Questions
- 1.
What is subarachnoid hemorrhage?
- 2.
How is subarachnoid hemorrhage diagnosed?
- 3.
How are patients with subarachnoid hemorrhage managed?
- 4.
How is elevated intracranial pressure diagnosed and treated?
- 5.
What is traumatic brain injury?
- 6.
What are the risks of acute ischemic stroke, and how is stroke diagnosed and treated?
- 7.
How common are seizures after head injury, and how are they treated?
- 8.
What is encephalopathy, and how is it treated?
- 9.
A 35-year-old man sustained injuries in a motor vehicle accident and was taken to the nearest emergency department. His vital signs, x-rays, and laboratory values were normal. As his wounds were sutured, he complained of the worst headache of his life. His mental status acutely deteriorated. He was diagnosed with subarachnoid hemorrhage (SAH) and increased intracranial pressure (ICP), which were treated. After an uneventful course in the intensive care unit (ICU), he was transferred to the floor, where he experienced a seizure. After the seizure, he remained confused and combative. Later, he sustained an unwitnessed cardiac arrest from which he had return of spontaneous circulation after 30 minutes. However, he never regained consciousness.
1
What is subarachnoid hemorrhage?
SAH is the extravasation of blood into the subarachnoid space, which is located between the pia and the arachnoid membrane. The most common cause of SAH is traumatic head injury. Traumatic SAH in elderly adults often occurs after falls and head injury, whereas the most common cause in young people is motor vehicle accidents.
Nontraumatic SAH is most commonly associated with intracranial aneurysm rupture (approximately 80%–90%). Approximately 5% of the population harbor an aneurysm, and 20%–30% of people with an aneurysm have multiple aneurysms; however, very few aneurysms rupture spontaneously. Although SAH is responsible for about 5% of all strokes, the incidence of spontaneous SAH is only 25 per 100,000 in the United States. The peak incidence of aneurysmal SAH is between 50 and 60 years of age, with a higher prevalence in women and African Americans. Other nontraumatic causes include vascular malformations, tumors, and infections.
Risk factors for aneurysmal SAH are multifold, consisting of both modifiable and genetic factors. In the United States, smoking, hypertension, alcohol abuse, and cocaine abuse (especially in young people) are independent risk factors for SAH. Genetic diseases such as polycystic kidney disease and connective tissue diseases (e.g., Marfan syndrome, Ehlers-Danlos syndrome) increase the risk of developing aneurysms. In certain populations, such as the Japanese and Finnish, a familial syndrome is associated with intracranial aneurysms. The strongest risk factor for SAH is a history of previous SAH. There appears to be a higher incidence of SAH in winter and spring months as well as an association between atmospheric pressure changes and number of SAHs per day; the reasons are unknown.
2
How is subarachnoid hemorrhage diagnosed?
The symptom classically associated with aneurysmal SAH is described as “the worst headache of my life” and is reported in nearly 80% of awake patients. Many patients also report a sentinel headache, which generally occurs 2–8 weeks before a major SAH. Other symptoms include nausea, vomiting, pain radiating to the legs (blood pooling in lumbar cistern), nuchal rigidity, meningeal signs, cranial nerve palsies, focal neurologic symptoms, loss of consciousness, and seizures. Death occurs in 12% of people before receiving treatment.
Timely diagnosis is essential for SAH because prompt treatment has a significant impact on outcome. SAH is misdiagnosed in 12% of patients, leading to delayed treatment and is associated with a fourfold higher risk of death or disability at 1 year. Although SAH accounts for only 1% of all patients with headaches presenting to the emergency department, approximately 20% of all patients with SAHs present with sentinel headaches before major SAH. When a patient presents to the emergency department with a headache, a high index of suspicion for possible leak (sentinel headache) could prevent rerupture and be lifesaving.
Noncontrast computed tomography (CT) scan of the head is the diagnostic test of choice for acute SAH. The probability of detecting SAH by CT scan is related to time from SAH and severity of bleeding. The sensitivity of CT scans is nearly 100% within the first 12 hours after bleeding; sensitivity decreases to 90% at 24 hours and 60%–80% by days 5 and 6. The modified Fisher score, based on CT scan findings, is used to predict the likelihood of vasospasm ( Table 91-1 ). In patients with negative or inconclusive CT scan findings, examination of cerebrospinal fluid (CSF) with lumbar puncture for the presence of red blood cells or xanthochromia suggests the diagnosis of SAH. SAH is unlikely in patients with severe headache and a negative CT scan and lumbar puncture. Fluid attenuated inversion recovery (FLAIR) or proton density–weighted magnetic resonance imaging (MRI) can be used to diagnose SAH. However, because of cost, availability, and logistics of MRI, it is not widely used to diagnose acute SAH.
Grade | CT Scan Findings | Chance of Developing Vasospasm (%) |
---|---|---|
0 | No SAH or IVH | NA |
1 | Thin SAH, no IVH | 24 |
2 | Thin SAH with IVH | 33 |
3 | Thick SAH, no IVH | 33 |
4 | Thick SAH with IVH | 40 |
The “gold standard” for diagnosis of cerebral aneurysm as a cause of SAH is cerebral angiography. The advantages of cerebral angiography are better resolution of smaller aneurysms (<5 mm) and the ability to treat (coil) at the same time. Disadvantages of cerebral angiography are invasiveness requiring the proper facilities, skilled physicians, and large iodinated dye loads. CT angiography (CTA) is nearly as effective in diagnosing aneurysms that are >5 mm. For aneurysms <5 mm, the sensitivity is roughly 60%–80%. Magnetic resonance angiography (MRA) has been increasingly used to help diagnose the cause of SAH. However, MRA has major limitations including availability, cost, motion artifacts, and long duration making it unsuitable for unstable patients. Both CTA and MRA require interpretation by skilled radiologists and are diagnostic only.
3
How are patients with subarachnoid hemorrhage managed?
Evaluation of a patient with suspected SAH should start with the ABCs (airway, breathing, circulation) followed by a mental status examination. Although many patients with SAH present without airway compromise, the potential for neurologic deterioration from continued bleeding is significant. Continuous monitoring of mental status is extremely important because changes may herald loss of consciousness, inability to protect the airway, and respiratory failure requiring emergent intubation.
Examination for external signs of trauma helps differentiate traumatic versus nontraumatic causes of SAH. In the case of traumatic SAH, it is important to rule out other injuries that might require immediate treatment or that may complicate care (e.g., cervical spine injury, pneumothorax, tamponade, rupture of intraabdominal organs). A complete neurologic examination should also be performed.
Several clinical measurement scales have been used to estimate the severity and outcome of SAH. The Hunt and Hess grading scale, which is based on patients’ clinical condition, is the most widely used. It classifies severity and estimated risk of mortality after SAH ( Table 91-2 ).
Grade | Clinical Examination | Mortality (%) |
---|---|---|
1 | Mild headache Slight nuchal rigidity | 1 |
2 | Moderate-to-severe headache Severe nuchal rigidity Cranial nerve palsy | 5 |
3 | Mild focal deficit Lethargy Confusion | 19 |
4 | Stupor Moderate-severe hemiparesis Slight decerebrate rigidity | 40 |
5 | Coma Decerebrate rigidity Moribund | 77 |
When the diagnosis of a SAH is confirmed, further work-up is required to identify the etiology. Traumatic causes usually have a clear history of injury or are associated with stigmata such as scalp trauma or skull fracture. Nontraumatic SAHs are most likely due to cerebral aneurysms (approximately 80%–90%). Of aneurysms, 90% are located in the anterior circulation ( Box 91-1 ), and 10% are located in the posterior circulation. Other causes include arteriovenous malformation, intracranial dissection with pseudoaneurysm rupture, endocarditis with mycotic aneurysm rupture, meningitis, encephalitis, cavernous malformations, and cerebral vasculopathies.
The cornerstone of treatment for SAH is securing the aneurysm to control bleeding and prevent rebleeding. Throughout the 1980s, open surgical clipping was the only treatment available. In the 1990s, endovascular coiling using platinum coils was introduced. Endovascular coiling excludes aneurysms from the circulation by filling the aneurysm with platinum coils, which results in clot formation, decreasing the potential for rebleeding.
There is still controversy at the present time regarding whether open surgical clipping or endovascular coiling is superior. The decision whether to coil or clip is determined on a patient-by-patient basis taking into account the size, shape, and location of each aneurysm, patient condition, and the experience of available physicians. The International Subarachnoid Aneurysm Trial (ISAT) showed a statistically significant improvement in disability and death at 1 year in patients who had endovascular coiling compared with surgical clipping (24% vs. 31%). At 7-year follow-up, the surgical cohort had significantly higher mortality and seizure rates. However, early rebleeding was higher in the endovascular group. Although endovascular coiling has become more common and widely available, preference among centers is largely related to the culture, familiarity, and comfort of the facility with either clipping or coiling. Some recommendations suggest that surgical clipping is preferable for aneurysms >10 mm or aneurysms with wide necks.
Whether clipping or coiling is preferred at a particular institution, early treatment is recommended. In a follow-up article looking at the ISAT data, early intervention with either clipping or coiling resulted in improved outcomes. Past recommendations dictated that treatment should either occur early (0–3 days) or, if a patient’s condition warranted, be delayed (after 10 days). Intervention was not recommended between days 4 and 10 because of the increased risk for vasospasm. However, more recent studies have shown that intervention during days 5–10 yielded better outcomes than late interventions. The practice of delaying treatment is now questionable. Early intervention resulted in the best outcomes.
Medical management of SAH focuses on treating the complications of SAH. Rebleeding is the most treatable cause of poor outcomes. In unrepaired patients, the risk of rebleeding is 3%–4% within the first 24 hours, 2% on day 2, up to 20% within the first 2 weeks, and up to 50% by 6 months. Seizures are associated with increased risk of rebleeding in patients with untreated aneurysms. The only certain way to prevent rebleeding is aneurysmal clipping or coiling. Antifibrinolytics, such as aminocaproic acid (2–4 g loading dose, followed by 1 g/hour infusion) or tranexamic acid (1 g loading dose, followed by 1 g every 6–8 hours), have been used to prevent early rebleeding. However, because antifibrinolytics are associated with vasospasm, the current recommendation is to limit their use to the first 3 days. Both antifibrinolytics are to be employed cautiously in patients with histories of myocardial infarction, hypercoagulopathy, deep vein thrombosis, or pulmonary embolus. Lowering blood pressure with labetalol, nicardipine, or clevidipine has been shown to decrease risk of rebleeding.
Cerebral artery vasospasm can lead to worse mortality and disability in patients with SAH. It usually occurs between days 3 and 14 after SAH. Symptomatic vasospasm, defined as clinical deterioration secondary to vasospasm, is seen in 20%–40% of patients. Risk factors for the development of vasospasm include poor clinical status, thick blood on CT scan (>1 mm thick), hypertension, volume depletion, low cardiac output, smoking, fever, and vasospasm on initial angiography (higher risk of subsequent vasospasm).
The “gold standard” for diagnosis of cerebral vasospasm is cerebral angiography. Transcranial Doppler (TCD) ultrasound can be used at the bedside to diagnose vasospasm. Although changes in mean flow velocities of the middle cerebral artery of >200 cm/second are highly suggestive of cerebral vasospasm, other vascular territories are unreliable. TCD scans can be performed daily, and elevated velocities on TCD scans can precede symptoms by 2 days. If TCD suggests vasospasm, further investigation (e.g., CTA, MRA, cerebral angiography) should be performed to confirm the diagnosis.
Most patients with SAH are treated prophylactically to prevent vasospasm. At the present time, nimodipine is the only medication shown in clinical trials to improve outcomes. Nimodipine is usually given for 2–3 weeks, and the dose is based on blood pressure. A suggested protocol is 60 mg every 4 hours for systolic blood pressure (SBP) >140 mm Hg, 30 mg for SBP 120–140 mm Hg, and no drug for SBP <120 mm Hg. Normovolemia should be the goal in patients with SAH.
The treatment for patients who develop symptomatic vasospasm includes “triple H” therapy and direct arterial calcium channel blocker injection by cerebral angiography. “Triple H” therapy includes hypertension, hydration, and hemodilution. The goal is to increase the SBP 20–30 mm Hg above baseline but not >220 mm Hg. This goal can be accomplished with pressors, fluids, or both. Hemodilution is generally achieved by administering crystalloids to dilute the hematocrit, which also accomplishes hydration. If possible, repeat angiography should be performed to facilitate intraarterial injection of calcium channel blockers to relax the cerebral artery.
Hydrocephalus and elevated ICP can occur. Hydrocephalus is treated with an external ventricular device to divert CSF flow, which also treats increased ICP. Other management strategies for elevated ICP are hypertonic saline and mannitol to decrease brain swelling.
Seizures are a possible complication of SAH, and most patients are placed on prophylactic anticonvulsant therapy for the first 7 days. If the patient has a seizure or the aneurysm is not treated, anticonvulsant therapy is warranted for a longer period.
Hyponatremia usually occurs secondary to cerebral salt wasting syndrome and is not due to syndrome of inappropriate antidiuretic hormone (SIADH). Cerebral salt wasting is characterized by hyponatremia and dehydration with excessive urine output. Treatment entails correcting the hyponatremia with salt tablets or hypertonic saline and maintaining balanced fluid and urine outputs. It is important to rule out SIADH because treatment for SIADH (which is fluid restriction) should be avoided as it can worsen the patient’s volume status and increase risk of vasospasm from dehydration.
4
How is increased intracranial pressure diagnosed and treated?
The cranial vault comprises brain (80%), blood (10%), and CSF (10%). Because the volume in the cranial vault is fixed (noncompliant system), a small change in volume results in a large change in ICP. Some CSF and venous blood can shift extracranially to maintain ICP within normal limits (5–13 mm Hg). However, there is limited capacity for this compensatory mechanism ( Figure 91-1 ). Elevated ICP is a medical and surgical emergency. Irreversible brain injury or death can result if immediate action is not taken.

Increased ICP occurs secondary to space-occupying lesions, increased CSF (i.e., hydrocephalus), increased cerebral blood volume, and cerebral edema ( Box 91-2 ). In the setting of intact cerebral autoregulation, increases in cerebral blood flow do not usually result in increased cerebral blood volume. However, vascular abnormalities that disrupt cerebral autoregulation can lead to increased cerebral blood flow. Sinus thrombosis can lead to outflow obstruction of venous blood flow, causing elevated ICP. Cerebral edema leads to elevated ICP by four different mechanisms: vasogenic (vessel damage owing to mass, infection, or contusion), cytotoxic (ischemia, cell membrane failure), hydrostatic (increased transmural pressure secondary to hydrocephalus), and hypoosmolarity.
Space-occupying lesions
- •
Tumor
- •
Hematoma
- •
Air
- •
Abscess
- •
Foreign body
Cerebrospinal fluid accumulation
Obstructive
- •
Cerebral aqueduct stenosis
- •
Tumor
- •
Congenital malformation (e.g., Chiari, Dandy-Walker)
Nonobstructive (communicative)
- •
Impaired cerebrospinal fluid resorption
- •
Hemorrhage (e.g., SAH, IVH)
- •
Infection (e.g., meningitis, ventriculitis)
- •
Scarring or fibrosis (from infection or inflammatory process)
- •
Increased production
- •
Choroid plexus papilloma
Increased cerebral blood volume
- •
Loss of cerebral autoregulation
- •
Sinus thrombosis
Cerebral edema
- •
Vasogenic
- •
Cytotoxic
- •
Hydrostatic
- •
Hypoosmolarity
IVH, Intraventricular hemorrhage. SAH, subarachnoid hemorrhage.
Increased ICP can lead to decreased cerebral perfusion pressure (CPP). CPP is calculated by subtracting the ICP from the mean arterial pressure (MAP). If central venous pressure (CVP) is greater than ICP, the CPP is calculated by MAP − CVP. Decreases in CPP can result in reductions in cerebral blood flow leading to ischemia ( Figure 91-2 ). Neuronal injury can also result from direct brain tissue compression and herniation in the setting of increased ICP.

The generally acceptable threshold for ICP is <20–25 mm Hg. ICP >20–25 mm Hg mandates aggressive clinical management. Patients may intermittently have plateau waves of increased ICP >60–80 mm Hg, which are not associated with worse outcomes unless they last >30 minutes.
Signs of elevated ICP can be observed on neurologic examination. Mental status changes could range from mild confusion to coma. Papilledema may indicate elevated ICP; however, it can be observed in patients with normal ICP. Uncal herniation produces an ipsilateral fixed and dilated pupil (cranial nerve [CN] III palsy). CN VI palsy, inability to turn the eye outward, may be present. Posturing, either decorticate (flexor) or decerebrate (extensor), is a nonspecific sign of elevated ICP. Decorticate posturing occurs when the injury is between the cortex and the red nucleus. Decerebrate posturing occurs when the injury is below the red nucleus. Posturing provides only general localizations and not absolute anatomic diagnoses. Abnormal respiratory patterns, such as Cheyne-Stokes respiration, central neurogenic hyperventilation, sustained inspiratory effort, clustered breathing, and ataxic breathing, can also be observed in patients with elevated ICP. The only definitive way to diagnose elevated ICP is to measure it directly.
The concept of CPP-directed treatment of increased ICP was introduced >30 years ago. There has been a small improvement in outcome statistics, but mortality still ranges from 20%–30%. When increased ICP is diagnosed, emergent treatment must be initiated. Surgical consultation should be sought immediately for possible evacuation of blood or excess fluid or ventricular drainage of CSF. The goals of therapy are to reduce ICP and attain a CPP of 60–100 mm Hg. CPP is optimized by increasing MAP and reducing ICP ( Box 91-3 ). If ICP remains elevated, osmotherapy with either mannitol or hypertonic saline should be considered. Mannitol and hypertonic saline are the mainstays of treatment of neurologic emergencies related to cerebral edema and elevated ICP. There are no data showing superiority of either mannitol or hypertonic saline. However, mannitol should be administered with caution to patients with a low ejection fraction or renal failure.
Immediate surgical consultation
Increase MAP
Vasopressors
Isotonic fluids
Decrease intracranial pressure
Elevate head of bed 30–45 degrees
Avoid administration of free water
Maintain mildly elevated plasma sodium levels (150–155 mEq/L)
Avoid increases in cerebral metabolic rate
Avoid hyperthermia
Avoid shivering
Prevent seizures
Control pain
Continue normocarbia (pCO 2 37–40 mm Hg)
May institute short-term hyperventilation (pCO 2 30-32 mm Hg)
If intracranial pressure remains elevated
Osmotherapy
Mannitol 20%
0.5–1 g/kg bolus followed by 0.5 g/kg every 4–6 hours
Goal: 300–320 mOsm/L in serum
Caution in patients with low ejection fractions or renal failure
Hypertonic saline (3% NS)
1 mg/kg/hour
Goal: Sodium 150–155 mEq/L
NS, Normal saline; pCO 2 , carbon dioxide tension.
More recently, small volumes of high-osmolarity solutions (23.4% saline) have been used to treat intracranial hypertension crises. The theory behind the use of high-osmolarity solutions is that they create a driving force to mobilize water from the interstitial and intracellular compartments of the brain into the intravascular compartment. Reduction in brain water can lead to a >50% reduction in ICP in the acute setting allowing additional time to arrange for other diagnostic or therapeutic interventions. Advantages of high-osmolarity saline include low cost; amenable to repeat administrations; low total volume; and freedom from allergenicity, which mannitol is not. Treatment with high-osmolarity saline does have some risks, although complications are uncommon. Recognized complications include hyperosmolarity, central pontine myelinolysis, subdural hematomas, congestive heart failure, acid-base derangements, and coagulopathies. This treatment still requires further investigation to determine the optimal dose as well as the safest and most effective mode of administration.
ICP monitoring is considered the standard of care in most clinical centers, although some studies have shown no change in mortality with ICP monitoring versus monitoring with imaging and clinical examinations only. Several techniques are available for ICP monitoring ( Table 91-3 ). The goal of all these monitoring devices is to provide early warning of impending brain hypoxia or ischemia as well as improving cerebral hemodynamics and oxygenation. Some noninvasive methods allow the medical team to look for additional signs of elevated ICP.
Monitoring Technique | Advantages | Disadvantages |
---|---|---|
Ventricular catheter | “Gold standard” Allows for CSF drainage | Invasive Risk of infection Risk of bleeding Must be calibrated |
Intraparenchymal catheter | Intraparenchymal or subdural placement Easier to place than ventricular catheter Lower infection rate | Drift of measurements over time Measures localized pressure only where the sensor is placed |
NIRS | Measures cerebral oximetry Detects cerebral ischemia Noninvasive | Lack of standardization among commercial NIRS cerebral oximeters Risk of measuring extracranial blood oxygen levels |
Optic nerve sheath diameter | Noninvasive Optic sheath is a continuation of dura Increase in optic sheath diameter correlates with increased ICP | Emerging technology Change detected in millimeters |
Transcranial Doppler | Noninvasive | Requires training Assesses regional blood flow Higher failure rate when difficult to obtain windows for Doppler |

Full access? Get Clinical Tree
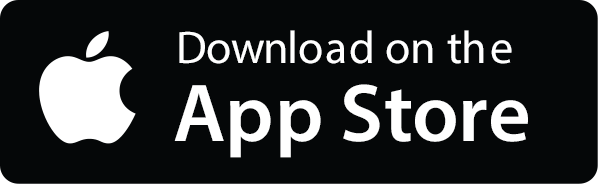
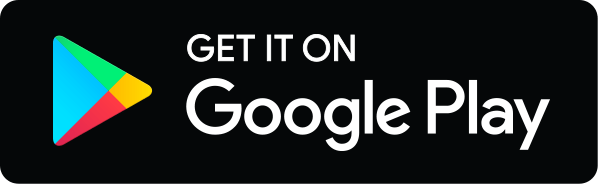
