Abstract
Triiodothyronine (T3), the strongly biologically active thyroid hormone. T3 comprises only 10% of the hormones released by the thyroid gland. In the circulation, T3 is very highly protein bound (99.7%), mainly to albumin, and has a short half-life (24 h). Only the unbound fraction of T3 is able to diffuse into the tissues to exert its effects.
The thyroid gland
Which hormones are synthesised by the thyroid gland?
The thyroid gland is located in the anterior neck and consists of two lobes connected by an isthmus. It secretes two hormones:
Triiodothyronine (T3), the strongly biologically active thyroid hormone. T3 comprises only 10% of the hormones released by the thyroid gland. In the circulation, T3 is very highly protein bound (99.7%), mainly to albumin, and has a short half-life (24 h). Only the unbound fraction of T3 is able to diffuse into the tissues to exert its effects.
Thyroxine (T4), a weakly biologically active hormone, is the main hormone synthesised by the thyroid gland (90%). T4 is also very highly protein bound, but mainly to a specific carrier protein: thyroxine-binding globulin. At 7 days, the half-life of T4 is much longer than that of T3. Around half of the weakly active T4 is converted to the active form, T3, in the peripheral tissues. The other half is converted to an inactive hormone called reverse T3. Bound T3 and T4 provide a large reservoir of thyroid hormone, which delays the onset of symptoms in hypothyroidism.
What are the physiological effects of triiodothyronine?
T3 is able to diffuse across cell membranes to reach the cell nucleus, where it regulates gene transcription. T3 therefore has physiological effects on most tissue types in the body, with the exception of the spleen and the thyroid gland itself. The main physiological effects of T3 are perhaps best illustrated when T3 concentration is either abnormally high or low, as occurs in hyperthyroidism and hypothyroidism, respectively:
Metabolism. T3 affects the activity of a wide range of metabolic processes, such as lipolysis and gluconeogenesis. Hyperthyroidism results in an increase in basal metabolic rate (BMR) and an increased availability of metabolic substrates, such as free fatty acids and glucose. In hypothyroidism, the opposite occurs.
Growth and development. Hypothyroidism in childhood causes growth retardation. T3 is especially important in the development of the central nervous system (CNS), where it stimulates neuronal myelination and nerve axon growth.
Respiratory system. In hyperthyroidism, O2 consumption and CO2 production are increased due to the increase in BMR. In turn, V̇E increases.
Cardiovascular system. In hyperthyroidism, T3 increases the number of β-adrenergic receptors in the heart. The result is an increase in heart rate (HR) and myocardial contractility, leading to an increase in cardiac output.
CNS. T3 has an important effect on mood: in hypothyroidism, depression and psychosis may occur, whilst hyperthyroidism is associated with anxiety.
Musculoskeletal system. In hyperthyroidism, T3 induces protein catabolism, which predominantly affects proximal muscles, resulting in proximal myopathy.
How are triiodothyronine and thyroxine synthesised?
The thyroid gland is made up of multiple follicles: spheres of follicular cells surrounding a core of thyroglobulin, a large protein containing many tyrosine residues. T3 and T4 are synthesised as follows (Figure 81.1):
Iodide (I‾) uptake. I‾ is actively transported from the circulation to the follicular cells through a Na+/I‾ co-transporter. As a result of this secondary active transport, 25% of the body’s I‾ is stored within the thyroid. The uptake of I‾ is stimulated by thyroid-stimulating hormone (TSH). I‾ diffuses through the follicular cell and into the follicular lumen.
I‾ oxidation. As I‾ is relatively inert, it must be oxidised to the more reactive iodine (I2) by thyroid peroxidase involving hydrogen peroxide (H2O2), a reaction that is promoted by TSH.
I2 reaction with tyrosine. Once synthesised, I2 reacts with the tyrosine residues of the surrounding thyroglobulin protein. Tyrosine may be iodinated at one or two positions, resulting in mono-iodotyrosine (MIT) or di-iodotyrosine (DIT), respectively.
Oxidative coupling. Two of the iodinated tyrosine molecules are then coupled together. If two DIT molecules join, the resulting compound is T4; if MIT is coupled to DIT, the result is T3. This oxidative coupling of tyrosine residues is promoted by TSH.
The end result of this process is small T3 and T4 molecules dispersed throughout the large thyroglobulin protein.1 It is estimated that the thyroid gland contains a sufficient store of T3 and T4 to meet the body’s requirements for 1–3 months. In response to TSH, droplets of thyroglobulin are endocytosed by the follicular cells. Within the follicular cell, T3 and T4 are separated from thyroglobulin and released into the circulation.
How is the plasma concentration of thyroid hormones regulated?
TSH has multiple roles in both the synthesis and release of thyroid hormones. TSH is released by the anterior lobe of the pituitary gland in response to hypothalamic secretion of thyrotropin-releasing hormone (TRH; see Chapter 80). In turn, TRH release is controlled through a negative-feedback loop: T3, the biologically active thyroid hormone, inhibits the release of TRH at the hypothalamus. T3 is highly protein bound; only the unbound fraction is able to inhibit the hypothalamus.
Disturbances in the hypothalamic–pituitary–thyroid axis usually result from thyroid gland dysfunction:
Hypothyroidism most commonly results from Hashimoto’s thyroiditis, an autoimmune disease of the thyroid gland in which antibodies are directed against thyroid peroxidase or thyroglobulin. The result is a reduction in T3 and T4 secretion. In response, the hypothalamus and anterior lobe of the pituitary gland increase their secretion of TRH and TSH, respectively; a high measured TSH normally implies hypothyroidism.
Hyperthyroidism is most commonly due to Graves’ disease, an autoimmune condition that results in increased synthesis and secretion of T3 and T4. The hypothalamus and anterior lobe of the pituitary gland respond by decreasing their secretion of TRH and TSH. A low measured TSH normally implies hyperthyroidism.
What is Graves’ disease?
Graves’ disease is an autoimmune disease: autoantibodies stimulate TSH receptors in the thyroid gland, causing excessive synthesis and release of T3 and T4. The clinical effects of Graves’ disease can be divided into:
Those due to hyperthyroidism. Symptoms include palpitations, heat intolerance, weight loss despite increased appetite, fine tremor, diarrhoea and excessive sweating. Clinical signs include sinus tachycardia, atrial fibrillation, lid lag and a smooth, diffusely enlarged thyroid gland (a ‘goitre’).
Those caused by the autoantibodies. Graves’ eye disease is caused by the same TSH receptor autoantibodies that also target fibroblasts in the extraocular muscles. The resulting inflammation causes exophthalmos (upper lid retraction), proptosis (bulging eyes) and conjunctivitis.
What are the management options in Graves’ disease?
There are three treatment options in hyperthyroidism:
Anti-thyroid drugs. Carbimazole and propylthiouracil mainly act by inhibiting the thyroid peroxidase-catalysed oxidation of I‾ to I2. Without I2, the iodination of tyrosine cannot occur.
Radioiodine. As discussed above, I‾ is actively concentrated in the thyroid gland. Likewise, radioactive iodine (131I) is also actively taken up by the thyroid gland, where the β-radiation emitted causes damage and necrosis of thyroid tissue.
Surgery. Total thyroidectomy is a permanent solution to hyperthyroidism, but carries additional risks: recurrent laryngeal nerve injury, parathyroid gland damage and post-operative haematoma, which may cause airway obstruction.
All of the above options (usually) render the patient hypothyroid: T4 replacement is therefore required. None of the above options have any effect on Graves’ eye disease. As it is mediated by autoantibodies, severe Graves’ eye disease may be treated with corticosteroids or by surgical debulking.
Clinical relevance: anaesthesia for thyroid surgery
There are many indications for thyroid surgery: thyroid malignancy, hyperthyroidism and goitre with associated complications, such as tracheal compression. Anaesthesia for thyroid surgery may be particularly challenging owing to the proximity of the thyroid gland to the trachea.
Prior to thyroid surgery, the patient should be rendered euthyroid by one of the medical methods above. In addition to the usual clinical assessments of the airway, a computed tomography scan of the neck may be used to assess the size and position of any goitre. It is also important to identify compression or invasion of the structures surrounding the thyroid gland: the trachea (resulting in stridor), superior vena cava (obstruction), sympathetic chain (Horner’s syndrome) and recurrent laryngeal nerve (hoarse voice).
Induction of anaesthesia may require a gaseous or awake fibre-optic technique if the trachea is compressed or the anatomy significantly distorted. Occasionally, the only airway option is a tracheostomy performed under local anaesthesia. Intraoperative electrophysiological testing of the recurrent laryngeal nerve may preclude the use of muscle relaxants following induction – a remifentanil infusion is therefore commonly used.
A number of serious post-operative complications may occur:
Haemorrhage, which can cause tracheal compression and rapid airway obstruction. Any suspicion of developing airway obstruction warrants an urgent surgical review and removal of the surgical clips – a clip remover should be kept at the patient’s bedside.
Recurrent laryngeal nerve palsy, which may be due to surgical retraction or transection and may be unilateral or bilateral. Bilateral recurrent laryngeal nerve palsy results in complete adduction of the vocal cords and therefore complete airway obstruction. Immediate reintubation is required and a tracheostomy may be necessary.
Severe hypocalcaemia – the parathyroid glands may be inadvertently damaged or excised during thyroid surgery, resulting in hypocalcaemia. The signs and symptoms of hypocalcaemia are discussed below. Of note: severe hypocalcaemia may result in laryngospasm.
Tracheomalacia, characterised by flaccid tracheal cartilage that collapses on inspiration, resulting in airway obstruction. Patients who have long-standing or very large goitres are at much greater risk of tracheomalacia. Prior to extubation, it is useful to deflate the endotracheal tube cuff to check for air leak.
Regulation of calcium homeostasis
What are the physiological functions of calcium?
Ca2+ has numerous biological roles, the most important of which are:
Structural – calcium phosphate gives bone its rigidity.
Haemostasis – Ca2+ is an essential cofactor in the coagulation cascade. Blood samples are prevented from clotting through the addition of EDTA or citrate, which irreversibly binds Ca2+ (see Chapter 72).
Resting membrane potential (RMP) – extracellular Ca2+ concentration affects the cell membrane Na+ permeability, which in turn affects the RMP of excitable cells. Hypocalcaemia acts to depolarise the cell membrane towards the threshold potential. Thus, nerves may undergo spontaneous depolarisation, resulting in tetany (see Chapter 52). Clinical signs of hypocalcaemia include Trousseau’s sign (carpal spasm following inflation of a blood pressure cuff) and Chvostek’s sign (inducing facial spasm when tapping over the zygoma).
Neurotransmitter release – Ca2+ influx into the terminal bouton triggers the exocytosis of vesicles filled with neurotransmitter into the synaptic cleft (see Chapter 53).
Excitation–contraction coupling – Ca2+ influx into skeletal, cardiac or smooth muscle is essential for the binding of myosin to actin (see Chapters 54, 56 and 57).
Cell signalling – Ca2+ is an important second messenger. For example, G proteins that act via inositol triphosphate use Ca2+ as the intracellular messenger.

Full access? Get Clinical Tree
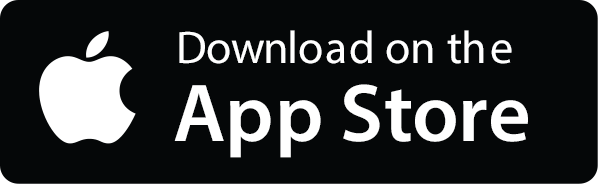
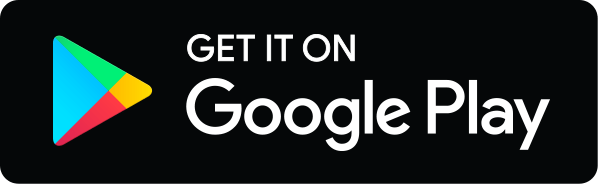
