Introduction
The taxonomy of disorders of consciousness encountered in the perioperative period is in the state of flux. So, rather than formally defining emergence delirium, I would like to start with a particularly colorful description of the phenomenon by Shoum (1): “Immediately upon a gentle, unstimulated awakening, the patient became disoriented, combative, and agitated. He ripped off the electrocardiogram electrodes, the blood pressure cuff, and the IV catheter and ran from the procedure room … After 15 minutes of continuously attempting to communicate with him as we ran, pursued by the entire security department and hospital chaplain, among others, 2 police vehicles responded. He then recognized his wife’s best friend and neighbor who was there to drive him home … After she reoriented him and accompanied him to the postanesthesia care unit, he described seeing her in a small expanding circle of light, in an otherwise black nightmare. He asked why his fists were bleeding and if he had fallen down, and I explained what had transpired; he apologized profusely.” While this clearly represents an extreme case of emergence delirium and may have been amplified by preexisting posttraumatic stress disorder (1); agitation, confusion, and dissociative reactions are not uncommon upon emergence from anesthesia. This chapter will specifically focus on these phenomena observed immediately in the postanesthetic period. I will collectively refer to these phenomena as emergence delirium. After reviewing some clinical literature on emergence delirium, I will propose a hypothesis that may explain why it is so commonly encountered upon emergence from anesthesia.
Delirium can be observed during any portion of the postoperative course and may be associated with long-term morbidity (2). Delirium is also common in hospitalized elderly patients who do not have surgery or anesthesia (3). It is likely that incidence and severity of delirium is multifactorial. Both predisposing factors such as preexisting cognitive dysfunction, age, and medical illness as well as precipitating factors such as exposure to specific drugs, need for intensive care, sleep deprivation, and many others likely contribute to the etiology of delirium in hospitalized patients (4). If there is a case to be made for the direct involvement of anesthesia in delirium, however, it is most compelling for the delirium which immediately follows emergence. This is why our focus here is on this subtype of delirium.
One of the earliest systematic studies of emergence delirium by Smessaert et al. (5) classified recovery from anesthesia in children (>10 years of age) and adults into three groups ranging from calm uneventful recovery (1) to marked agitation delirium and uncooperative behavior (3). They found that approximately 2.8% of all patients were markedly delirious. This early work identified two factors that were associated with postanesthetic delirium: anesthetic agent and the type of operation. Another pioneering work by Eckenhoff et al. (6) characterized “emergence excitement” as sobbing, thrashing, and disorientation. In contrast to Smessaert et al., this study also included children 3 years of age and older. Eckenhoff et al. provided one of the very first experimental demonstrations that the incidence of emergence delirium is inversely proportional to age. Both Smessaert et al. (5) and Eckenhoff et al. (6) identified cyclopropane and barbiturates as anesthetic agents most likely to be associated with emergence delirium. While neither one of these is in wide clinical use today, emergence delirium remains a common clinical problem. This suggests that, while there may be anesthetic agent related factors that make delirium more or less likely, delirium is a common feature of recovery from anesthesia induced with mechanistically distinct anesthetic agents.
Review of Clinical Literature
Emergence delirium is most common in children. Cole et al. (7) estimate that up to 30% of children between 6 months and 10 years of age exhibit agitation immediately upon emergence from anesthesia some 40 years after the study by Eckenhoff et al. (6). In fact, the incidence in children between 3 and 10 years old estimated by Voepel-Lewis et al. (8) to be 18% is essentially identical to that estimated by Eckenhoff et al. (6) to be 13% in the same age group.
Because of higher incidence, emergence delirium has received significant attention in the pediatric population. Yet, consistent with early literature (5,6) it continues to affect adults. Munk et al. (9), Eckenhoff et al. (6), and Smessaert et al. (5) estimate that the incidence of delirium in adults is 3.7%. Lepouse (10) uses Riker agitation scale to determine the incidence of acute agitation upon emergence from anesthesia in an adult population and determined it to be 4.7%. Approximately one-quarter of these were found to be dangerously agitated (Riker Scale of 7). Yu et al. (11) find a significantly higher incidence of agitation (21%). Yet severe agitation that required restraints estimated by Yu et al. (11) and Lepouse (10) were similar at ~1%–2% of patients. Remarkably, Yu et al. (11) found that use of doxapram, a central nervous system (CNS) stimulant, was associated with a 63% incidence of agitation, which the authors felt was precipitated by abrupt reversal of anesthesia. Kim et al. (12) also find high incidence of agitation (22%) in adults undergoing nasal surgery. In the study by Kim et al. (12) extreme agitation defined by Richmond Agitation Sedation Scale (RASS) score of 4 was significantly lower at 3.4%. Note again that this incidence in adults is consistent with Smessaert et al. (5) who estimate the incidence to be between 9% (in teenagers) and 2.4% in patients older than 70 and approximately 3% in adults. Again, note that despite the fact that anesthetic agents, modes of anesthesia administration, and surgical techniques have undergone dramatic changes over the past 50 years, not much difference in the incidence of agitation has been observed.
Munk et al. (9), Eckenhoff et al. (6), and Smessaert et al. (5) all focus on the hyperactive form of delirium akin to that described so vividly by Shoum (1). Yet agitation is not the only sign of emergence delirium. While agitation attracts more medical personnel and may result in immediately obvious consequences such as pulling out IVs (9) hypoactive aspects of delirium may go unnoticed and may even pass for the desired “smooth emergence.” Radtke et al. (13) focused on what they referred to as “hypoactive emergence” defined by RASS less than or equal to −2 and estimated its incidence to be around 3%. Hypoactive emergence was associated with longer stays.
Many different anesthetic and sedative drugs have been associated with postanesthetic delirium. Doxapram, a CNS stimulant, has been mentioned above (11). Benzodiazepines are known to be associated with delirium in the intensive care unit, but it is not clear to what degree benzodiazepines contribute to emergence delirium (14). Both pain (e.g., 15) and increased doses of opioids (e.g., 16) were associated with postoperative delirium. Some studies (e.g., 9,11) suggest that use of volatile anesthetics was associated with greater incidence of emergence delirium than intravenous agents, while others (10,13) have failed to find a convincing association between the choice of anesthetic technique and incidence of delirium. At least in part the differences between different clinical trials are likely due to the differences in the definitions and assessment techniques used to estimate incidence and quantify severity of emergence delirium (17). In order to make any meaningful comparisons between anesthetic regimens or other aspects of perioperative care such as pain management, standard, versatile, and clinically meaningful assessment of delirium would have to emerge. That being said, there appears to be consensus among all studies that have been performed over the course of several decades that many mechanistically distinct anesthetics can lead to emergence delirium.
Novel Hypothesis for Emergence Delirium
Why should anesthetics lead to disruption of normal cognition upon emergence? One possibility is that because many anesthetics are promiscuous on the molecular level (18), there may be a subset of molecular targets common to many different agents and that actions of anesthetics on these targets contribute to emergence delirium. Just like there are likely many distinct molecular pathways that can be altered to produce the state of anesthesia, there are likely to be many different molecular pathways that contribute to emergence delirium. Finally, there are quite likely idiosyncrasies of neuronal architecture and physiology in different patients that may predispose, or conversely protect, them from emergence delirium. While it is likely that all of the above factors are relevant for emergence delirium, here we will focus on a novel hypothesis that proposes a parsimonious explanation for why emergence delirium is so prevalent and why despite a true revolution in anesthetic drugs and techniques the incidence of emergence delirium remains relatively unchanged.
The basic tenet of this hypothesis is that recovery of consciousness after anesthesia must be structured and that alteration of this structure manifests as a variety of phenotypes that are together known as emergence delirium. To explain what I mean by structure, I will start with the nearly uniformly held views concerning the etiology of general anesthesia. Brown et al. (19) define the state of anesthesia as “drug-induced, reversible condition composed of the behavioral states of unconsciousness, amnesia, analgesia, and immobility.” Multiple lines of evidence converge on the fact that anesthetics interact with specific molecular targets in order to give rise to the state of anesthesia (20,21–25). Indeed, our understanding of how to induce and maintain the state of anesthesia is grounded in pharmacokinetic and pharmacodynamic principles (26) which state that the observed effect (“depth of anesthesia”) is directly related to the concentration of the anesthetic agent at the “effect site.” We are used to characterizing anesthetic drugs on the basis of quantities such as potency reflected in the familiar concentration-response curve (Figure 1.1A). There are, however, several lines of evidence that converge on the fact that knowing pharmacologic quantities such as drug concentration, affinity, and efficacy is insufficient to explain the state of anesthesia.
Figure 1.1 Novel hypothesis for emergence delirium. Standard PK-PD approach maintains that the concentration of the anesthetic agent determines the effect of this agent (e.g., depth of anesthesia) through the concentration-response curve (A). Experiments by Kelz and colleagues (27–29) demonstrate that the concentration-response curve for induction is consistently right shifted with respect to concentration-response curve for emergence (B). The area between the induction and emergence curves (gray shading in B) implies that knowing the anesthetic concentration is not sufficient to specify the response. C. Illustrates the Levinthal’s “paradox” of protein folding. It is not clear how, once the three-dimensional structure of the protein is denatured, the unfolded polypeptide chain searches the conformation space in order to refold. D. Illustrates that Levinthal’s paradox could apply to the brain recovering consciousness after anesthesia. In order to rediscover brain activity patterns compatible with normal consciousness and cognition, the brain must navigate an essentially infinite space of activity patterns. One possibility consistent with experimental evidence (e.g., 38), much like refolding protein, is the brain samples just a few discrete metastable activity patterns en route to recovery. Alterations in neuronal mechanisms that are responsible for state stabilization and transitions between states will therefore dramatically alter recovery trajectory and may impede a prompt return of normal consciousness and cognition.
Consider a situation that arises in operating rooms all over the world countless times every day. At the end of surgery as incisions are being dressed, the end tidal anesthetic concentration is gradually reduced and neuromuscular blockade is reversed. The patient is breathing spontaneously but their eyes are closed. They do not seem to be bothered by the usually noxious presence of an endotracheal tube and are oblivious to the events around them. Once surgical drapes come down, the anesthesiologist taps the patient on the shoulder and calls their name. This prompts the patient to immediately open their eyes. Commonly, awakening is associated with coughing induced by the endotracheal tube. Upon extubation the patient is able to follow some simple commands, and occasionally able to ask questions concerning the surgery. The patient has clearly made a state transition from being unconscious to being awake. Yet, because this transition is so abrupt, the concentration of anesthetic at the “effect site” could not have changed to any appreciable degree. This everyday observation suggests that knowing the anesthetic concentration is not enough to determine how deeply the patient is anesthetized.
Indeed, careful experimental work in animals by Kelz and colleagues (27–29), confirms this observation. Using state of the art techniques of measuring anesthetic concentration in the brain – the target organ of anesthetics – the authors confirmed that the dose-response curve for induction of and emergence from anesthesia are distinct (Figure 1.1B; reviewed in 30). Some human evidence for anesthetic hysteresis has been found by Warnaby et al. (31). Yet the evidence for hysteresis in the study by Kuizenga et al. (32) was underwhelming. While controlling for pharmacokinetic variables in human trials is a daunting task (33), animal literature obtained across taxa from fruit fly to mammals strongly argues for existence of hysteresis. The consistent differences between the concentration-response curves for induction and emergence imply that knowing the anesthetic concentration is not enough. Some other nonpharmacologic variables must play a role.
Why should this be? I will argue that this asymmetry between induction and emergence from anesthesia is in fact a generic property that has very little to do with anesthetics per se but is a direct consequence of the fact that the brain is a complex system. To illustrate this point, I would like to turn to a seemingly unrelated problem: protein folding. Nobel laureate Christian Anfinsen proposed that, at least for small globular proteins, the native three-dimensional conformation is dictated solely by the amino acid sequence (34). This observation was based on the fact that one can denature (e.g., by heating) small proteins, and watch them recover their native fold (Figure 1.1C). This set of observations prompted Cyrus Levinthal to perform an interesting thought experiment (35). Consider a 100 amino acid protein. This protein has 99 peptide bonds and therefore 98 different phi and psi bond angles. If one bins the possible values of these angles into just three bins, the number of protein conformations is 3198. If we assume that the protein folds by randomly going from one conformation to the next until it stumbles upon the correct conformation, protein folding would take longer than the age of the known universe even if the protein could switch conformations every picosecond. This thought experiment is known as Levinthal’s paradox.
A similar paradox can be stated for the brain recovering consciousness after anesthesia. What specifically allows the brain to exhibit consciousness is a matter of enduring mystery (36). Few seriously doubt, however, that consciousness has a fundamental connection to the structure and activity of the brain. Yet, clearly randomly connecting neurons in a culture dish do not result in anything even remotely as complex and functional as the waking brain much like a flailing unfolded polypeptide chain is unlikely to catalyze any useful chemical process. Thus, it is fair to say that, while we are not sure what specifically allows the brain to give rise to conscious experience, the specific combination of parameters (e.g., synaptic strength and biophysical characteristics of individual neurons) is not likely to occur by chance. Therefore, within the very large universe of possible states of the brain (akin to many possible states of unfolded protein) there exists only a small region where consciousness can manifest (Figure 1.1C). Induction of anesthesia is therefore movement of the brain from within the “consciousness region,” to the vast universe of possible configurations which do not give rise to consciousness (i.e., are operationally defined as anesthetized). Yet, in order for consciousness to recover, the brain must somehow navigate this vast universe to return to the small region of the parameter space where consciousness can once again manifest. Note that this argument is exactly isomorphic to the thought experiment by Levinthal. Thus, removal of the anesthetic from the brain is no more of an explanation of recovery of consciousness than lowering the temperature is an explanation of how proteins are able to refold after being denatured.
It should be stated that the term paradox is somewhat of a misnomer for Levinthal’s thought experiment. There is nothing paradoxical about protein folding. Rather Levinthal’s gedanken experiment points to the necessity of structure that informs protein folding. Indeed, proteins do not find their final three-dimensional conformation by haphazardly going from one conformation to the next. Instead, proteins are thought to fold through a series of intermediates that are transiently stabilized (Figure 1.1D). For instance, perhaps an alpha helix could form, then another one, then these alpha helices would form a bundle. In fact, something similar to this happens in the brain during recovery from anesthesia. To demonstrate this, we recorded local field potentials (local versions of the electrical signals recorded in electroencephalogram in human research) in rats during recovery from isoflurane (37,38). What we observed was a stark departure from the predictions of the pharmacology-centric view of anesthesia. Even when the anesthetic concentration was fixed, the brain spontaneously transitioned between several discrete patterns of activity. Furthermore, we showed that not all state transitions were possible. As a consequence of these state transitions, recovery of consciousness much more closely resembled booting of a computer or a folding protein, rather than gradual washout of the anesthetic from the effect site. In a complementary set of experiments performed on humans (39) and nonhuman primates (40) we demonstrated using a novel analysis technique applied to electrocorticography that during loss of consciousness induced with anesthetics, neuronal dynamics become stabilized. Together the stabilization of neuronal dynamics, and spontaneous, presumably noise-induced transitions between different discrete activity patterns, greatly shrink the space of all possible neuronal activity patterns. These stabilized activity patterns make up a scaffold that allows the brain to recover consciousness on a physiological time scale much like stabilization of folding intermediates allows a protein to recover its natural three-dimensional conformation after a perturbation.
Observations made by others in clinical studies and laboratory investigation performed in a variety of animal model systems are consistent with this hypothesis. Chander et al. (41) show that EEG patterns can undergo abrupt transitions during recovery of consciousness after anesthesia and that certain activity patterns observed during recovery can be associated with postoperative pain. Kafashan et al. (42) demonstrate using functional magnetic resonance imaging (fMRI) in human volunteers that functional connectivity measured using correlations in blood oxygen level-dependent (BOLD) signal fluctuates during stable sevoflurane concentration. Lee et al. (43) observed abrupt changes in the network properties inferred from high density EEG in human subjects receiving propofol. Ishizawa et al. (44) demonstrate discontinuous state transitions in neuronal dynamics in nonhuman primates during loss of consciousness. We (30) demonstrated using a simple mathematical model that the state transitions observed by us and by others can indeed give rise to hysteresis observed between concentration-response curves for induction and emergence.
We are currently actively investigating the neuronal mechanisms involved in stabilizing different activity patterns and allowing state transitions between them. Regardless of the specific details, however, a combination of empirical observations made in a variety of animal models, human volunteers, and patients converges on the fact that recovery of consciousness after anesthesia must be structured. This structure is predicted by the very fact that the brain is a complex nonlinear dynamical system. Quite generally, recovery from strong perturbations in such systems is not guaranteed (45). The very fact that millions of patients have recovered consciousness after anesthesia is a testament to the robustness of the mechanisms which allow recovery of consciousness.
Yet, it is easy to see that alterations in these mechanisms can give rise to abnormal recovery patterns which, in the clinical settings, might manifest as delirium. Indeed, recent clinical evidence suggests that neuronal activity patterns observed under anesthesia can predispose to subsequent delirium. Recent studies (46–48) suggest that monitoring and controlling “depth of anesthesia” may decrease the incidence of postoperative delirium. Preliminary results by Whitlock et al. (49) in cardiothoracic surgical patients failed to reach statistical significance, but indicated a trend toward reduction of delirium in patients whose anesthetic was titrated to have Bispectral Index (BIS) values in the 40–60 range. In an observational study (50) an association between intraoperative burst suppression and delirium after cardiac surgery was found. Another study (51) concurs that duration of suppression observed intraoperatively correlates positively with incidence of postoperative delirium. Furthermore, Fritz et al. (52) suggest that burst suppression at lower anesthetic concentration is particularly significant for postoperative delirium. These previous studies focus on burst suppression (or equivalently low BIS scores) simply because burst suppression is trivially identified in neuronal recordings. While it is possible that burst suppression specifically may be associated with abnormal recovery, it is far more likely that detailed investigation of neuronal dynamics during recovery of consciousness coupled with detailed mechanistic investigation of the neuronal processes that allow consciousness to recover will together lead to a much better understanding of emergence delirium and perhaps suggest clinical strategies which will help avoid this common complication.

Full access? Get Clinical Tree
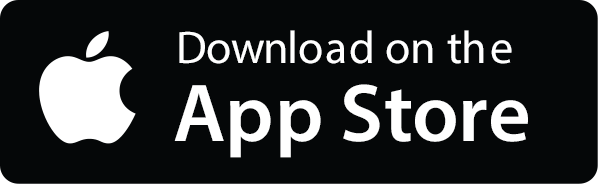
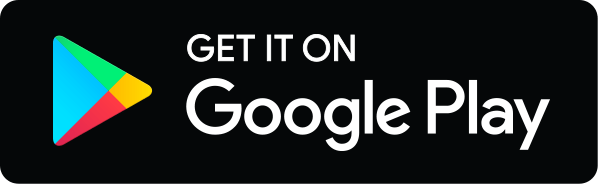