Chapter 5 Third Pillar of PBM—Harnessing and Optimization of the Patient’s Physiological Tolerance to Anemia
5.1 Perioperative Optimization of the Anemia Tolerance
5.1.1 Tolerance to Low Hemoglobin Values
Throughout the evolutionary history of Homo sapiens, the primary effect of acute bleeding was hypovolemia with no decline in the hemoglobin concentration, because blood loss could only be compensated secondarily through the influx of intracellular and interstitial fluid into the vascular system. However, in 1866 the physiologist Hugo Kronecker observed that patients with hypovolemia, hitherto fatal, were able to survive on infusion of a saline solution; with this discovery, artificial volume expanders such as crystalloids and colloids gained their present-day significance in medicine (Kronecker 1886). Although the use of such solutions to compensate for blood loss is a very modern phenomenon in terms of the evolutionary history, the human body is in most cases capable of compensating dilutional anemia resulting from intravenous volume expansion. There are case reports of patients who survived surgery with almost no circulating oxygen carriers (Dai et al 2010). The fact that nonphysiological low hemoglobin concentrations can be compensated if normovolemia is maintained makes it possible to dispense with the immediate transfusion of red blood cell (RBC) concentrates in cases of bleeding.
There are widespread inter- and intraindividual variations in the ability to tolerate low hemoglobin values. Depending on a patient’s underlying diseases and cardiac reserve and on the specific clinical situation, very different hemoglobin concentrations can still be compensated or will lead to tissue hypoxia with subsequent injury to the patient. In the following section, we shall elaborate on various influencing factors and on ways to optimize a patient’s tolerance to anemia.
5.1.2 Individual Tolerance to Anemia
▶ Oxygen demand and oxygen supply. All organs of the human body have their own specific oxygen demand. However, the oxygen demand does not only vary from one organ to another, but it can also vary greatly for the same organ depending on the given situation and its related metabolic effects. If one adds the oxygen demand of all organs, one obtains the body’s total oxygen demand. The oxygen supply, on the other hand, can be calculated as the product of arterial oxygen content and cardiac output. Under resting conditions, the oxygen supply outstrips the oxygen demand by a factor of 4–5. Among other things, this “luxury” supply can balance out transient peaks in the oxygen demand of individual organs. The key determinant of adequate oxygen delivery to the body tissues (DO2) is the regional oxygen supply, which is in turn determined by the oxygen content of the arterial blood and the regional blood flow in the respective organ. The latter differs greatly depending on the underlying disease, which might mean that individual organs are more affected by a limited oxygen supply than others. In the worst case, tissue hypoxia can develop in some organs despite an adequate oxygen supply in the body overall.
Note
Even if the oxygen supply is theoretically sufficient to assure adequate tissue oxygenation, individual organs might be affected by tissue hypoxia.
▶ Compensatory mechanisms in anemia. If the blood volume lost in an acute bleeding situation is replaced with crystalloids or colloids, this results in hemodilution, i.e., the dilution of all blood components, leading to anemia. The subsequent drop in the arterial oxygen content is compensated by a rise in cardiac output and enhanced arteriovenous oxygen extraction. This means that young, healthy patients can tolerate dilutional anemia even if it is extreme. Only at very low hemoglobin concentrations can the loss of circulating oxygen carriers no longer be compensated by a rise in cardiac output. In such a situation, the safety margin of tissue oxygenation is completely exhausted, with the ensuing onset of tissue hypoxia. It is impossible to predict which organs will be affected by tissue hypoxia, as this depends on the perfusion and the resulting oxygen demand of each organ during anemia.
These compensatory mechanisms form the basis for optimization of the perioperative anemia tolerance and thus for the measures of the third pillar of PBM, which should be employed in everyday clinical practice (Goodnough and Shander 2012):
Preoperative preparation of the patient.
Intraoperative optimization of oxygen transport and tissue oxygenation, and application of evidence-based transfusion thresholds.
Postoperative balancing of DO2 and oxygen consumption (VO2).
5.1.3 Preoperative Quantification of the Anemia Tolerance
The body’s ability to compensate for acute anemia can vary greatly from one patient to another. Surprisingly, to date there is no routine clinical test to predict the level of acute dilutional anemia that would critically restrict the DO2 to individual organs, or the entire body, in a given patient. This is because parameters such as regional perfusion and VO2 vary widely between patients in different clinical situations. Therefore, it is currently not possible prior to an operation to determine the hemoglobin concentration that can be deemed “safe” for a patient in terms of compensation for acute anemia. However, this does not mean that it is generally impossible to estimate the potential risk through taking a detailed preoperative medical history and evaluating the patient.
▶ Evaluation of the cardiac reserve. Since the compensatory rise in cardiac output during acute anemia is mainly compensated by a rise in stroke volume, the cardiac reserve is a key determinant of the amount of acute anemia that can be compensated. For this reason, it is recommended that the compensatory ability of the patient’s heart is evaluated prior to surgery with anticipated major blood loss (Habler et al 2006). However, it is not known what kinds of preoperative assessment would be best suited to predict and avoid the development of intraoperative tissue hypoxia. Potential options include the use of standardized evaluation forms, preoperative electrocardiography (ECG), and more complex procedures such as exercise ECG. However, to date there are no clinical studies to demonstrate the superiority of these investigations to a standardized medical history in this context. It is therefore no surprise that pre-operative tests aimed at determining the anemia tolerance of individual patients are only rarely performed in routine clinical practice (van Gelder et al 2012).
▶ Other organ systems. If the evaluation is confined to the cardiovascular system alone, there is a risk of overly focusing on a single organ system without taking other organs into account whose tissue oxygenation is equally at risk. Growing evidence indicates that there are major differences in the ability of various organs to compensate for acute anemia (Torres et al 2005). For example, a hemoglobin concentration of less than 7 g/dL in cardiopulmonary bypass patients led to a marked deterioration in the postoperative renal function, whereas other organs, such as the brain, were not affected by the restricted oxygen supply (Habib et al 2003, Habib et al 2005, Karkouti et al 2005a). Therefore, the preoperative evaluation should include all organ systems that are susceptible to anemia-induced injury, so as to determine the hazard potential for each individual organ.
Note
In routine clinical practice, there are many obstacles to estimating a patient’s ability to compensate for anemia. This makes it much more difficult to set physiological transfusion thresholds.
▶ Transfusion threshold based on hemoglobin. For the reasons outlined above, a different approach tends to be taken in clinical practice. Typically, the decision to administer intra- and postoperative transfusions is based on a hemoglobin threshold value. This value is often determined on the basis of the preoperative tests that have been carried out, but in the vast majority of cases it is well above what the body can actually compensate for.
The range given in the literature is generally between 6 g/dL and 10 g/dL. Whereas a transfusion will generally be required to prevent long-term tissue hypoxia if the hemoglobin concentration is less than 6 g/dL, there is virtually no patient with a hemoglobin concentration of more than 10 g/dL who must receive a transfusion to improve the tissue oxygenation. With hemoglobin values between 6 g/dL and 10 g/dL, the decision depends on comorbidities, local circumstances, and other needs, but in most patients without cardiac impairment a hemoglobin concentration of 8 g/dL is more than sufficient. This approach has been repeatedly verified in clinical studies, both intraoperatively and in intensive care patients (Marik and Corwin 2008, Hajjar et al 2010, Carson et al 2011, Vuille-Lessard et al 2012). Based on these data, it can be postulated that an intraoperative hemoglobin concentration of over 8 g/dL is not needed in the majority of patients with good cardiac health.
The transfusion of RBC concentrates once this generally accepted transfusion threshold has been reached guarantees a safety margin to ensure the maintenance of tissue oxygenation in all organs; however, it has the drawback that many patients receive an RBC transfusion—with all its associated risks—before this is actually warranted by their tissue oxygenation status.
Even though the literature agrees on a transfusion threshold of 8 g/dL for restrictive transfusion regimens, impressive data from the SOAP (Sepsis Occurrence in Acutely Ill Patients) and ABC (Australian Breakthrough Cancer) studies demonstrate that the average hemoglobin concentration in asymptotic patients approaches a value of 10 g/dL, both from previously lower and previously higher values, after a 14-day stay on the intensive care unit (ICU) (Vincent et al 2002, Sakr et al 2010). This phenomenon, which was observed in both studies, suggests that in routine clinical practice a hemoglobin concentration of 10 g/dL has been knowingly or unknowingly adopted as the “transfusion threshold” for all patients, regardless of the published evidence. However, it is beyond doubt that there is no justification to take measures aimed at achieving a hemoglobin concentration of 10 g/dL in ICU patients regardless of their clinical situation and concomitant diseases.
Note
The typical hemoglobin-based transfusion thresholds are 6 g/dL for patients with good cardiac health, and 8 g/dL for those with cardiac impairments. Only in individual cases can a hemoglobin concentration of 10 g/dL be justified.
5.1.4 Preoperative Estimation of the Anticipated Blood Loss
Preoperatively, it is easier to estimate the anticipated blood loss than to predict a patient’s tolerance to anemia. Estimation of the anticipated blood loss does not only help to identify how many RBC units the blood bank should issue preoperatively, but it also helps to determine the scope of the measures that need to be in place to reduce the need for perioperative allogeneic transfusions (see also Chapter 2.3).
5.1.5 Intraoperative Optimization of the Oxygen Demand to Oxygen Supply Ratio
Within the framework of the third pillar of modern PBM, optimization of the ratio of oxygen demand to oxygen supply represents the most important measure to optimize a patient’s tolerance to anemia. By reducing the intraoperative oxygen demand and increasing the oxygen supply, it will be possible for the patient to tolerate lower hemoglobin values than would otherwise be the case.
Intraoperative Reduction of the Oxygen Demand
Therapeutic Hypothermia
There are various methods to achieve an intraoperative reduction of the body’s oxygen demand. One of the best known is, no doubt, lowering of the core temperature, thus inducing therapeutic hypothermia (Azmoon et al 2011). Therapeutic hypothermia also reduces the oxygen demand of individual organs by around 7 % per degree Celsius, thus increasing the tolerance to hypoxia (Polderman 2009). For example, hypothermia significantly improves the likelihood of survival during cardiopulmonary resuscitation (Bernard et al 2002).
However, data from an animal model did not demonstrate unequivocally that therapeutic hypothermia could significantly improve the physiological tolerance to anemia (Perez-de-Sá et al 2002). Besides, this modality has another drawback that militates against its use on a broad scale in clinical practice: therapeutic hypothermia leads to a marked deterioration in the coagulation activity of plasma (Watts et al 1998) and platelets (Kermode et al 1999), and can therefore aggravate intraoperative bleeding. For this reason, all guidelines on the treatment of intraoperative bleeding published to date recommend maintenance of a normal body temperature. Hence, therapeutic hypothermia has a very low priority as a measure to increase the tolerance to anemia in cases of acute bleeding.
Choice of Volume Expander
Depending on the clinical situation, local circumstances, and the treating physician, blood loss is initially replaced using either crystalloids or colloids, or a combination of both. The choice of intravenous fluid to be used for volume expansion is determined by numerous considerations, such as its impact on the microcirculation, kidney function, blood coagulation, and development of interstitial edema. Until recently, it was not known whether the choice of intravenous fluid also had implications for the tolerance to anemia. Whereas the choice did not appear to have any effect in earlier studies with animal models (Van der Linden et al 1993), a study of trauma patients carried out in 2010 revealed that the administration of a hetastarch solution significantly reduced the mortality compared with administration of a purely crystalloid intravenous fluid (Ogilvie et al 2010). This finding was confirmed in an animal study that investigated the effects of lactated Ringer, gelatin, hydroxyethyl starch (HES) 130, and HES 450 solutions on an individual’s tolerance to anemia. The results showed that it is possible to increase the tolerance to anemia through the use of HES 130 compared with all the other solutions, in particular in comparison with lactated Ringer solution (Pape et al 2012b). These more recent findings suggest that modern colloidal solutions are indeed able to increase the perioperative tolerance to anemia. However, since the use of colloids is associated with other problems and no large clinical trials have been conducted in this context, their use cannot be generally recommended for the purpose of increasing the perioperative tolerance to anemia, even if the initial results are promising.
Effects of Anesthesia on the Tolerance to Anemia
The induction of general anesthesia leads to a considerable drop in a patient’sVO2. This phenomenon seems to hold out interesting prospects in terms of increasing the perioperative tolerance to anemia.
However, the link between anesthesia depth and tolerance to anemia has proved to be much more complex in clinical practice. In experiments with dogs, Van der Linden et al demonstrated that the deepening of general anesthesia with halothane did not result in a significant reduction in the so-called critical hemoglobin concentration and therefore in the maximum tolerable amount of acute anemia (Van der Linden et al 2000, Van der Linden et al 2003). Surprisingly, the drop in VO2 induced by this measure was accompanied by a marked decline in the compensatory mechanisms at play in acute anemia. Compared with less deeply anesthetized animals, a reduction in the hemoglobin concentration in the deeply anesthetized dogs did not result in a substantial increase in cardiac output. In the case of halothane, this can be easily explained by the cardiodepressive effects of this inhalational anesthetic. However, Van der Linden et al demonstrated the same effect for Ketanest, which has far weaker cardiodepressive effects (White 1982). Thus, the physiological mechanisms responsible for tolerance to anemia can certainly be influenced by changing the depth of anesthesia; however, the relationship between tolerance to anemia and depth of anesthesia is not linear but probably has an inverted U-shape. In a clinical situation, the optimum anesthesia depth with respect to a patient’s individual tolerance to anemia is difficult to predict. In particular, it is not known whether there are significant differences in the anemia tolerance of sedated versus awake patients.
More recent studies suggest that it is possible to increase the tolerance to anemia through sufficient muscle relaxation. Pape et al (2012a) showed in an animal model that the critical hemoglobin concentration could be significantly reduced by muscle relaxation. Whether these findings can be extrapolated to the clinical situation is currently unclear.
Note
A patient’s tolerance to anemia can be increased by maintaining an optimal intravascular volume during surgery and by adapting anesthesia.
Intraoperative Optimization of the Oxygen Supply
The supply of oxygen to the body tissues (the DO2) is calculated as the product of cardiac output and arterial oxygen content. From a physiological point of view, it seems beneficial to increase the DO2 in acute anemia using several different methods. In theory, this can be achieved either by increasing the cardiac output (optimization of cardiovascular function) or by augmenting the arterial oxygen content.
Optimization of the Cardiovascular Function
In acute anemia, the drop in blood viscosity and the ensuing reduction in endothelial shear forces give rise to an increased release of endothelial nitric oxide, resulting in peripheral arteriolar vasodilatation. This leads to a drop in the mean arterial pressure, regardless of the rise in cardiac output. If the limits of dilutional anemia are reached, this results not only in a critical impairment of all DO2 parameters, but also in a dangerous drop in the coronary perfusion pressure. In animal experiments, the concurrent use of acute normovolemic hemodilution with vasodilator administration did not lead to a disproportionate impairment in cardiac output, but the perfusion pressure of individual organs dropped below the minimum pressure needed (Crystal et al 1991).
▶ Vasopressors and inotropes. One way to counter the phenomenon described would be to administer vasopressors to maintain the organ-specific perfusion pressure. Animal experiments have shown that the tolerance to anemia can be improved through stabilization of the coronary perfusion pressure with noradrenaline (Meier et al 2007). The use of inotropes can also increase the tolerance to anemia (Pawlik et al 1975, Pawlik et al 1976). In both cases, the positive effects of the respective therapeutic approach outweighed the side effects. By contrast, increasing the cardiac output only through raising the heart rate does not appear to improve the tolerance to anemia (Kertscho et al 2012). However, it must be pointed out that almost all data published to date on this topic are based on animal models. Whether the various effects also occur in humans to the same extent, and whether it is indeed possible in the clinic to increase the tolerance to anemia by combining the measures described, has not yet been confirmed by evidence-based research.
Increasing the Arterial Oxygen Content
The oxygen content of arterial blood is determined by the oxygen bound to hemoglobin and the oxygen physically dissolved in plasma. Since there is a decline in the oxygen bound to hemoglobin in acute anemia, the arterial oxygen content can be expanded only by increasing the amount of oxygen physically dissolved in plasma. However, for many years now physiology textbooks have unanimously upheld the view that the amount of oxygen that is physically dissolved in plasma is very small compared with that bound to hemoglobin, and is thus negligible. However, this view fails to properly explain the physiological processes at play in acute anemia.
▶ Increasing the inspired oxygen concentration. An increase in the concentration of inspired oxygen translates into a direct proportionate increase in the partial pressure of oxygen (PO2) in arterial blood. With an inspired oxygen concentration of 100 %, the PO2 of arterial blood can assume values of more than 500 mmHg. Because of the large difference to the PO2 in tissues (< 20 mmHg), the oxygen that is physically dissolved in plasma is ideally suited to be utilized for tissue oxygenation. As the amount of oxygen bound to hemoglobin continues to decline in the presence of anemia, the dissolved plasma oxygen plays an increasing role in tissue oxygenation through hyperoxia. Thus, the dissolved oxygen is an important contributor to tissue oxygenation.
In animal experiments, the use of hyperoxic ventilation once the critical hemoglobin concentration had been reached resulted in improved survival rates (Meier et al 2004), an increased tolerance to anemia (Kemming et al 2003), and maintenance of tissue oxygenation even in the presence of extremely low hemoglobin concentrations (Habler et al 1998, Kleen et al 1998, Kemming et al 2003).
It is therefore no surprise that in most case reports of patients with an extremely low hemoglobin value and no possibility of acute RBC transfusion, the shortage of circulating oxygen carriers was addressed through ventilation with pure oxygen (Zollinger et al 1997, Dai et al 2010). The combination of data from animal experiments and patient case reports justifies the recommendation to administer oxygen in patients with an extremely low hemoglobin concentration to assure tissue oxygenation. However, to date there are no large clinical studies to prove that the use of hyperoxia in acute anemia does indeed improve tissue oxygenation in the clinic. It is also not known whether ventilation with high oxygen concentrations has a positive impact on long-term survival in patients with moderate anemia. In the worst-case scenario, it is conceivable that the side effects of hyperoxia (e.g., hyperoxic arteriolar vasoconstriction, formation of radicals, neurologic toxicity, atelectasis) outweigh the positive effects on oxygen transport and tissue oxygenation.
Note
A patient’s tolerance to anemia can be improved through intraoperative optimization of the cardiovascular function with inotropes and vasopressors and by raising the arterial oxygen content.
5.1.6 Postoperative Optimization of the Oxygen Demand to Oxygen Supply Ratio
In general, the therapeutic principles described above also apply to the postoperative phase, even though the opportunities to influence oxygen supply and demand are more limited than during the intraoperative phase because of less extensive monitoring.
Besides, it was a longstanding belief that an increase in the postoperative hemoglobin concentration, with its accompanying increase in oxygen supply, has a positive impact on the patient’s functional recovery. Therefore, the transfusion thresholds recommended for the postoperative phase were generally higher than those recommended for the intraoperative phase. Only recently has it become clear that this is not the case. Two independent groups demonstrated in patients undergoing orthopaedic surgery that a restrictive transfusion regimen is just as safe as a liberal regimen in terms of postoperative functional recovery, regardless of the patients’ cardiac health (Carson et al 2011, Vuille-Lessard et al 2012).
▶ Infections with a septic course. During the postoperative phase, special attention should be paid to the development of infections with sepsis. For physiological reasons, it can be assumed that the onset of sepsis is accompanied by an increased oxygen demand (Regueira et al 2012) and severely impaired oxygen utilization, resulting in a reduced perioperative tolerance to anemia. It could be shown that the critical DO2 in septic animals was significantly higher than that in healthy animals (Morita et al 2003); however, the critical hemoglobin concentration did not differ significantly between septic and nonseptic animals (Lauscher et al 2011). Even though sepsis is associated with generalized impairments in the microcirculation, oxygen extraction, and oxygen utilization, the septic body retains some tolerance to anemia. In intensive care patients with sepsis, the 30-day mortality was independent of whether a restrictive or a liberal transfusion strategy was applied (McIntyre et al 2004).
Note
Because of the limited possibilities for monitoring, it is much more difficult to manage the oxygen supply and demand during the postoperative phase than during the intraoperative phase.
Conclusion
The third pillar of modern PBM (optimization of the anemia tolerance) is a very effective instrument in the hands of anesthesiologists to influence the perioperative consumption of allogeneic blood. Of paramount importance in this regard are the preoperative preparation of the patient, the intraoperative optimization of oxygen transport and tissue oxygenation, the application of evidence-based transfusion thresholds, and the postoperative balancing of DO2 and VO2. To achieve the maximum benefit, it is important to use a combination of these strategies in line with the needs of the patient rather than using a single measure.
5.2 Determinants of the Decision to Transfuse Red Blood Cells
5.2.1 General Considerations
Allogeneic RBC transfusion, which enables a myriad of surgical procedures involving major blood loss, is considered to be one of the most important medical achievements of the 20th century. Meticulous care must be taken with such blood products because of the transmission risk for certain diseases and the raised perioperative morbidity and mortality of transfused patients. Notwithstanding these risks, blood conserves also save lives, and some patients with postpartum bleeding or severe trauma are unlikely to survive without the transfusion of allogeneic blood. It is only thanks to the supply of allogeneic blood that certain complex operative procedures are possible, and blood products prolong the lives of patients with a hematopoietic disorder caused by, e.g., bone marrow disease. The costs incurred for the transfusion of blood products account for approximately 1.5 % of the budget of an average-sized hospital (Shander et al 2010). Whereas the infection risk posed by transfusion used to be the prime consideration, today there is a growing awareness of other complications of blood products unrelated to infections. These are particularly important for patients who are ill or seriously ill. Therefore, the use of blood products must be targeted and based on strict indication criteria.
Note
The general framework for transfusion is set out in the guidelines from various medical societies and associations (Napolitano et al 2009, BAEK 2011a, Ferraris et al 2011a, Carson et al 2012b, American Society of Anesthesiologists Task Force on Perioperative Blood Management 2015).
▶ Demand for blood products. In the past, homologous blood products were mainly used in perioperative medicine; however, in recent years this trend has changed with RBC concentrates now being increasingly transfused for nonsurgical indications such as gastrointestinal bleeding and impaired erythropoiesis. This change is driven by novel surgical techniques and, of course, by the availability of perioperative blood conservation methods. Since the worldwide implementation of blood conservation strategies, the overall incidence of RBC transfusion in medical and surgical patients has decreased, along with a decrease in the pretransfusion hemoglobin level (Roubinian et al 2014b). At the same time, in line with the trend toward aging societies in high-income countries, there is a rise in the number of hematologic and oncologic diseases requiring transfusion. A prospective study of the indications for RBC transfusion published in 2006 revealed that only 33 % of the transfusions were administered in the peri-operative setting, and only 5 % were for gynecologic/obstetric procedures. Nonsurgical indications were predominantly related to blood diseases (18.2 %), gastrointestinal bleeding (13.8 %), and nonhematologic tumors (8.8 %) (Wallis et al 2006). Transfused patients were on average 66 years old; only 25 % of the transfused RBC concentrates were administered to persons younger than 55 years. Patients aged 40 to 50 years received only 20 units of RBC concentrates per year per 1,000 patients, whereas patients aged 80 years and over received 10 times this amount (200 units per year per 1,000 patients).
▶ Indications. The main indications for transfusion are:
Acute anemia (e.g., because of traumatic blood loss).
Chronic intractable anemia (e.g., bone marrow disease).
Treatment-resistant chronic anemia.
▶ Transfusion criteria. RBC concentrates are transfused to prevent overt anemic hypoxia. However, since the clinical symptoms of anemia are nonspecific, a rational indication must be based on the hemoglobin concentration or the hematocrit and on consideration of the following criteria:
Cause, duration, and severity of anemia.
Extent and progression of blood loss.
Individual ability to compensate for reduced oxygen supply.
Comorbidities that may restrict the compensatory ability in acute anemia.
The patient’s current clinical condition.
Presence or absence of physiological transfusion triggers ( Table 5.1 ).
The patient’s intravascular volume status (RBC deficiencies cannot be reliably identified in hypovolemia).
In addition to these criteria, the latest findings from clinical studies on the relationship between anemia, RBC transfusion, and outcome must be taken into account. The etiology of any form of anemia must be investigated and causal treatment must be initiated whenever possible. The timely transfusion of RBC concentrates is vital in the presence of active bleeding, signs of hypoxia, or hemorrhagic shock. In such situations, the decision to administer a transfusion is based on the hemodynamic parameters and the symptoms of anemia with consideration of the incurred and anticipated blood loss.
Note
Causal therapy of anemia should be initiated whenever possible.
5.2.2 Transfusion in Otherwise Healthy Patients
A normovolemic decrease in the hemoglobin concentration to around 5 g/dL (hematocrit 15 %) is generally well tolerated by patients with normal cardiovascular function (Weiskopf et al 1998). If the hemoglobin value is less than 6 g/dL, there is a risk that individual organ systems will not receive the critical minimum supply of oxygen. At these values, even young, otherwise healthy persons can have ECG changes, impaired cognitive function, or a subjective feeling of tiredness. These changes can be reversed by increasing the hemoglobin value to above 7 g/dL or administering additional oxygen (Weiskopf et al 2002). For this reason, the administration of oxygen is viewed as an emergency measure for the treatment of acute anemia. Based on clinical observations, a hemoglobin value of 4.5 to 5 g/dL (hematocrit ~ 15 %) is considered to represent a critical threshold, in particular in the presence of risk factors; if this threshold is exceeded, a transfusion may be needed. However, the patient’s current intravascular volume status must also be taken into account when making a decision about the indication for transfusion. Laboratory values alone do not represent reliable transfusion triggers in patients with intravascular hypovolemia ( Table 5.2 ).
A Cochrane analysis published in 2012 containing data on 6,264 patients demonstrated that the use of a restrictive transfusion threshold (hemoglobin < 7–9 g/dL) reduced the risk of an individual patient receiving an RBC transfusion. The study authors concluded that patients without acute coronary heart disease and no active bleeding should only receive a transfusion if the hemoglobin value is below 7 to 8 g/dL. The benefits of avoiding transfusion are all the greater against a background of doubtful blood product safety (Carson et al 2012a).
Note
A transfusion trigger should serve as a discussion point and not as a strict indication to transfuse (Koch 2014).

Full access? Get Clinical Tree
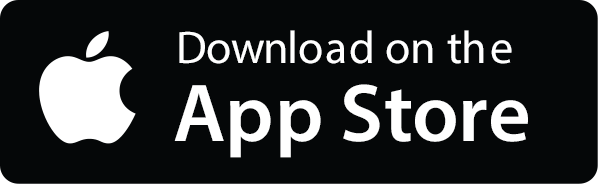
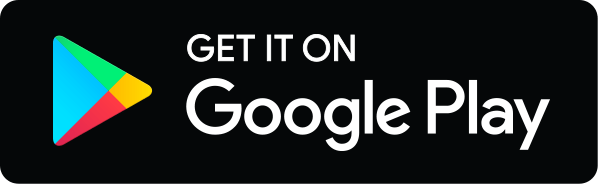
