as well as activation of hepatic acute-phase proteins and other metabolic disturbances.
Development of Systemic Inflammation
While the early local inflammatory response following surgical trauma is designed to limit the initial injury and prevent spread, when the response becomes uninhibited this results in a well-documented disorder known as systemic inflammatory response syndrome (SIRS). It is important to note that, as well as surgical trauma, SIRS can also occur as a result of infection, burns, pancreatitis, ischemia, and hemorrhage. SIRS can be divided into different stages depending on its severity, as determined by Roger Bone (1997) who produced an interesting paradigm to explain the general pathogenesis of SIRS following infection and trauma (3)(Table 9.1). Clinically, SIRS is defined as two or more of the following features (4):
fever of more than 38°C or less than 36°C (hyper- or hypothermia)
heart rate of more than 90 beats/min (tachycardia)
respiratory rate of more than 20 breaths/min (tachypnoea) or PaCO2 less than 32mmHg (hypocapnia)
abnormal white blood cell count >12,000/µL or <4000/µL (leukocytosis or leukopenia)
In some patients this severe inflammatory process is dampened and down-regulated; however, in many others it persists in an uncontrolled fashion. The unregulated inflammatory processes that occur during SIRS can result in complex disturbance of homeostasis, and is associated with significant morbidity and mortality.
Table 9.1 Pathophysiology of the Systemic Inflammatory Response Following Surgical Trauma and Other Disease States
Severe SIRS and Multiorgan Dysfunction
There are thought to be five general mechanisms behind the development of multiorgan dysfunction following SIRS. First, SIRS has a profound vasodilatory effect that results in abnormal distribution of circulating blood volume and subsequent tissue hypoperfusion. Second, the increased vascular permeability leads to the displacement of fluids into the interstitial space and subsequent parenchymal edema. The third contributing factor is disseminated intravascular coagulation, which occurs due to the combination of endothelial damage, increased expression of cell adhesion molecules, and small thrombi in the microcirculation. Fourth, neutrophils produce reactive oxygen species (ROS) and proteases, which result in oxidative damage and contribute to endothelial dysfunction. Finally, activation of inducible nitric oxide synthase (iNOS) results in the increased production of NO, which, in turn, contributes to further inflammation. In addition, NO has the ability to react with superoxide, leading to cell toxicity due to peroxynitrate formation.
Cardiovascular Dysfunction
The cardiovascular system is sensitive to the effects of pro-inflammatory mediators and vasoactive substances that are produced in excess during SIRS. In response to pro-inflammatory cytokines, NO is produced from L-arginine and the action of iNOS. NO is primarily responsible for the profound fall in systemic vascular resistance that is observed in patients with SIRS. The subsequent hypotension is often refractory to conventional treatment with fluids, ionotropes, and vasoconstrictors, resulting in tissue hypoperfusion despite compensatory increases in cardiac output due to the decreased afterload. In fact, independent of the decrease in preload and afterload, intrinsic myocardial depression has been observed within 24 hours of the onset of SIRS. The increased systemic levels of NO also contribute to diastolic dysfunction, whereby the myocardium relaxes abnormally. This ultimately results in a further reduction in cardiac output and tissue perfusion.
Ologunde et al. discusses the processes by which systemic inflammation, involving cytokine induction, leukocyte infiltration, and cell death by apoptosis, results in cardiac dysfunction (5). IL-6, IL-1, and TNF-α have all been implicated in the pathophysiology of heart failure, with higher levels of these cytokines associated with a worsening New York Heart Association (NYHA) classification status (6). Due to the presence of cytokine receptors within the myocardium, it is likely that the cytokine cascade causes myocyte cell death, leukocyte infiltration, and a subsequent reduction in cardiac contractility. Various animal models of ischemic injury have demonstrated a correlation between increased systemic cytokine levels and the presence of distant organ failure.
Respiratory Dysfunction
Pulmonary impairment is a common complication of SIRS and manifests as tachypnoea, hypoxemia, and respiratory alkalosis. In severe cases, it may develop into acute lung injury (ALI) and acute respiratory distress syndrome (ARDS). While the precise pathogenesis of respiratory dysfunction is not fully understood, it is thought to occur due to pulmonary capillary endothelial dysfunction. In turn, this results in interstitial and alveolar edema associated with exudative fluid that is rich in protein and phagocytic immune cells. The presence of increased pro-inflammatory cytokines results in increased permeability within the pulmonary vasculature, resulting in alveolar denudation and basement membrane dysfunction. The increased endothelial permeability is also facilitated by systemic oxidative stress and enhanced leukocyte infiltration within lung parenchyma. These processes also cause increased membrane lipid peroxidation and pulmonary cell lysis. The death of type-1 pneumocytes, which make up 90%–95% of the alveolar surface, due to enhanced inflammatory and oxidative stress is thought to play an important role in the disruption of gaseous exchange and progression toward ALI and ARDS.
Renal Dysfunction
Acute kidney injury (AKI) is a common complication of SIRS and is associated with high rates of morbidity and mortality. AKI has been found to affect one in five patients during hospital admission following a major surgical intervention. However, the diagnosis of postoperative AKI is often delayed and managed suboptimally, resulting in significant clinical sequelae. In fact, recent studies have demonstrated that even small, transient rises in creatinine are associated with increased mortality. Therefore, an improved understanding of postoperative AKI is necessary in order to ensure prompt diagnosis and prevent a decline in renal function.
Prerenal AKI is the most common type in surgical patients. Surgery itself possesses significant risk factors for the development of AKI due to significant fluid losses in the perioperative patient, for example due to perioperative fasting, hemorrhage, and nasogastric tube losses. While the precise mechanisms for the pathogenesis of acute renal failure in SIRS are not fully understood, several mechanisms have been proposed. Autoregulation is a physiological process that is required in order to maintain optimum renal blood flow and glomerular filtration via the delicate regulation of vascular tone in the afferent and efferent arterioles. SIRS causes cytokine-induced systemic vasodilation, relative hypovolemia, and renal hypoperfusion, making it difficult to predict renal blood flow from conventional systemic blood pressure parameters. Due to the vasodilatory effects of cytokines, the kidney responds by producing intrinsic vasoconstrictors, particularly the arachidonic acid metabolites of leukotrienes and thromboxane. These substances reduce renal perfusion and facilitate renal dysfunction. In addition, similarly to the other tissues that are damaged during SIRS, the kidney is also susceptible to leukocyte-mediated parenchymal damage with associated neutrophil aggregation. However, it is important to note that, in contrast to noninfective SIRS, AKI may develop in parallel with increased renal blood flow during endotoxemia.
Neurological Dysfunction
The systemic inflammatory response following surgery is proportional to the extent of surgical insult, while excessive peripheral inflammation also has the potential to cause remote organ dysfunction and directly impact the central nervous system (CNS) (7). Surgical trauma results in activation of the innate immune system in a nuclear factor-κβ (NF-κβ)-dependent manner, resulting in the release of pro-inflammatory cytokines such as TNF-α that ultimately compromise the integrity of the blood-brain barrier (BBB) and allow leukocytes and other inflammatory cells to marginate into the brain. The systemic pro-inflammatory milieu causes sufficient disruption of the BBB and allows deposition of fibrinogen, a 340KDa glycoprotein, following experimental orthopedic surgery in mice. Compromise of the BBB promotes monocyte-derived migration of macrophages into the brain parenchyma, resulting in the intensification of CD11b immunoreactivity that has been identified in the surgical phenotype. In addition, the central inflammatory response following surgical trauma results in the release of danger-associated molecular patterns (DAMPs). DAMPs bind to pattern recognition receptors (PRRs), which are present within the BBB endothelium, causing further activation of pro-inflammatory pathways such as NF-κβ. It is important to recognize that, in the elderly, this is all occurring in the context of an already compromised BBB.
The release of pro-inflammatory cytokines during surgery-induced neuroinflammation also results in the transformation of inactivated microglia, the resident macrophages of the brain, to an activated, phagocytic state; this further propagates the inflammatory response. Microglia have the ability to remain activated for long periods, potentiating the progression toward a state of chronic neurodegeneration. Astrocytes, which are a characteristic star-shaped subtype of glial cells within the CNS, act synergistically with microglia by further contributing to the inflammatory cascade through the release of pro-inflammatory molecules such as TNF-α. It is important to note that the situation is not as binary as it may initially appear due to the dichotomous role of various cytokines that are classically considered as pro-inflammatory. For example, while TNF-α exerts significant pro-inflammatory effects, it has also been found to possess a paradoxical role via the nonapoptotic anti-inflammatory regulation of IL-12 and IL-23 (8). Various other pathways have also been shown to contribute to neurodegeneration and cognitive impairment, such as the cyclooxygenase (COX)-1 pathway that results in excessive microglial-dependent prostaglandin synthesis.
The abundance of pro-inflammatory cytokine receptors in various anatomical locations within the brain, such as the hippocampus, partly accounts for the profound vulnerability of the brain to the presence of systemic cytokines. Interestingly, the BBB may not be the only pathway for pro-inflammatory cytokines and peripheral macrophages to marginate into the CNS. Recent literature has demonstrated the ability for soluble cytokines to also access the CNS via stimulation of afferent C-fibers and subsequently migrate across the blood-spinal cord barrier (9).
Overall, the margination of systemic macrophages, activation of resident cerebral macrophages and the dissemination of pro-inflammatory cytokines from the peripheral circulation into the CNS all contribute to the process of postsurgical neurodegeneration and the morphological and functional consequences of disease.

Full access? Get Clinical Tree
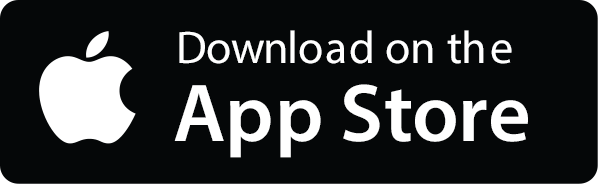
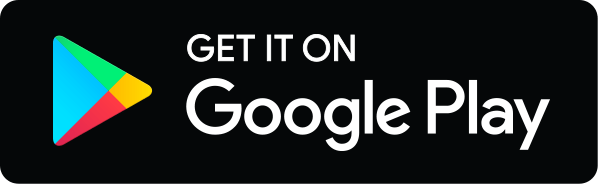
