Abstract
The heart is composed of pacemaker, conducting and contractile tissue. Each has a different action potential morphology allowing the heart to function as a coordinated unit.
The sino-atrial (SA) node is in the right atrium, and of all cardiac tissue it has the fastest rate of spontaneous depolarisation so that it sets the heart rate. The slow spontaneous depolarisation (pre-potential or pacemaker potential) of the membrane potential is due to increased Ca2+ conductance (directed inward). At −40 mV, slow voltage-gated Ca2+ channels (L channels) open, resulting in membrane depolarisation. Na+ conductance changes very little. Repolarisation is due to increased K+ conductance while Ca2+ channels close (see Figure 15.1a).
Physiology
Cardiac Action Potential
The heart is composed of pacemaker, conducting and contractile tissue. Each has a different action potential morphology allowing the heart to function as a coordinated unit.
The sino-atrial (SA) node is in the right atrium, and of all cardiac tissue it has the fastest rate of spontaneous depolarisation so that it sets the heart rate. The slow spontaneous depolarisation (pre-potential or pacemaker potential) of the membrane potential is due to increased Ca2+ conductance (directed inward). At −40 mV, slow voltage-gated Ca2+ channels (L channels) open, resulting in membrane depolarisation. Na+ conductance changes very little. Repolarisation is due to increased K+ conductance while Ca2+ channels close (see Figure 15.1a).
Contractile cardiac tissue has a more stable resting potential at −80 mV. Its action potential has been divided into five phases (see Figure 15.1b):
Phase 0 – describes the rapid depolarisation (duration < 1 millisecond) of the membrane, resulting from increased Na+ (and possibly some Ca2+) conductance through voltage-gated Na+ channels.
Phase 1 – represents closure of the Na+ channels while Cl− is expelled.
Plateau phase 2 – due to Ca2+ influx via voltage-sensitive type-L Ca2+ channels and lasts up to 150 milliseconds. This period is also known as the absolute refractory period in which the myocyte cannot be further depolarised. This prevents myocardial tetany.
Phase 3 – commences when the Ca2+ channels are inactivated and there is an increase in K+ conductance that returns the membrane potential to its resting value. This period is also known as the relative refractory period in which the myocyte requires a greater than normal stimulus to provoke a contraction.
Phase 4 – during this the Na+/K+ ATPase maintains the ionic concentration gradient at about −80 mV, although there will be variable spontaneous ‘diastolic’ depolarisation.
Arrhythmias
Tachyarrhythmias
These may originate from enhanced automaticity where the resting potential of contractile tissue loses its stability and may reach its threshold for depolarisation before that of the SA node. This is seen during ischaemia and hypokalaemia.
Ischaemic myocardium may result in oscillations of the membrane potential. These after-potentials may reach the threshold potential and precipitate tachyarrhythmias.
Re-entry or circus mechanisms describe how an ectopic focus may originate, leading to tachyarrhythmias (see Figure 15.2).
Figure 15.1 Action potentials of (a) pacemaker and (b) contractile tissue.
Figure 15.2 Atrioventricular nodal re-entrant tachycardia (action potential followed by refractory period). In this situation, there are two anatomically and physiologically distinct conduction pathways within the atrioventricular node (AVN). The fast pathway has a long refractory period, whereas the slow pathway has a short refractory period. (a) A normal atrial action potential (AP) travels at different velocities through the two pathways. The AP in the slow pathway arrives at the refractory final common pathway and is therefore terminated (b). (b) also demonstrates how the slow pathway recovers from its refractory state more quickly than the fast pathway. If a premature atrial impulse arrives at the origins of the two pathways and finds the fast pathway still refractory, it will only travel down the slow pathway (c). Because it travels slowly down the slow pathway it is not terminated by refractory tissue as in (b) and may travel on into the ventricles but also retrogradely up the fast pathway (d). Because of the short refractory period of the slow pathway the impulse may travel down the slow pathway (e) to continue the circus movement thereby generating the self-perpetuating tachycardia. The atrioventricular re-enterant tachycardia seen in Wolff–Parkinson–White (WPW) syndrome is generated in a similar manner except that the accessory pathway (bundle of Kent) is distinct from the atrioventricular node (AVN).
Classification of Anti-Arrhythmics
Traditionally anti-arrhythmics have been classified according to the Vaughan–Williams classification (see Table 15.1). However, it does not include digoxin and more recently introduced drugs such as adenosine. In addition, individual agents do not fall neatly into one category, e.g. sotalol has class I, II and III activity.
Table 15.1 Vaughan–Williams classification
Class | Mechanism | Drugs |
---|---|---|
Ia | Na+ channel blockade – prolongs the refractory period of cardiac muscle | quinidine, procainamide, disopyramide |
Ib | Na+ channel blockade – shortens the refractory period of cardiac muscle | lidocaine, mexiletine, phenytoin |
Ic | Na+ channel blockade – no effect on the refractory period of cardiac muscle | flecainide, propafenone |
II | β-Adrenoceptor blockade | propranolol, atenolol, esmolol |
III | K+ channel blockade | amiodarone, bretylium, sotalol |
IV | Ca2+ channel blockade | verapamil, diltiazem |
Anti-arrhythmics may also be divided on the basis of their clinical use in the treatment of:
Supraventricular tachyarrhythmias (SVT) (digoxin, adenosine, verapamil, β–blockers, quinidine)
Ventricular tachyarrhythmias (VT) (lidocaine, mexiletine)
Both SVT and VT (amiodarone, flecainide, procainamide, disopyramide, propafenone, sotalol)
Digoxin toxicity (phenytoin).
Supraventricular Tachyarrhythmias
Digoxin
Presentation
Digoxin is a glycoside that is extracted from the leaves of the foxglove (Digitalis lanata) and is available as oral (tablets of 62.5–250 µg, elixir 50 µg.ml−1) and intravenous (100–250 µg.ml−1) preparations. The intramuscular route is associated with variable absorption, pain and tissue necrosis.
Uses
Digoxin is widely used in the treatment of atrial fibrillation and atrial flutter. It has been used in heart failure but the initial effects on cardiac output may not be sustained and other agents may produce a better outcome. It has only minimal activity on the normal heart. It should be avoided in patients with ventricular extrasystoles or ventricular tachycardia (VT) as it may precipitate ventricular fibrillation (VF) due to increased cardiac excitability.
Treatment starts with the administration of a loading dose of between 1.0 and 1.5 mg in divided doses over 24 hours followed by a maintenance dose of 125–500 µg per day. The therapeutic range is 1–2 µg.l−1.
Mechanism of Action
Digoxin has direct and indirect actions on the heart.
Direct – it binds to and inhibits cardiac Na+/K+ ATPase leading to increased intracellular Na+ and decreased intracellular K+ concentrations. The raised intracellular Na+ concentration leads to an increased exchange with extracellular Ca2+ resulting in increased availability of intracellular Ca2+, which has a positive inotropic effect, increasing excitability and force of contraction. The refractory period of the atrioventricular (AV) node and the bundle of His is increased and the conductivity reduced.
Indirect – the release of acetylcholine (ACh) at cardiac muscarinic receptors is enhanced. This slows conduction and further prolongs the refractory period in the AV node and the bundle of His.
In atrial fibrillation the atrial rate is too high to allow a 1:1 ventricular response. By slowing conduction through the AV node, the rate of ventricular response is reduced. This allows for a longer period of coronary blood flow and a greater degree of ventricular filling so that cardiac output is increased.
Side Effects
Digoxin has a low therapeutic ratio and side effects are not uncommon:
Cardiac – these include various arrhythmias and conduction disturbances – premature ventricular contractions, bigemini, all forms of AV block including third-degree block, junctional rhythm and atrial or ventricular tachycardia. Hypokalaemia, hypercalcaemia or altered pH may precipitate side effects. The ECG signs of prolonged PR interval, characteristic ST segment depression, T wave flattening and shortened QT interval are not signs of toxicity.
DC cardioversion – severe ventricular arrhythmias may be precipitated in patients with toxic levels and it is recommended to withhold digoxin for 24 hours before elective cardioversion.
Non-cardiac – anorexia, nausea and vomiting, diarrhoea and lethargy. Visual disturbances (including deranged red–green colour perception) and headache are common while gynaecomastia occurs during long-term administration. Skin rashes are rarely seen and may be accompanied by an eosinophilia.
Interactions – plasma levels are increased by amiodarone, captopril, erythromycin and carbenoxolone. They are reduced by antacids, cholestyramine, phenytoin and metoclopramide. Ca2+ channel antagonists produce variable effects; verapamil will increase, while nifedipine and diltiazem may have no effect or produce a small rise in plasma levels.
Kinetics
The absorption of digoxin from the gut is variable depending on the specific formulation used, but the oral bioavailability is greater than 70%. It is about 25% plasma protein-bound and has a volume of distribution of 5–10 l.kg−1. Its volume of distribution is significantly increased in thyrotoxicosis and decreased in hypothyroidism. It undergoes only minimal hepatic metabolism, being excreted mainly in the unchanged form by filtration at the glomerulus and active tubular secretion. The elimination half-life is approximately 35 hours but is increased significantly in the presence of renal failure.
Toxicity
Plasma concentrations exceeding 2.5 µg.l−1 are associated with toxicity although serious problems are unusual at levels below 10 µg.l−1. Despite these figures the severity of toxicity does not correlate well with plasma levels. However, a dose of more than 30 mg is invariably associated with death unless digoxin-specific antibody fragments (Fab) are used.
Treatment of Digoxin Toxicity
Gastric lavage should be used with caution as any increase in vagal tone may precipitate further bradycardia or cardiac arrest. Owing to Na+/K+ ATPase inhibition, hyperkalaemia may be a feature and should be corrected. Hypokalaemia will exacerbate cardiac toxicity and should also be corrected. Where bradycardia is symptomatic, atropine or pacing is preferred to infusions of catecholamines, which may precipitate further arrhythmias. Ventricular arrhythmias may be treated with lidocaine or phenytoin.
If plasma levels rise above 20 µg.l−1, there are life-threatening arrhythmias or hyperkalaemia becomes uncontrolled, digoxin-specific Fab are indicated. These are IgG fragments. Digoxin is bound more avidly by Fab than by its receptor so that it is effectively removed from its site of action. The inactive digoxin–Fab complex is removed from the circulation by the kidneys. There is a danger of hypersensitivity or anaphylaxis on re-exposure to digoxin-specific Fab.
Adenosine
Adenosine is a naturally occurring purine nucleoside consisting of adenine (the purine base) and d-ribose (the pentose sugar), which is present in all cells.
Presentation
Adenosine is presented as a colourless solution in vials containing 3 mg.ml−1. It should be stored at room temperature.
Uses
Adenosine is used to differentiate between SVT, where the rate is at least transiently slowed, and VT, where the rate does not slow. Where SVT is due to re-entry circuits that involve the AV node, adenosine may convert the rhythm to sinus. Atrial fibrillation and flutter are not converted by adenosine to sinus rhythm as they are not generated by re-entry circuits involving the AV node, although its use in this setting will slow the ventricular response and aid ECG diagnosis.
Mechanism of Action
Adenosine has specific actions on the SA and AV node mediated by adenosine A1 receptors that are not found elsewhere within the heart. These adenosine-sensitive K+ channels are opened, causing membrane hyperpolarisation, and Gi-proteins cause a reduction in cAMP. This results in a dramatic negative chronotropic effect within the AV node.

Full access? Get Clinical Tree
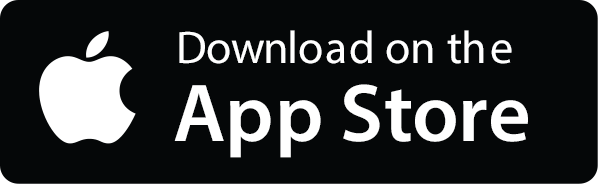
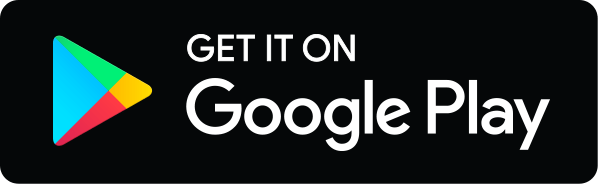
