Summary
The anesthesia workstation, commonly referred to as the “anesthesia machine,” is a complex and very specialized piece of equipment that is relatively unique in medical practice. It is, in essence, a device to control the delivery of medical gases to patients, including oxygen, air, nitrous oxide, and volatile anesthetics, along with a specialized ventilator adapted to operating room conditions. The safe use of the anesthesia workstation requires proper training, preuse checkout, and continuous monitoring of its function. The medical literature is replete with examples of patient harm from inappropriate use of the anesthesia workstation and from mechanical or electrical failure of its components. Additionally, volatile anesthetics, while valuable in medical practice, have a very low therapeutic index and manifest severe, and even fatal, side effects when administered improperly. Finally, many patients under general anesthesia are paralyzed for surgery and ventilated through an endotracheal tube. Their safety is completely dependent on the anesthesia professional’s use of the anesthesia workstation to deliver breathing gases, remove carbon dioxide from exhaled gas, and precise administration of volatile anesthetics.
Introduction
The anesthesia workstation, commonly referred to as the “anesthesia machine,” is a complex and very specialized piece of equipment that is relatively unique in medical practice. It is, in essence, a device to control the delivery of medical gases to patients, including oxygen, air, nitrous oxide, and volatile anesthetics, along with a specialized ventilator adapted to operating room conditions. The safe use of the anesthesia workstation requires proper training, preuse checkout, and continuous monitoring of its function. The medical literature is replete with examples of patient harm from inappropriate use of the anesthesia workstation and from mechanical or electrical failure of its components. Additionally, volatile anesthetics, while valuable in medical practice, have a very low therapeutic index and manifest severe, and even fatal, side effects when administered improperly. Finally, many patients under general anesthesia are paralyzed for surgery and ventilated through an endotracheal tube. Their safety is completely dependent on the anesthesia professional’s use of the anesthesia workstation to deliver breathing gases, remove carbon dioxide from exhaled gas, and precise administration of volatile anesthetics. Obviously, mechanical or operator error can be catastrophic and the design and functionality of the contemporary anesthesia workstation represent an evolution of numerous safety features and engineering to decrease the incidence of mechanical and human error.
Overview of Gas Flow from Pipeline and Cylinder Supply Gas Flow through the Anesthesia Workstation
The anesthesia workstation receives, processes, and administers medical gases and volatile anesthetics. The delivery of volatile anesthetics to the patient breathing circuit, which connects to the patient’s airway, requires medical gases such as oxygen, nitrous oxide, and air. The anesthesia workstation receives the gas source from facility pipelines (e.g., intermediate pressure system) in the walls that eventually link to the operating room and from cylinder inlets (e.g., high-pressure system) that attach to the anesthesia workstation [Reference Davey, Diba and Ward1, Reference Sinclair, Thadsad and Barker2]. The wall gas conduits in an operating room are color-coded and fitted via a specific tube diameter to the workstation by gas type. Yellow represents air; green signifies oxygen, and blue denotes nitrous oxide. The diameter–index safety system (DISS) provides gas-specific outlet fastening and helps prevent accidental gas mixing and attachment. The required pipeline gas pressure supplied to the workstation is around 50 psi [Reference Dorsch and Dorsch3–Reference Gurudatt5].
Cylinders offer gas supply if the central source fails or is unavailable. Gas flows through a fail-safe device called a hanger–yoke assembly, which fastens to workstations and ensures unidirectional flow. The apparatus incorporates gas-specific pins and holes (e.g., pin index safety system (PISS)), and gas travels past a filter to prevent debris from accumulating in the cylinder and machine [Reference Dorsch and Dorsch3, Reference Butterworth, Mackey, Wasnick, Butterworth, Mackey and Wasnick6]. Gas cylinders also carry the same color-coded features to help prevent inappropriate assembly and gas mixing.
The high-pressure cylinder gas then flows through an initial pressure regulator, consisting of a diaphragm and a spring, to form a reduced, constant pressure of 45–60 psi. For nitric oxide, gas flows through a nitric oxide pressure regulator and an additional valve, called the fail-safe valve, that closes nitric oxide flow when oxygen from the pipeline or cylinder pressures reach low levels (<20 psi) [Reference Ehrenwerth, Eisenkraft and Berry4]. This helps to prevent a hypoxic gas mixture, in the event of failure or depletion of oxygen supply. For the intermediate-pressure system, the gas source facility pipelines enter the gas flow circuit immediately after bypassing the initial pressure regulator, as the gas pressure is already reduced at approximately 50 psi [Reference Cowles, Butterworth, Mackey and Wasnick7].
After nitric oxide and oxygen pass through second-stage pressure regulators, both gases reach their respective flow control valves (e.g., needle valve), which control the rate of gas flow to the breathing circuit. Color-coded, specifically sized, and textured flow control knobs turn counterclockwise or clockwise to increase or decrease the flow rate, respectively. Gas enters flowmeter glass tubes where measurement of pressure occurs based on the principle that, among other factors, flow through a resistance (e.g., glass tube diameter) corresponds to pressure [Reference Dorsch and Dorsch3]. The bobbin (e.g., float or ball) within the flowmeter glass tube rises in height with increasing flow and lowers in height with decreasing flow. Increasingly, medical gases in newer systems are electronically metered into the breathing circuit and animated flowmeter tubes/displays are replacing glass tubes, with the control dials mimicking the dials on older, mechanical systems.
Following flow and pressure measurements, gases mix in a chamber called the manifold at the top of the flowmeter, according to specific sequences in gas flowmeters. Gas flow exits the flowmeter in a sequence that requires oxygen flowing out first [Reference Butterworth, Mackey, Wasnick, Butterworth, Mackey and Wasnick6]. Arranging oxygen downstream reduces the risk of hypoxic mixtures should a leak emerge from an upstream gas flowmeter tube. Again, this is true only in mechanical, nonelectronic systems. Following gas mixing, gas travels to the common gas outlet and breathing circuit [Reference Caplan, Vistica, Posner and Cheney8, Reference Brockwell and Andrews9]. Table 3.1 describes a summary of the pipeline supply gas flow.
Table 3.1 Pipeline supply gas flow
Steps | Nitrous oxide pipeline | Oxygen pipeline |
---|---|---|
1 | “Fail-safe” valve | |
2 | Nitrous oxide/oxygen pressure regulator | |
3 | Flow control valve | |
4 | Flowmeter glass tube | |
5 | Manifold | |
6 | Common gas outlet | |
7 | Breathing circuit |
Overview of Air and Oxygen Flush Flow
Air may also flow through the anesthesia workstation. Air can travel through the low-pressure circuit (e.g., components after the flowmeters) of the anesthesia workstation, and it also encounters a secondary pressure regulator. Air may also flow to the flowmeter assembly for measuring and ultimately delivery to the breathing circuit and patient, even without sufficient oxygen pressure present.
A direct link from the first oxygen pressure regulator to the common gas outlet is an additional oxygen circuit within the anesthesia workstation that a provider may use with the oxygen flush button (e.g., valve). By circumventing the flowmeter, manifold, and vaporizer, the circuit flows through an oxygen flush regulator to deliver 100% oxygen and supply an oxygen pressure starting from 50 psi [Reference Patil, Shetmahajan and Divatia10, Reference Thompson and Wilkinson11]. Functions of the oxygen flush button include diluting anesthetic gases or mixtures in the breathing circuit with oxygen, inflating the patient breathing bag, and supporting situations in which the patient requires high-flow oxygen at 35–75 L min−1, such as with mask ventilation [Reference Ehrenwerth, Eisenkraft and Berry4, Reference Brockwell and Andrews9]. In newer, fully electronic, and computerized gas delivery systems, there is a mechanical backup/override that can provide oxygen to the breathing circuit in the event of an electrical or software failure.
Overview of Volatile Anesthetic Gas Flow
Volatile anesthetics enter the anesthetic workstation gas flow in the low-pressure system, which consists of the components after the flowmeters (e.g., vaporizers) and lies slightly above atmospheric pressure. Injection and mixing of volatile anesthetics with the combined gas flow occur immediately after the flowmeters. Since volatile anesthetics exist as liquids in room temperature and atmospheric pressure (with the exception of desflurane), vaporization, the transforming of liquid to vapor, must occur before delivery to the common gas outlet and breathing circuit [Reference Brockwell and Andrews9, Reference Hendrickx and De Wolf12]. Since desflurane’s vapor pressure is close to atmospheric pressure, it must be delivered through a specialized “vaporizer” that heats and pressurizes its vapor, which is then electronically metered into the fresh gas flow. Modifications of the flowmeters and vaporizers influence respective gas compositions before gas flows through the anesthetic workstation’s common gas outlet, which attaches to the anesthetic breathing system (e.g., circuit) [Reference Butterworth, Mackey, Wasnick, Butterworth, Mackey and Wasnick6].
Overview of Anesthetic Breathing System Gas Flow
The anesthetic breathing system serves to distribute gases, including oxygen and anesthetic gases, and remove carbon dioxide from the patient. Three commonly used anesthetic breathing systems include the Mapleson F (Jackson-Rees) system, Bain circuit, and circle system. Based on a number of factors, classifications of anesthetic breathing systems include open, semi-open, semi-closed, and closed [Reference Butterworth, Mackey, Wasnick, Butterworth, Mackey and Wasnick13].
The most commonly utilized anesthetic breathing system is the circle system, which employs an inspiratory and expiratory limb made up of tubular arrangements to create a circular gas flow. Gas flows from the common gas outlet to the inspiratory limb and travels through a series of tubular extensions that include corrugated tubes, tracheal tube connectors, and endotracheal tubes to reach the patient’s lung. On exhalation, gases, including carbon dioxide generated by cellular respiration, flow through the expiratory limb to reach a carbon dioxide processor [Reference Dorsch and Dorsch3, Reference Hendrickx and De Wolf12, Reference Butterworth, Mackey, Wasnick, Butterworth, Mackey and Wasnick13]. Gas flows through a Y-piece, which connects the inspiratory and expiratory limbs to the tracheal tube connector, on inhalation and exhalation to complete the circular gas flow. The design also includes check valves to create unidirectional gas flow through the inspiratory and expiratory limbs. Some systems apply rebreathing of exhaled gases, which function to reduce overall anesthetic use and maximize humidification of inhaled gases [Reference Hendrickx and De Wolf12].
Workstation Components
Gas Inlets and Safety Systems
Anesthesia machines typically have multiple gas inlets, with pressure gauges for each inlet [Reference Butterworth, Mackey, Wasnick, Butterworth, Mackey and Wasnick14]. Pipeline inlets are those that supply gas from pipelines that run throughout the healthcare facility [Reference Butterworth, Mackey, Wasnick, Butterworth, Mackey and Wasnick14]. These inlets usually provide oxygen and nitrous oxide from the facility’s central supply [Reference Butterworth, Mackey, Wasnick, Butterworth, Mackey and Wasnick14]. The pipeline inlets utilize tubing that connects to the anesthesia machine via a noninterchangeable DISS fitting that mitigates the risk of incorrect hose attachment [Reference Butterworth, Mackey, Wasnick, Butterworth, Mackey and Wasnick14]. A second gas inlet provides gas from a secondary source stored as either compressed gas (air, oxygen, helium) or liquefied under pressure (nitrous oxide, carbon dioxide) in the cylinders [Reference Butterworth, Mackey, Wasnick, Butterworth, Mackey and Wasnick14]. This gas supply is typically used as a backup supply if the primary pipeline source were to fail [Reference Das, Chattopadhyay and Bose15]. A hanger–yoke assembly attaches these gas cylinders to the anesthesia machine by utilizing a PISS [Reference Das, Chattopadhyay and Bose15]. This safety system is crucial in ensuring correct connection of gases when needed. The yoke assembly safety system consists of index pins, a washer, a gas filter, and a check valve [Reference Das, Chattopadhyay and Bose15]. The check valve prevents retrograde gas flow through the gas inlet back into the attached cylinder [Reference Das, Chattopadhyay and Bose15]. To ensure additional safety, the cylinders are thoroughly checked and tested by manufacturers regularly to ensure the safety of both patients and healthcare professionals operating the machinery [Reference Butterworth, Mackey, Wasnick, Butterworth, Mackey and Wasnick14]. There is also a consistent color-coded labeling system to safely differentiate between the different gases stored in these cylinders [Reference Butterworth, Mackey, Wasnick, Butterworth, Mackey and Wasnick14].
Gauges and Sensors
The pipeline inlet consistently provides gases to the anesthesia machine at approximately 50 psig [Reference Das, Chattopadhyay and Bose15]. The pressure at which a cylinder source provides gases is more variable but is usually downregulated to approximately 45 psig [Reference Das, Chattopadhyay and Bose15].
Pressure Regulators
While the pipeline gas supply maintains relatively constant pressure, variable gas pressure in cylinders complicates flow control [Reference Das, Chattopadhyay and Bose15]. In order to utilize cylinder gas sources more safely, anesthesia machines feature a pressure regulator that keeps the pressure between 45 and 47 psig [Reference Das, Chattopadhyay and Bose15].
Oxygen Flush Valve
The oxygen flush valve provides additional safety to the anesthesia machine by allowing high-flow, high-pressure oxygen to flow directly from the source into the breathing circuit [Reference Petty16]. In this way, oxygen bypasses the vaporizers and flowmeters [Reference Petty16]. Malfunction of this feature is rare but can cause significant harm to the patient if this occurs, resulting in barotraumas or intraanesthetic awareness [Reference Petty16].
Pressure Sensors, Fail-Safe, and Alarms
When oxygen pipeline pressure drops, a fail-safe device senses this drop and ensures that the set oxygen concentration is maintained despite the loss of oxygen pressure [Reference Mun and No17]. The fail-safe system maintains the oxygen concentration by promptly shutting off the flow of nitrous oxide [Reference Mun and No17]. The drop in pressure of oxygen sets off both audible and visual alarms that draw attention to the issue [Reference Dosch and Tharp18].
Oxygen Ratio Proportioning Systems
Most modern machines use a proportioning safety device, in addition to the “fail-safe” pressure threshold shutoff valve [19]. Depending on the manufacturer of the anesthesia machine, these devices either mechanically link the oxygen and nitrous oxide flow controls or reduce the flow of nitrous oxide and other gases via pressure feedback from the oxygen supply, so that the proportion of nitrous oxide to oxygen results in a minimum oxygen concentration at the common gas outlet of between 23% and 25% [19].
Fresh Gas Controllers and Flowmeters
In a circle system, fresh gas flow is typically set to minute ventilation [Reference Patil, Shetmahajan and Divatia10]. If fresh gas flow exceeds the patient’s alveolar minute ventilation, then the fresh gas will force out the remaining exhaled alveolar gas in the breathing circuit before the next inspiration [Reference Patil, Shetmahajan and Divatia10]. If the exhaled carbon dioxide volume is equal to or greater than the patient’s tidal volume, then the next inspiration will only contain fresh gas [Reference Patil, Shetmahajan and Divatia10]. Fresh gas decoupling is an element in newer workstation models that prevents high volumes and pressures from being transported to the patient during inspiration, resulting in a more precise delivery of tidal volume [Reference Butterworth, Mackey, Wasnick, Butterworth, Mackey and Wasnick20]. Flowmeters measure each individual gas before they mix together with other gases and enter into the vaporizer [Reference Butterworth, Mackey, Wasnick, Butterworth, Mackey and Wasnick20]. As noted previously, the oxygen flowmeter is positioned the furthest downstream, which helps maintain flow to the patient in the event of an upstream leak [Reference Butterworth, Mackey, Wasnick, Butterworth, Mackey and Wasnick20]. Electronic flow meters are less likely to develop leaks and breakages since there are fewer mechanical parts [Reference Patil, Shetmahajan and Divatia10]. The electronic numerical display allows for inspection of gas flow even in a dimmed operating room, and data will be electronically sent to an information system, making it an advantageous choice for many surgeries and procedures [Reference Patil, Shetmahajan and Divatia10].
Workstation Ventilators: Bellows versus Piston
Traditionally, anesthesia ventilator bellows are driven either pneumatically by oxygen or by pressurized air [Reference Patil, Shetmahajan and Divatia10]. Some newer ventilators use electronically driven pistons to generate gas flow and do not require oxygen or pressurized gas consumption, an economical feature [Reference Patil, Shetmahajan and Divatia10]. Ventilator bellows in nonpiston machines can be ascending or descending, based on whether the bellow ascends or descends during the inspiratory phase [Reference Patil, Shetmahajan and Divatia10]. A descending bellow is generally safer since it will not fill during a circuit leak [Reference Patil, Shetmahajan and Divatia10]. An advantage of a piston ventilator is the ability to deliver accurate tidal volumes to the patient, regardless of patient size or poor lung compliance [Reference Patil, Shetmahajan and Divatia10].
Vaporizers and Manifold
Vaporizers are located between the flowmeters and the common gas outlet [Reference Butterworth, Mackey, Wasnick, Butterworth, Mackey and Wasnick14]. They receive gas flow from the flowmeter and add a concentration-calibrated amount of volatile anesthetic agent to the gas [Reference Butterworth, Mackey, Wasnick, Butterworth, Mackey and Wasnick14]. Vaporizers are temperature-corrected for the specific anesthetic agent supplied, allowing for the transport of a constant concentration of agent, irrespective of temperature or flow [Reference Butterworth, Mackey, Wasnick, Butterworth, Mackey and Wasnick14]. This temperature correction is significant because high temperatures cause increased amounts of gas to be elaborated from the volatile, liquid state [Reference Butterworth, Mackey, Wasnick, Butterworth, Mackey and Wasnick14]. Oxygen enters the manifold downstream to the other gases, which prevents hypoxia in case there is a gas leak upstream [Reference Butterworth, Mackey, Wasnick, Butterworth, Mackey and Wasnick14]. Unidirectional valve malfunction has occurred in older anesthesia machines because of condensation from water vapor [Reference Patil, Shetmahajan and Divatia10]. Condensation can be reduced by heating the manifold and using water traps to collect the liquid [Reference Patil, Shetmahajan and Divatia10].
Common Gas Outlet and Check Valve
The common gas outlet is the single connection between the vaporized volatile anesthetic and the patient’s airway [Reference Butterworth, Mackey, Wasnick, Butterworth, Mackey and Wasnick14]. The common gas outlet supplies the tidal volume delivered to the patient during inspiration [Reference Butterworth, Mackey, Wasnick, Butterworth, Mackey and Wasnick14]. Gas composition in the gas outlet is affected by different elements, compared to gas composition in the breathing circuit [Reference Butterworth, Mackey, Wasnick, Butterworth, Mackey and Wasnick14]. In the gas outlet, the composition of the gas is affected by alterations in flowmeters and vaporizers [Reference Butterworth, Mackey, Wasnick, Butterworth, Mackey and Wasnick14]. Additionally, a one-way check valve is positioned between the vaporizers and the oxygen flush [Reference Butterworth, Mackey, Wasnick, Butterworth, Mackey and Wasnick14]. The purpose of the check valve is to prevent retrograde gas flow into the pipeline supplies, which can occur from variations in positive pressure ventilation in older anesthesia machines [Reference Butterworth, Mackey, Wasnick, Butterworth, Mackey and Wasnick14].

Full access? Get Clinical Tree
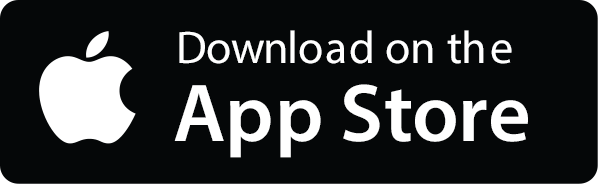
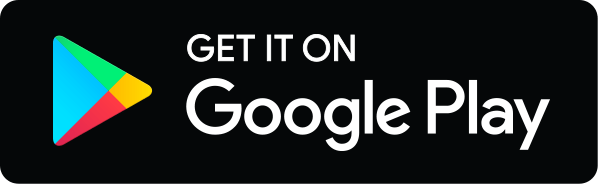
