Abstract
Despite more than six decades of clinical experience and considerable research, the characteristics of ‘optimal’ CPB remain imprecisely defined. This chapter discusses the main areas of controversy (Box 26.1).
Despite more than six decades of clinical experience and considerable research, the characteristics of ‘optimal’ CPB remain imprecisely defined. This chapter discusses the main areas of controversy (Box 26.1).
Box 26.1 Controversies in CPB management
- CPB perfusion characteristics
Optimum perfusion pressure
Pressure versus flow
Pulsatile versus non-pulsatile
- Temperature
Normothermia versus hypothermia
- Acid–base management
pH-stat versus α-stat
- Hb
Minimum haematocrit on CPB
- CPB equipment
Pump characteristics
Circuit surface modification
Reservoir characteristics
Processing cardiotomy blood
- Mini CPB
Reduced RBC transfusion to correct haemodilution versus logistic challenges
- Ventilation and oxygenation
Optimal target for oxygenation
To ventilate or not to ventilate the lungs?
Pressure versus Flow
Prolonged periods of hypotension and hypoperfusion are deleterious, with the brain being the organ most at risk. Despite the fact that routine CPB perfusion pressures of 40–60 mmHg represents deliberate hypotension, the majority of patients appear to survive without evidence of significant injury. When the pressure falls below this range, an increase in either pump flow rate or administration of a vasoconstrictor to increase the SVR is the usual response. The question of whether perfusion pressure or flow rate is more important for organ preservation remains unanswered. The proposed advantages and disadvantages of higher and lower CPB perfusion pressure are summarized in Table 26.1.
Table 26.1 Proposed advantages and disadvantages of low and high CPB perfusion pressure
Perfusion pressure | Advantages | Disadvantages |
---|---|---|
Lower (<60 mmHg) | ↓ Incidence of emboli ↓ Haematological trauma ↓ Collateral warming of the heart Less blood in operative field ↑ Myocardial protection ↓ Cardiotomy suction | Cerebral and renal hypoperfusion |
Higher (>80 mmHg) | Vital organ perfusion maintained In event of cerebral injury secondary to emboli, collateral flow is said to be pressure-dependent | ↑ Haematological trauma ↑ Risk of emboli ↑ Bleeding complications ↑ Suture line stress ↑ Collateral flow to heart ↓ Reduced duration of cardioplegia |
At normocarbia, cerebral autoregulation maintains the cerebral blood flow (CBF) over a range of perfusion pressures (50–150 mmHg). During hypothermic CPB, the lower limit of cerebral autoregulation falls to as low as 30 mmHg. In the absence of significant cerebrovascular disease, cerebral hypoperfusion will be unlikely with a MAP higher than 40 mmHg and this remains the lowest acceptable pressure in adult practice. There is general agreement that a target of perfusion pressure of 50–60 mmHg is safe in the majority of cases.
Cerebral autoregulation is altered by a number of disease processes (e.g. hypertension, DM) and, to a lesser extent, by volatile anaesthetic agents and vasodilators. It has been argued that two groups of patients might benefit from an increased perfusion pressure (i.e. 70–80 mmHg) during CPB – those with altered cerebral autoregulation and those with cerebrovascular disease (Box 26.2).
Box 26.2 Patient groups which may benefit from a higher CPB perfusion pressure
Advanced atherosclerosis
Severe (grade IV and V) atheromatous disease of the descending thoracic aorta (visible on TOE) is a good marker of atheromatous disease near the aortic cannulation site, which is not well visualized with TOE
Chronic hypertension
In patients with chronic, poorly controlled hypertension the pressure–flow autoregulation curve is shifted to the right, therefore a mean perfusion pressure > 50 mmHg is required to maintain the flow
Cerebrovascular disease
Patients with cerebrovascular disease and those with a history of stroke may have impaired regional cerebral autoregulation and are at a greater risk of a neurological injury
DM
These patients appear to have an impaired metabolism–flow coupling during CPB, with possibly some loss of pressure–flow regulation. It has therefore been postulated, but not proven, that a higher perfusion pressure is required during rewarming or during normothermic CPB.
Age > 70 years
Increasing age does not affect cerebral autoregulation per se. However, there may be slower vasodilatation of cerebral resistance vessels during rewarming, leading to transient episodes of metabolism–flow mismatch, with resultant ischaemia. Unless there is coexisting atherosclerotic or hypertensive disease there is, as yet, no evidence that age per se is a reason for using high perfusion pressures.
In 1995, a randomized trial of higher (80–100 mmHg) versus lower (50–60 mmHg) CPB perfusion pressures in 248 CABG patients showed that combined neurological and cardiac outcomes, 6 months after surgery, were significantly better in the higher-pressure group. The study was criticized for analyzing combined outcomes, for using multiple comparisons, for the finding of a greater stroke rate (7.2%) in the lower-pressure (control) group and for being insufficiently powered to detect a 50% reduction in stroke rate. The following year, TOE findings in 75% of these patients were published and it became apparent that the incidence of high-grade aortic atheroma was greater in the lower-pressure group (~30% versus 40%). It can be tentatively concluded that higher perfusion pressures may reduce the risk of stroke in patients with severe aortic atheroma (Box 26.2).
By contrast, a retrospective study of ~3,000 patients undergoing CABG surgery demonstrated a significant association between lower CPB perfusion pressure and increased postoperative stroke or coma. This finding might indicate that patients deemed to be at higher risk were managed using higher perfusion pressures. Alternatively, it might be concluded that a higher perfusion pressure during CPB is not without risk.
Multiple variables, including blood viscosity, flow rate, depth of anaesthesia and vasoactive drug use, affect the MAP. The effect of these variables on clinical outcomes makes the interpretation of studies assessing the MAP difficult and, as such, there is currently insufficient evidence to recommend an optimal MAP for patients undergoing cardiac surgery.
Studies examining the influence of the CPB flow rate on the CBF and metabolism have produced conflicting results – the principal study design flaw being the failure to control for the haematocrit. The only conclusion that can be drawn is that, within the bounds of usual clinical practice, modest changes in flow rate have little impact on the CBF at normocarbia.
It should be borne in mind that:
Tissue oxygen delivery is a function of the pump flow rate, SaO2 and haematocrit
The pump flow rate gives no indication of regional organ perfusion
The only organ amenable to flow modification at a constant pressure is the brain (by changing the PaCO2). Evidence of inadequate tissue perfusion includes a reduced SvO2, metabolic acidosis and hyperlactataemia. Assuming a normal Hb concentration, the recommended pump flow rate at normothermia is 2.2 l min–1 m–2. The reduction in metabolic rate that accompanies hypothermia (50% for every 7 °C) permits flow rates to be reduced.
In a recent study, TCD was used to monitor cerebral autoregulation and to tailor the perfusion pressure during non-pulsatile CPB in 617 patients undergoing cardiac surgery. Interestingly, the lower and upper limits of autoregulation were reported as 65±12 mmHg and 84±11 mmHg, and the ‘optimal’ MAP was 78±11 mmHg.
Normothermia versus Hypothermia
Hypothermic CPB was widely practised until the mid 1990s. Studies suggesting that normothermic cardioplegia and CPB may improve myocardial protection during cardiac surgery led to the adoption of so-called ‘warm-heart’ or normothermic techniques. The principal concern is that an improved myocardial outcome is achieved at the expense of decreasing the margin of safety afforded by hypothermia and increasing the risk of neurological injury.
The Toronto Warm Heart Investigators reported no increase in adverse neurological outcomes in patients maintained at normothermia (33–37 °C versus 25–30 °C) during CPB, whereas the Atlanta Group (Mora et al.) reported a marked increase in neurological injury. Based on patient numbers, however, the evidence accumulated in subsequent studies suggests that the avoidance of hypothermia during CPB does not increase the risk of adverse neurological outcomes (Box 26.3).
Published in 2001, a Cochrane review of 17 studies (Rees et al.) revealed that hypothermia was associated with a trend towards a reduction in non-fatal strokes and a trend towards an increase in non-stroke-related perioperative deaths. In addition, hypothermia was associated with a higher incidence of myocardial damage and low CO syndrome, although the temperature management strategy appeared to have no impact on the incidence of non-fatal MI. The authors concluded that there was no definite advantage of hypothermia over normothermia in the incidence of clinical events.
Box 26.3 Reasons for failure to demonstrate hypothermic neuroprotection
Normothermic CPB in many cases meant a temperature of 35.5 °C, which may have conferred a degree of neuroprotection
Inadvertent cerebral hyperthermia during the rewarming phase of hypothermic CPB
Patients in both hypothermic and normothermic CPB groups were relatively normothermic at times of greatest cerebral vulnerability – aortic cannulation/decannulation and onset/offset of CPB
Pulsatile versus Non-Pulsatile Flow
It has long been thought that pulsatile CPB is physiological and therefore beneficial. The extra kinetic energy applied to blood during pulsatile flow appears to improve RBC transit, capillary perfusion and microvascular flow, and to enhance lymphatic drainage. In patients undergoing prolonged periods of CPB using non-pulsatile flow, increases in catecholamines and activation of the renin–angiotensin system leads to systemic vasoconstriction, reduced organ perfusion and a predisposition to low CO syndrome following separation from CPB. Generation of pulsatile flow can be achieved with programmable roller pumps, ventricular blood pumps, compression plate pumps and with an IABP during non-pulsatile flow. Most modern CPB machines can deliver pulsatile flow without additional equipment or expense (Box 26.4). The contradictory conclusions of clinical studies may in part be explained by differences in the pressure–flow characteristics of pulsatile CPB employed.
Box 26.4 Putative benefits of pulsatile and non-pulsatile CPB
Pulsatile
↑ Myocardial (subendocardial) perfusion, oxygenation and contractility
↑ Renal (cortical) blood flow and urine output
↑ Cerebral perfusion
↓ Catecholamine, renin, angiotensin, aldosterone and lactate levels
Preserved baroreceptor function
Maintenance of pancreatic β-cell function
Non-pulsatile
↓ Haemolysis
↓ Platelet damage
Less complex CPB system
In patients with pre-existing renal insufficiency, pulsatile CPB appears to be associated with improved postoperative renal function. The application of an AXC abolishes any potential benefit to the ischaemic heart during pulsatile CPB. Published in 1995, a Canadian study of 316 CABG patients demonstrated that pulsatile CPB was associated with a lower mortality and cardiovascular morbidity but there was no improvement in neurological and cognitive outcomes (Murkin et al). One reason why subsequent investigations may have failed to replicate these findings is the lack of definition of what constitutes pulsatile flow, how to quantify it and whether or not a certain period of bypass time is required before a difference is seen. In addition, the amount of haemodynamic energy delivered by different pulsatile pumps can vary widely and the pressure waveform can be affected by other components of the bypass system. A recent review called for future investigators to standardize CPB systems and conduct when investigating this question.
Alpha-Stat versus pH-Stat
The solubility of a gas in a liquid is inversely proportional to temperature. As the temperature falls, the total gas content remains unchanged but the partial pressure exerted by the gas will fall as a result of increased solubility. In a hypothermic patient, blood-gas analysis performed at 37 °C will mask a fall in the PaO2 and PaCO2. The PaCO2 is reduced by 4.4% for every 1 °C decrease in temperature, roughly 2 mmHg for each degree less than 37 °C. If temperature-corrected blood-gas analysis is performed, hypothermic patients will appear hypocapnic and alkalotic. Two blood-gas management strategies have emerged to maintain normal physiology, α-stat and pH-stat blood-gas management (Table 26.2).
Table 26.2 The differences between the clinical applications of α-stat and pH-stat blood-gas management strategies
α-stat | pH-stat |
---|---|
Derives its name from the ionization state of enzymatic α-histidine-imidazole groups, which is maintained constant Blood-gas analysis results are not corrected for temperature Target is ‘normal’ blood gases at 37 °C Temperature-corrected hypocarbia and alkalosis tolerated No additional CO2 administered to patient | So-called because pH is maintained at ~7.4 regardless of blood temperature Blood-gas analysis results are corrected for temperature Target is ‘normal’ blood gases at blood temperature Temperature-uncorrected hypercarbia and acidosis tolerated Additional CO2 administered to patient |
During hypothermic CPB, blood vessels maintain their responsiveness to PaCO2 and modulate organ blood flow accordingly. The impact of the blood-gas management strategy on neurological, cardiac and renal outcomes following hypothermic CPB has generated considerable debate (Table 26.3).
Table 26.3 Putative advantages and disadvantages of α-stat and pH-stat blood-gas management strategies
Advantages | Disadvantages | |
---|---|---|
α-stat | Cerebral autoregulation preserved ↓ Cerebral microembolization Improved neurological outcomes ↓ Cerebral injury post DHCA Improved myocardial function | Risk of cerebral hypoperfusion |
pH-stat | More uniform cerebral cooling ↑ H+ reduces organ metabolism HbO2 dissociation curve right shifted | Pressure-passive CBF ↑ Cerebral microembolization ↑ Free-radical-induced tissue damage |

Full access? Get Clinical Tree
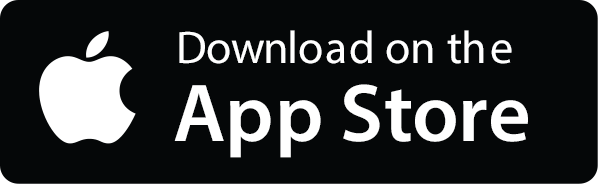
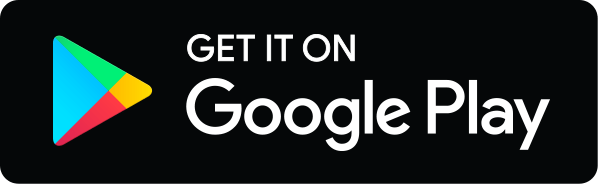
