Abstract
Total body water represents approximately 60% of body weight (45 litres in a 75 kg male). Sixty-six per cent is intracellular water (30 litres), 33% is extracellular (15 litres). The extracellular fluid volume is 25% intravascular (3 litres) and 75% extravascular (interstitial) (12 litres). Total intravascular volume combines intravascular extracellular fluid (3 litres) and cellular components (2 litres).
Body Fluid Compartments
Total body water represents approximately 60% of body weight (45 litres in a 75 kg male). Sixty-six per cent is intracellular water (30 litres), 33% is extracellular (15 litres). The extracellular fluid volume is 25% intravascular (3 litres) and 75% extravascular (interstitial) (12 litres). Total intravascular volume combines intravascular extracellular fluid (3 litres) and cellular components (2 litres).
Intracellular
The composition of the intracellular volume is maintained by a metabolically active cellular membrane. It has a low sodium concentration (10 mmol.l−1) and a high potassium (150 mmol.l−1) concentration.
Interstitial (Extravascular)
The interstitial volume is that part of the extracellular volume that is not present in the plasma. It has an electrolyte composition that is similar to plasma with a high sodium concentration (140 mmol.l−1) and a low potassium concentration (4 mmol.l−1). It has less protein than the plasma and therefore a lower oncotic pressure.
Intravascular
The intravascular compartment has a composition similar to the interstitial space. The plasma has a number of key functions, which include providing the fluid volume necessary to suspend the red cells, clotting and immunological functions.
Movement between Compartments
Traditionally, fluid moves between compartments via osmotic and hydrostatic gradients, as predicted by the Starling equation:
Capillary hydrostatic pressure (Pc) is affected by circulating volume and intravenous fluid administration.
Interstitial hydrostatic pressure (Pi) is affected by interstitial volume.
The reflection coefficient (σ) is altered in states of acute inflammation, trauma, surgery or illness resulting in loss of barrier function and increase in transcapillary flux (Jv). Chronic ill health and poor nutritional status result in hypoalbuminemia and subsequent reduction in capillary oncotic pressure and tissue oedema.
πi represents interstitial oncotic pressure.
Traditionally, net Jv is out of the capillary into the interstitium at the arteriolar end but the direction of Jv reverses at the venous end to be into the capillary. Thus, fluid is lost from the capillary at the arteriolar end and reabsorbed at the venous end. According to Starling, intravenous infusion of artificial colloids increases the capillary oncotic pressure (πc) and promotes fluid reabsorption into the capillaries.
However, an alternative ‘revised’ Starling equation has been suggested, in which no fluid reabsorption takes place within the capillary, even at the venous end (the ‘no absorption rule’). Consequently, Jv out of the capillary occurs all along its length, with fluid not being reabsorbed directly into the capillaries at all, but rather being returned to the circulation via the lymphatics. This is based on the relatively recent discovery of an endothelial glycocalyx layer (glycocalyx), an endoluminal web of membrane bound proteoglycans, glycoproteins and glycosaminoglycans which is present in capillaries, arterioles and venules.
The glycocalyx separates plasma from a ‘protected’ region of the sub-glycocalyx space which is almost protein free. The sub-glycocalyx space contains non-circulating plasma volume and has a colloid oncotic pressure of approximately 70% of the capillary lumen. The sub-glycocalyx colloid oncotic pressure (πsg) replaces the interstitial colloid oncotic pressure πi as a major determinant of transcapillary flow along oncotic gradients. Jv is therefore proportional to πc – πsg. Jv out of the capillary depends entirely on Pc when the πc – πsg difference is maximal (as is usual in health) therefore in a euvolaemic patient infusion of colloid (or crystalloid) increases the Pc and fluid moves out of the capillary despite the potential increase in πc (seen with colloid) .
The volume status of the patient and subsequent Pc is the most important determinant of fluid movement out of the capillaries, more so than the colloid oncotic effect of the infused fluid, in contrast to traditional Starling physiology.
In hypovolaemia or vasodilation, Pc is low. Infusion of crystalloid or colloid does not increase Pc above the oncotic pressure gradient πc – πsg, and as a result, Jv remains close to zero. The important role played by Pc in Jv is especially in evidence where the capillary hydrostatic pressure falls with the induction of anaesthesia.
In inflammatory states there is a reduction in plasma albumin (an important contributor to πc) and trans-capillary escape of albumin to the tissues. Administering albumin does not seem to improve flow back into the tissues due to the no absorption rule. An increase in capillary porosity, such as might occur in severe inflammation, sepsis or trauma, increases the Jv out of the vasculature for any given Pc.
Volume Kinetics
It is possible to apply a two-compartment model to intravenous fluids with two functional, rather than anatomical compartments, V1 and V2 (see Figure 21.1). V1 corresponds to plasma volume and V2 represents the interstitial fluid volume that can be expanded by fluid moving out of the plasma after intravenous infusion. V2 is smaller than the total interstitial volume because not all of the interstitial fluid volume can be expanded (e.g. that contained inside the skull or long bones).
Crystalloids are reported to expand the plasma volume by 20%, but this is an understatement in patients undergoing general anaesthesia who are vasodilated and where the plasma volume expanding effects are greater and longer lasting. This is because there is a dramatic fall in fluid movement from V1 to V2 under general anaesthesia. The pattern is such that at the onset of general or spinal anaesthesia fluid movement falls to such an extent (temporarily) that fluid transfer out of the vascular space (equivalent to Jv) might stop completely depending on the fall in mean arterial pressure.
Intravenous Fluids
Intravenous fluids may broadly be classified into crystalloid and colloid solutions.
Crystalloid Solutions
Solutions of inorganic ions and small organic molecules dissolved in water are referred to as crystalloids. The main solute is either glucose or sodium chloride (or both), and the solutions may be isotonic, hypotonic, or hypertonic with respect to plasma. Table 21.1 lists the contents of common intravenous crystalloid solutions.
Table 21.1 Composition of crystalloids
Na+ mmol.l−1 | Cl− mmol.l−1 | K+ mmol.l−1 | HCO3− precursor mmol.l−1 | Ca2+ mmol.l−1 | Mg2+ mmol.l−1 | Glucose mmol.l−1 | pH | Osmolarity mOsm.l−1 | Osmolality mOsm.kg−1 (tonicity) | |
---|---|---|---|---|---|---|---|---|---|---|
Plasma | 134–145 | 95–105 | 3.5–5.3 | 24–32 | 2.2–2.6 | 0.8–1.2 | 3.5–5.5 | 7.35–7.45 | 275–295 | |
0.9% NaCl | 154 | 154 | 0 | 0 | 0 | 0 | 0 | 4.5–7.0 | 308 | 286 |
0.18% NaCl & 4% glucose | 31 | 31 | 0 | 0 | 0 | 0 | 222 | 4.5 | 284 | 52 |
0.45% NaCl & 4% glucose | 77 | 77 | 0 | 0 | 0 | 0 | 222 | 432 | 134 | |
5% glucose | 0 | 0 | 0 | 0 | 0 | 0 | 278 | 3.5–5.5 | 278 | 0 |
0.9% NaCl in 5% glucose | 154 | 154 | 0 | 0 | 0 | 0 | 278 | 586 | 286 | |
Hartmanns | 131 | 111 | 5 | 29 (lactate) | 2 | 0 | 0 | 5.0–7.0 | 278 | 256 |
Lactated Ringers | 130 | 109 | 4 | 28 (lactate) | 1 | 1 | 0 | 6.0–7.5 | 273 | 256 |
Plasma-lyte | 140 | 98 | 5 | 27 (acetate) 23 (gluconate) | 0 | 1.5 | 0 | 7.4 | 295 | 271 |
8.4% sodium bicarbonate | 1000 | 0 | 0 | 1000 | 0 | 0 | 0 | 2000 |
0.9% Sodium Chloride
First used in the late 1800s, 0.9% saline is a solution of sodium and chloride. The 154 mmol.l−1 sodium and 154 mmol.l−1 of chloride maintains electrochemical neutrality. It is difficult to describe it as ‘normal’ when the chloride content is nearly 50% greater than plasma. There is a plethora of evidence from a variety of patient and volunteer studies that use of 0.9% saline causes unwanted and potentially undesirable physiological effects. Infusion of 0.9% saline in healthy volunteers has been shown to cause the following effects; metabolic acidosis (hyperchloraemic), renal vasoconstriction, reduced renal blood flow, reduced glomerular filtration rate, abdominal pain, tiredness and difficulty thinking.
Balanced Solutions
To address the problems with 0.9% sodium chloride, balanced solutions have been developed that provide an ion content similar to that of plasma by avoiding the excess chloride. One potential option with which to substitute chloride in balanced solutions (to maintain overall electrochemical neutrality) is bicarbonate. However, difficulties with storage and poor solubility of bicarbonate salts has led to the use of bicarbonate precursors. The most common bicarbonate precursors are the organic anions lactate, acetate or gluconate.
Balanced Solutions with Lactate
Ringer’s solution appeared in the 1800s and was probably the first balanced crystalloid that contained sodium chloride, potassium chloride and calcium chloride. The solution as we know it today is Lactated Ringer’s, after sodium lactate was added by Alexis Hartmann in the 1930s. The exact composition and marketing name of Lactated Ringer’s varies slightly between different manufacturers. Commercial solutions available include Hartmann’s solution and compound sodium Lactate.
The advantages of lactate are that it can be metabolised at high rates (up to 100 mmol.hr-1) in patients with normal liver function so that up to 4 litres can be given quickly to a healthy adult patient weighing 70 kg without any lactate accumulation. Metabolism is to carbon dioxide (CO2) or via gluconeogenesis. A potential disadvantage in the peri-operative use of lactate-balanced solutions is the need for a functioning liver and the increased oxygen consumption due to its metabolism. Accumulation of lactate from balanced solutions might confound the use of lactate as a marker of tissue hypoperfusion, but there is no associated acidaemia because these solutions contain lactate not lactic acid.

Full access? Get Clinical Tree
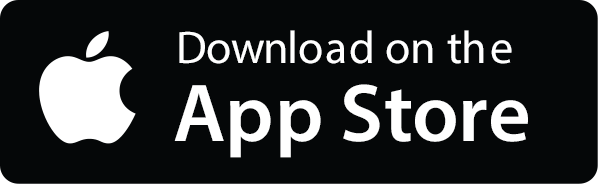
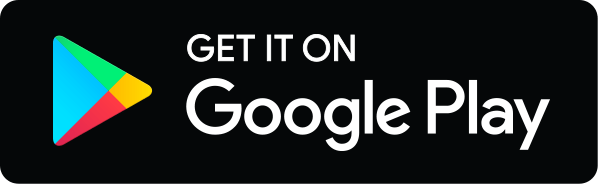
