Abstract
In 2003, the completion of the Human Genome Project resulted in the sequencing of every human gene and subsequently heralded the ‘age of the genome’. Whilst the knowledge of genetics has revolutionised medicine, the phenotypic significance of most genes remains poorly understood. This will be a major focus of physiological research in the future.
In 2003, the completion of the Human Genome Project resulted in the sequencing of every human gene and subsequently heralded the ‘age of the genome’. Whilst the knowledge of genetics has revolutionised medicine, the phenotypic significance of most genes remains poorly understood. This will be a major focus of physiological research in the future.
What is a chromosome?
An individual’s genetic code is packed into the nucleus of each cell, contained in a condensed structure called chromatin. When the cell is preparing to divide, chromatin organises itself into thread-like structures called chromosomes; each chromosome is essentially a single piece of coiled deoxyribonucleic acid (DNA). In total, each cell contains 46 chromosomes (23 pairs), with the exception of the gamete cells (sperm and egg), which contain only 23 chromosomes.
There are two main types of chromosome:
Autosomes, of which there are 22 pairs.
Allosomes (sex chromosomes), of which there is only one pair, XX or XY.
Both types of chromosome carry DNA, but only the allosomes are responsible for determining an individual’s sex.
What is DNA?
DNA is a polymer of four nucleotides in sequence, which is usually bound to a complementary DNA strand and folded into a double helix (Figure 3.1). The DNA strand can be thought of as having two parts:
A sugar–phosphate backbone, made of alternating sugar (deoxyribose) and phosphate groups. The sugars involved in the DNA backbone are pentose carbohydrates, which are produced by the pentose phosphate pathway (PPP; see Chapter 77).
Nucleobases, four different ‘bases’ whose sequence determines the genetic code:
– Guanine (G);
– Adenine (A);
– Thymine (T);
– Cytosine (C).
The double-helical arrangement of DNA has a number of features:
Antiparallel DNA chains. The two strands of DNA run in antiparallel directions.
Matching bases. The two strands of DNA interlock rather like a jigsaw: a piece with a tab cannot fit alongside another piece with a tab – nucleotide A does will not fit alongside another nucleotide A. The matching pairs (called complementary base pairs) are:
– C matches G;
– A matches T.
Hydrogen bonding. The two strands of DNA are held together by hydrogen bonds (a particularly strong type of van der Waals interaction) between the matching bases.
Figure 3.1 Basic structure of DNA.
What is RNA? How does it differ from DNA?
The amino acid sequence of a protein is encoded by the DNA sequence in the cell nucleus. But when the cell needs to synthesise a protein, the code is anchored in the nucleus, and the protein-manufacturing apparatus (the endoplasmic reticulum (ER) and Golgi apparatus; see Chapter 2) is located within the cytoplasm. RNA overcomes this problem: RNA is produced as a copy of the DNA genetic code in the nucleus and is exported to the cytoplasm, where it is used to synthesise protein.
In some ways, RNA is very similar to DNA. RNA has a backbone of alternating sugar and phosphate groups attached to a sequence of nucleobases. However, RNA differs from DNA in a number of ways:
RNA sugar groups have a hydroxyl group that DNA sugars lack (hence ‘deoxy’-ribonucleic acid).
RNA contains the nucleobase uracil (U) in place of thymine (T).
RNA usually exists as a single strand; there is no antiparallel strand with which to form a double helix.
There are three major types of RNA:
Messenger RNA (mRNA). In the nucleus, mRNA is synthesised as a copy of a specific section of DNA – this process is called transcription. mRNA then leaves the nucleus and travels to the ribosomes of the rough endoplasmic reticulum (ER), the protein-producing factory of the cell.
Transfer RNA (tRNA). In the cytoplasm, the 20 different types of tRNA gather the 20 different amino acids and transfer them to the ribosome, ready for protein synthesis.
Ribosomal RNA (rRNA). Within the ribosome, rRNA aligns tRNA units (with the respective amino acids attached) in their correct positions along the mRNA sequence. The amino acids are joined together and a complete protein is released.

Full access? Get Clinical Tree
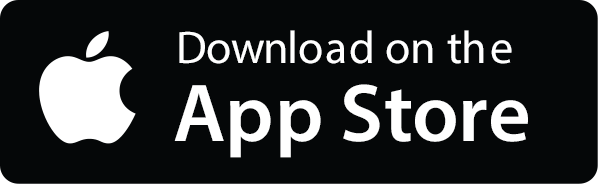
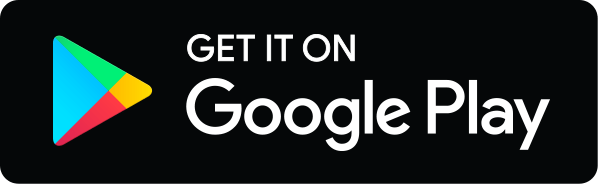