Summary
Kidneys are paired organs, the primary function of which is to excrete metabolic byproducts involving physiologic processes of filtration, reabsorption, secretion, and excretion. The nephron forms the basic functional unit of kidneys and are primarily involved in urine production, excretion of metabolic waste, maintaining serum osmolality, and acid–base homeostasis. All these physiologic processes involve neurohormonal mechanisms and transporters.
Basic Renal Physiology
Kidneys are paired organs, the primary function of which is to excrete metabolic byproducts involving physiologic processes of filtration, reabsorption, secretion, and excretion. The nephron forms the basic functional unit of kidneys and is primarily involved in urine production, excretion of metabolic waste, maintaining serum osmolality, and acid–base homeostasis. All these physiologic processes involve neurohormonal mechanisms and transporters.
The nephron has its origin in the cortex at the glomeruli and ends at the papilla by joining the collecting ducts. The glomeruli of the nephron contain afferent and efferent capillary tufts where the ultrafiltrate flows into the proximal convoluted tubule (PCT), all of which lie in the cortex. The loop of Henle (LOH), distal convoluted tubule (DCT), and collecting duct lie in the medulla. Nephrons are anatomically classified into cortical and juxtamedullary nephrons. Juxtamedullary nephrons extend into the medulla and play an indispensable role in urine concentration [Reference Preuss1]. Approximately 99% of the filtrate is reabsorbed as it moves down the nephron tubules.
Juxtaglomerular Complex
The juxtaglomerular complex is a small structure within each nephron involved in the regulation of the nephron, and has three different cell types:
1. Macula densa: specialized cells of the DCT that regulate the juxtaglomerular apparatus (JGA) via paracrine regulation of sodium and chloride sensing
2. Juxtaglomerular cells: innervated by sympathetic nerves and secrete renin, based on afferent arteriolar pressure and chloride concentration
3. Extraglomerular mesangial cells: contractile cells that help regulate vascular tone.
Renal Blood Supply
The kidneys represent only 0.5% of body weight but receive approximately 20–25% of cardiac output via the renal arteries. The renal arteries arise from the aorta, dividing into segmental, interlobar, and arcuate arteries. These further give rise to afferent arterioles, forming a network or glomerulus, and then evolve into efferent arterioles. Efferent arterioles form the vasa recta and eventually drain into the renal veins via peritubular capillaries.
Regulation of Renal Blood Flow
Although renal blood flow is determined by arteriovenous pressure difference, it is renal autoregulation that ensures sustainable blood flow with blood pressure fluctuation, involving myogenic and neurohumoral mechanisms [Reference Preuss1]. Autoregulation of renal blood flow normally occurs at mean arterial blood pressures of 80–180 mmHg, via afferent arteriolar tone and myogenic autoregulation.
1. Renin–angiotensin–aldosterone system (RAAS) axis: This is a neurohormonal feedback system that regulates blood pressure and maintains body fluid balance by regulating renal blood flow, which involves renin, angiotensin II, and aldosterone. Any cause leading to renal hypoperfusion is detected by the afferent arteriole, hyponatremia is detected by the macula densa, or increased renal beta-1 receptor sympathetic activation; leads to renin secretion by the juxtaglomerular cell. Renin then converts angiotensinogen produced by the liver to angiotensin I, which is then converted to angiotensin II in the lungs via angiotensin-converting enzyme. Angiotensin II acts as potent vasoconstrictor and maintains the glomerular filtration rate (GFR) via efferent arteriole vasoconstriction, and stimulates thirst by secretion of antidiuretic hormone (ADH) from the posterior pituitary. Angiotensin II also induces aldosterone secretion from the adrenals, which increases sodium and water reabsorption. This aids in restoring blood volume and blood pressure, and stimulates secretion of hydrogen ions and potassium to maintain a homeostatic milieu.
2. Tubuloglomerular feedback: This feedback system communicates between the tubules and the glomeruli, and maintains the GFR over a wide range of fluctuating blood pressures. The macula densa in the JGA responds to sodium concentration in the DCT as an indicator of the GFR. Sodium chloride is sensed by apical Na–K–2Cl cotransporters. Sodium chloride concentration in the tubular fluid leads to increased or decreased GFR via reflex changes in afferent arteriolar vascular tone. Hypotonic urine leads to vasodilation of the afferent arteriole, leading to increased GFR. Hypertonic urine leads to vasoconstriction of the afferent arteriole, leading to decreased GFR.
a. Factors increasing tubuloglomerular feedback: adenosine, thromboxane, aldosterone, angiotensin II, and prostaglandins.
b. Factors decreasing tubuloglomerular feedback: atrial natriuretic peptide, cyclic adenosine monophosphate, nitric oxide, and prostacyclin.
Autonomic Autoregulation
Sympathetic innervation to the kidneys is through the celiac and renal plexuses, which carry T4–L1 outflow. Sympathetics innervate the JGA via beta-1 and renal vessels via alpha-1 receptors. Norepinephrine binds to alpha-1 receptors, leading to vasoconstriction of arterioles and decreased blood flow. Dopamine binds to D1 receptors and causes arteriolar vasodilation, which increases blood flow.
Hormonal Regulation via Natriuretic Peptides
1. Atrial natriuretic peptide (ANP): secreted in response to overextension of the atrium secondary to volume overload.
2. Brain natriuretic peptide (BNP): secreted in response to overextension of the ventricles secondary to volume overload.
Increased volume overload leads to increased sodium and water excretion. These peptides also increase the GFR via dilation of afferent arterioles and inhibit adrenocorticotrophic hormone (ACTH), renin, and aldosterone secretion.
Glomerular Filtration Rate and Creatinine Clearance
Glomerular filtration is the process by which the kidney filters blood primarily based on the hydrostatic and osmotic pressure difference. The GFR is used as a surrogate marker of kidney function and for staging of chronic kidney disease (CKD). Normal GFR varies according to body habitus, sex, and age – males: 120 mL/(min 1.73 m2). A GFR of < 60 mL min−1 denotes the stage when CKD complications start to arise. Creatinine clearance is the rate of clearance of creatinine by the kidneys and is used clinically to estimate the GFR.
Creatinine Clearance (Urine Creatinine × Urine Flow Rate/Serum Creatinine)
Ultrafiltration is a vital step in urine formation in the glomeruli of the nephron at a glomerular filtration rate (GFR) of 125 mL min−1. The basement membrane lies between the glomerulus and Bowman’s capsule and acts as the only barrier within the nephron. This ultrafiltration leads to high filtration pressure called the hydrostatic pressure (see Table 18.1). The glomeruli selectively filter the substrate, despite greater permeability compared to the regular capillaries [Reference Preuss1]. It is impermeable to larger-sized plasma proteins but effectively filters dissolved substances [Reference Preuss1]. Water, electrolytes, and glucose are reabsorbed, along with amino acids. The nephron tubules accompany peritubular capillaries that allow movement of various solutes from the tubules to peritubular capillaries [Reference Preuss1].Transport of these solutes occurs via active transport that requires energy consumption, as in the case of sodium reabsorption (see Table 18.1). If active transport is coupled with another substrate, for example, sodium and glucose, then it is termed cotransport. When positive ions are actively transported, negative ions follow to maintain electrical neutrality, such as chloride and urea (see Table 18.2) [Reference Preuss1]. Passive mechanism moves solutes across their gradients.
Table 18.1 Reabsorbed substances and transporters
Reabsorbed substance | Segment | Transporters |
---|---|---|
Water |
|
|
Sodium |
|
|
Chloride |
|
|
Calcium |
|
|
Bicarbonate |
|
|
Phosphate |
|
|
Glucose |
|
|
Table 18.2 Secreted substances and transporters
Secreted substance | Segment | Transporters |
---|---|---|
Potassium |
|
|
Hydrogen ions |
|
|
Urea |
|
|
Anesthesia for Kidney Transplant
With advances in immunosuppression and use of immunoglobulins since the 1960s, use of allografts has had more successful outcomes with living donor grafts than with cadaver donors. End-stage renal disease (ESRD) (with the most common etiologies being diabetes mellitus, hypertension, and glomerular diseases) affects multisystem physiology, and its effects on the cardiovascular system account for approximately 50% mortality. Thorough knowledge of changes in multisystem physiology is important [Reference Baxi, Jain and Dasgupta2]. A comprehensive preoperative examination, laboratory tests, human leukocyte antigen (HLA) typing and crossmatching, hemodialysis within 24 hours, volume status, and evaluation of cardiac function are of prime importance. Intraoperatively, standard American Society of Anesthesiologists (ASA) monitors, with invasive blood pressure and central venous pressure (CVP) monitoring, are routinely utilized. Intraoperative arteriovenous fistula monitoring is done every 15 minutes. Propofol is utilized as a standard induction agent and cisatracurium is a mainstay muscle relaxant. Succinylcholine should be used for rapid sequence induction only if a patient has a recent normal potassium level. Fentanyl is the main opioid utilized, whereas morphine, hydromorphone, and meperidine are avoided due to accumulation of secondary metabolites. Desflurane, isoflurane, and total intravenous anesthesia (TIVA) are commonly utilized; however, sevoflurane is also commonly utilized, even though there is a theoretical risk of compound A resulting in renal injury in a dose-dependent manner, as shown in animal models [Reference Mittel and Wagener3]. Potassium-containing fluids are generally avoided. Postoperatively, patients can be extubated and rarely require critical care unit monitoring unless indicated [Reference Baxi, Jain and Dasgupta2]. Numerous drugs have been utilized for immunosuppression to revert graft rejection, namely cyclosporin, sirolimus, interleukin 2, daclizumab, and azathioprine. Induction (first phase) of immunosuppressants is started a week before transplant, and maintenance (second phase) is utilized to prevent graft rejection for 3–6 months. Long-term immunosuppression (third phase) is utilized life-long. A commonly used regimen includes tacrolimus as the prime drug for maintenance and mycophenolate for adjunctive oral maintenance. Methylprednisolone is used as an adjunct to the induction regimen. Thymoglobulin is used both for induction and for acute rejection, and basiliximab is utilized in the immediate period following transplantation [Reference Baxi, Jain and Dasgupta2].
Regional Anesthesiology for End-Stage Renal Disease and Chronic Kidney Disease
Given hematologic abnormalities in ESRD, including abnormal coagulation profile, dysfunctional platelets, heparin use, electrolyte abnormalities, and acid–base abnormalities, regional anesthesia for transplant is commonly utilized [Reference Baxi, Jain and Dasgupta2]. A combined spinal epidural technique can provide supplemental analgesia, but most facilities have utilized general anesthesia for predictable anesthetic depth during renal transplant [Reference Baxi, Jain and Dasgupta2]. There is also a potential for local anesthetic toxicity, especially in plexus volume blocks where larger doses of local anesthetic are utilized. No difference has been found in patients with ESRD and normal individuals with regard to optimally placed nerve block duration and quality. Brachial plexus blocks are commonly utilized for arteriovenous fistula surgery. At the authors’ institution, a combination of 15 mL of 0.5% ropivacaine and 15 mL of 1.5% mepivacaine is commonly utilized for preoperative supraclavicular block in arteriovenous fistula surgery. If the block is patchy or incomplete, general anesthesia is reverted to with use of endotracheal tube placement or TIVA, with similar goals and concerns for general anesthesia in renal transplant patients.
Perioperative Management in Clinical Renal Conditions
End-Stage Renal Disease
High morbidity and mortality rates constantly plague ESRD patients, decreasing successful health or surgical outcomes. In comparison to those with normal-functioning kidneys, ESRD patients who undergo dialysis have longer mechanical ventilation times, longer intensive care unit (ICU) stays, and increased stays at the hospital [Reference Akdemir, Oztekin and Doluoglu4]. Before taking the patient to surgery, dialysis should be performed typically on the previous day and blood work should corroborate (if possible) hemodynamic stability. Hyperkalemia, volume overload, and glycemic control should be monitored prior to surgery, and clinicians should take note of changes in laboratory values [Reference Akdemir, Oztekin and Doluoglu4]. ESRD patients have decreased renal filtration and excretion of metabolites, so pharmacokinetics and pharmacodynamics should be monitored by the anesthesiologist perioperatively [Reference Carlo, Phisitkul, Phisitkul, Reddy and Amendola5]. Perioperative management of ESRD patients under general anesthesia should also take into account disease pathophysiology, involving cardiovascular dysfunction, fluid levels, electrolyte imbalances, anemia, and other complications [Reference Dhondup, Kittanamongkolchai and Vaughan6]. The anesthetic technique that is implemented depends on the functional status of the patient, coagulation changes, surgical necessity, and the preferences of the multidisciplinary team [Reference Carlo, Phisitkul, Phisitkul, Reddy and Amendola5]. Postoperative problems increase with age, signaling that the condition deteriorates due to the patient’s physical status and overall health. Restarting dialysis postoperatively and administering medications, such as heparin for blood clotting, help to restore normal functions and reduce the risk of bleeding, pain, or other complications [Reference Carlo, Phisitkul, Phisitkul, Reddy and Amendola5].

Full access? Get Clinical Tree
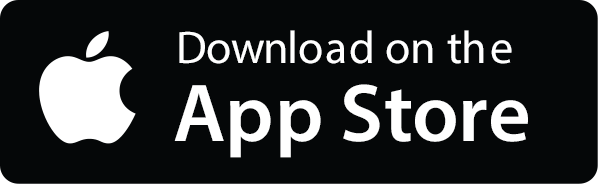
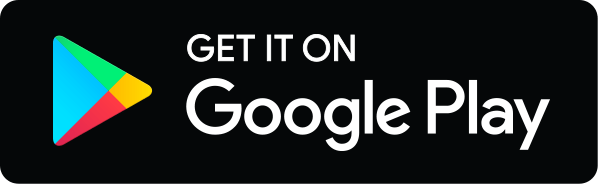
