Peter von Homeyer
I. Cardiac Anatomy
The normal heart is about the size of an adult fist, weighs approximately 300 g, and has a trapezoid shape with its apex oriented leftward and anterior in the chest (Fig. 3-1) (1). The anterior thoracic surface projections of the heart and great vessels, relative to the bony ribs, sternum, and xiphoid process, are shown in Figure 3-2. As the central blood pump of the human body, it contracts about 100,000 times per day and propels blood into the pulmonary and systemic circulations. The right and the left chambers are anatomically separated by septa. Only in the fetal circulation or in the setting of certain pathologies (e.g., atrial septal defect) is there direct communication of blood flow between the right and left sides. On each side, blood first flows from veins into a thin-walled atrium. It then flows through an atrioventricular (AV) valve into a muscular ventricle, and finally through a semilunar valve into the great arteries—the aorta on the left and the pulmonary artery (PA) on the right (Fig. 3-3).
Figure 3-1 Key anatomic features of heart from the anterior view (A) and posterior-inferior view (B). (From Moore KL, Agur AMR, Dalley II AF. Thorax. In: Moore KL, Agur AMR, Dalley II AF. Clinically Oriented Anatomy, 7th ed. Philadelphia: Lippincott Williams & Wilkins, 2013:131–149, with permission.)
Figure 3-2 Anterior surface projections of the heart and great vessels relative to the lungs and ribs. Note the close relation of the lung apices to the internal jugular and subclavian veins (relevant to placement of central venous catheters) and the bare area of the pericardium that can be accessed for pericardiocentesis with needle placement under and to the left of the xiphoid process of the sternum. (From Moore KL, Agur AMR, Dalley II AF. Thorax. In: Moore KL, Agur AMR, Dalley II AF. Clinically Oriented Anatomy, 7th ed. Philadelphia: Lippincott Williams & Wilkins, 2013:131–149, with permission.)
Figure 3-3 The course of normal blood flow from the great venous vessels through the right and left heart chambers to the systemic aorta. (From Moore KL, Agur AMR, Dalley II AF. Thorax. In: Moore KL, Agur AMR, Dalley II AF. Clinically Oriented Anatomy, 7th ed. Philadelphia: Lippincott Williams & Wilkins, 2013:131–149, with permission.)
The heart wall has three layers: the thin, inner, endocardium; the thick, middle myocardium; and the outer epicardium (i.e., the visceral pericardium). The myocardium is anchored to the cardiac fibrous skeleton, a system of dense collagen forming two rings and connective trigones that separate the atria and ventricles to prevent uncontrolled conduction of electrical impulses and also serve as anchors for the AV valves.
The right atrium (RA), superior vena cava (SVC), and inferior vena cava (IVC) form the right lateral border of the heart. Venous return from the heart itself enters the RA through the coronary sinus (CS), which collects blood from the major cardiac veins. The internal wall of the RA includes the right-sided wall of the interatrial septum (IAS). The center of the IAS features the oval fossa, a small groove that is a remnant of the foramen ovale. It allows oxygenated blood to cross from right to left in the fetal circulation. It usually closes after birth, but remains patent in 25% to 30% of the population.
The right ventricle (RV) forms most of the anterior surface of the heart, some of its inferior surface, and has about a sixth of the muscle mass of the left ventricle (LV). The muscular interventricular septum (IVS) functions as a contractile wall for both the RV and the LV.
The tricuspid valve (TV) has three distinct leaflets (anterior, septal, and posterior) that are attached to tendinous cords. It connects to papillary muscles that tighten to draw the valve cusp edges together in ventricular systole and prevent regurgitant flow through this AV valve. The RV wall is heavily trabeculated, with one prominent trabecula (moderator band) connecting the IVS with the anterior RV wall. It houses the right branch of the AV conduction bundle (see below).
The pulmonic valve (PV) is a semilunar valve with three defined cusps (anterior, left, right) that are pushed toward the wall of the right ventricular outflow tract (RVOT) with ventricular contraction in systole. After relaxation in diastole, the cusps close like an umbrella to prevent regurgitation. The main PA quickly bifurcates into right and left branches that lead deoxygenated blood to the pulmonary circulation for subsequent gas exchange.
Oxygenated blood returning from the lungs enters the left atrium (LA) through four pulmonary veins, normally two originating from each lung. The LA forms the majority of the base of the heart, with the small LA appendage as part of its anterolateral wall. The IAS has a small semilunar indentation representing the left-sided aspect of the oval fossa.
The LV forms the apex of the heart, as well as most of its left (lateral) and diaphragmatic (inferior) surfaces, and its normal maximal wall thickness is 10 mm (compared with 3 mm in the RV). The IVS is concave to the highly trabeculated LV wall, resulting in an almost circular LV chamber on anatomic cross-section.
Unlike the right-sided TV, the left-sided AV mitral valve (MV) is bicuspid, with anterior and posterior leaflets that are connected to anterolateral and posteromedial papillary muscles by tendinous cords similar to those of the TV. Both MV leaflets receive cords from papillary muscles that keep this valve shut in the setting of high intraventricular pressure during systole.
The inflow and outflow tracts of the LV lie almost parallel to each other, with the anterior leaflet of the mitral valve forming a natural separation between these two structures. The left ventricular outflow tract (LVOT) is more smooth walled and of round or oval shape, and it contains the aortic valve through which blood enters the systemic circulation. This valve has three distinct cusps named after the presence or absence of coronary artery ostia originating from the sinuses of Valsalva just above the valve: the left coronary, right coronary, and noncoronary cusps (Fig. 3-4).
Coronary artery anatomy is termed either right dominant or left dominant depending on which main coronary artery feeds the posterior descending branch (in the interventricular groove) to the posterior-inferior surface of the heart. Right dominant circulation is most common, found in 70% of the population.
Figure 3-4 Relation between the aortic valve cusps and the coronary arteries. Like the pulmonary valve, the aortic valve has three semilunar cusps: right, posterior, and left. During systole, ejected blood forces the cusps apart. During diastole, the cusps close and coronary artery flow occurs. (From Moore KL, Agur AMR, Dalley II AF. Thorax. In: Moore KL, Agur AMR, Dalley II AF. Clinically Oriented Anatomy, 7th ed. Philadelphia: Lippincott Williams & Wilkins, 2013:131–149, with permission.)
The coronary vasculature consists of the coronary arteries (Fig. 3-5) and the CS (described earlier). The arteries carry blood to most of the myocardium except the subendocardial layers, which receive oxygen directly via diffusion from blood inside the cardiac chambers. The left coronary artery (LCA) and the right coronary artery (RCA) arise from the respective sinuses in the proximal aorta. The RCA travels to the right of the PA in the AV groove and sends branches to the sinoatrial (SA) node (inside the RA wall) and to the right border of the heart. The RCA continues posteriorly in the AV groove and sends a branch to the AV node of the conducting system before entering the posterior interventricular groove. When the RCA continues down that groove to form the posterior interventricular branch, this is termed right dominant circulation (~70% of individuals). The LCA travels between the PA and the LA appendage and splits early in its course into the left anterior descending (LAD) artery and the circumflex branch. The LAD continues in the anterior interventricular groove all the way to the LV apex and around the inferior aspect of the heart where it often forms anastomoses with the branches of the posterior interventricular branch. The LAD also sends many septal branches to the IVS throughout its course, as well as prominent diagonal branch to the lateral wall. When the circumflex branch gives rise to the posterior interventricular branch, this is termed left dominant circulation (~30% of individuals). Such variable coronary artery anatomy is important when trying to understand the relation between coronary artery disease and regional dysfunction of ischemic myocardium.
Figure 3-5 Coronary artery anatomy for the typical right dominant pattern (see text for details) is shown from the anterior view (A) and the posterior view (B). (From Moore KL, Agur AMR, Dalley II AF. Thorax. In: Moore KL, Agur AMR, Dalley II AF. Clinically Oriented Anatomy, 7th ed. Philadelphia: Lippincott Williams & Wilkins, 2013:131–149, with permission.)
Normal electrical conduction is initiated by an electrical impulse generated in the SA node, a locus of specialized cardiac cells in the RA wall that have no contractile function. As the pacemaker center of the heart, the SA node autonomically generates an impulse at about 60 to 80 beats per minute. From the SA node, bundles of cells lead to the AV node located above the right fibrous trigone of the heart at the AV border. From the AV node, the impulse is conducted in the AV bundle that pierces the fibrous skeleton of the heart and splits just above the muscular IVS into right and left bundles. The right bundle continues toward the apex of the heart and then splits into smaller subendocardial RV branches. The left bundle splits close to its origin into a left anterior and a left posterior branch, which then further split into subendocardial LV branches near the apex of the heart (Fig. 3-6).
VIDEO 3-1
Pericardium
The pericardium is a double-layered sac around the heart. The visceral serous layer (epicardium) covers most of the heart’s surface. It extends to and reflects at the proximal portion of the great vessels and turns into the parietal pericardial sac. Between the two layers, a small amount of fluid is considered physiologic. The pericardium protects and restrains the heart, reduces friction associated with its constant movement within the mediastinum, and separates the heart and origin of the great vessels from other structures inside the mediastinum. Abnormal pericardial fluid collections (e.g., pericardial tamponade) can be accessed and withdrawn as described in Figure 3-2.
VIDEO 3-2
Pericardial Effusion
Figure 3-6 Impulses initiated at the sinoatrial (SA) node are propagated through the atrial musculature to the atrioventricular (AV) node, followed by conduction through the AV bundle and its right and left branches in the intraventricular septum (IVS) to the myocardium. (From Moore KL, Agur AMR, Dalley II AF. Thorax. In: Moore KL, Agur AMR, Dalley II AF. Clinically Oriented Anatomy, 7th ed. Philadelphia: Lippincott Williams & Wilkins, 2013:131–149, with permission.)
VIDEO 3-3
Heart Papillary Muscles
II. The Cardiac Cycle
The cardiac cycle consists of an orchestrated sequence of spontaneous electrical and contractile events occurring simultaneously in both the right and left sides of the heart. When combined with the flow-directing influence of the four unidirectional heart valves, a sequential rise and fall of fluid pressures within each of the four heart chambers results in a predictable pattern of chamber volumes and pressures that produces forward cardiac output and accompanying heart sounds associated with valve closure (2). These synchronized electrical and mechanical events are depicted under normal anatomic and physiologic conditions in Figure 3-7. Anatomic or physiologic abnormalities in any of these components can alter events of the cardiac cycle and ultimately impact cardiac performance.
VIDEO 3-4
Animated Cardiac Cycle
Figure 3-7 Mechanical and electrical events of the cardiac cycle also showing the left ventricular (LV) volume curve and the heart sounds. Note the LV isovolumic contraction period (ICP) and the relaxation period (IRP) during which there is no change in LV volume because the aortic and mitral valves are closed. The LV decreases in volume as it ejects its contents into the aorta. During the first third of systolic ejection (the rapid ejection period), the curve of emptying is steep. ECG, electrocardiogram. (From Pagel PS, Kampine JP, Stowe DF. Cardiac anatomy and physiology. In: Barash PG, Cullen BF, Stoelting RK, et al. Handbook of Clinical Anesthesia, 7th ed. Philadelphia: Lippincott Williams & Wilkins, 2013:239–262, with permission.)
Focusing on the left heart, depolarization of the LV associated with the QRS complex of the electrocardiogram (ECG) initiates LV contraction and begins the period of systole with closure of the mitral valve contributing to the first heart sound (S1). During early systole, both the mitral and aortic valves are closed; the mitral valve due to the positive LV → LA pressure gradient and papillary muscle contraction, and the aortic valve due to the positive aortic root → LV pressure gradient. Because LV volume is fixed during early systole, LV contraction results in a brief, yet rapid isovolumic elevation in LV pressure. The maximum rate of rise in LV pressure (+dP/dt) occurs during this brief isovolumic contraction period and is commonly used as the index of LV contractility (as discussed later).
Once the rapidly rising LV pressure exceeds aortic root pressure, the aortic valve passively opens and pulsatile aortic flow begins. Both the LV and aortic pressures continue to rise, then quickly peak and fall during the remainder of systole as ventricular contraction ceases and the LV repolarizes. The stroke volume ejected during systole is approximately two-thirds of the end-diastolic LV volume.
Systole ends when the slowly declining aortic root pressure exceeds the more rapidly falling LV pressure, resulting in passive closure of the aortic valve and its contribution to the second heart sound (S2). As diastole begins, both the mitral and aortic valves are briefly closed. During this short-lived, isovolumic relaxation period, LV pressure rapidly falls, while LA pressure slowly rises due to pulmonary inflow. When LA pressure exceeds LV pressure, the MV passively opens and diastolic filling of the LV begins. Diastole consists of four phases—isovolumic relaxation, early diastolic filling, diastasis, and atrial systole (LA contraction)—until the cardiac cycle is repeated with LV depolarization and contraction.
Similar parallel events occur in the right heart during the cardiac cycle with corresponding chamber volumes (i.e., right and left ventricular stroke volumes are equal under normal anatomic conditions) and tricuspid/pulmonic valve movements mirroring the mitral/aortic movements. As a result of peristaltic inflow, lesser cardiac muscle mass, and lesser contractile strength of the RV, there is no isovolumic contraction period in the RV. The RV and PA pressures are significantly lower than corresponding left-sided pressures. Right-sided systolic ejection time may exceed the left-sided time, resulting in later closure of the pulmonic valve (compared with the aortic valve) and a split S2. During spontaneous inspiration, venous return is increased to the right ventricle and decreased to the left ventricle, resulting in prolongation of the splitting of S2, referred to as physiologic splitting.
With spontaneous inspiration, venous return to the right ventricle is increased, resulting in prolonged ejection time compared with the left ventricle. This causes the pulmonic valve to close later than the aortic valve, producing respiration-induced variation in splitting of S2 (physiologic splitting).
III. Control of Heart Rate
Heart rate is determined by the constantly and often instantaneously changing balance between multiple intrinsic and extrinsic factors. Key intrinsic factors include autonomic efferent innervation (both sympathetic nervous system [SNS] and parasympathetic nervous system [PNS]) (see Chapter 4), neural reflex mechanisms, humoral influences, and cardiac rhythm. Extrinsic factors include direct- and indirect-acting pharmaceutical and recreational drugs, fear, hyperthermia, and others that affect heart rate through modulation of intrinsic factors.
Efferent autonomic tone to the heart is initiated in the anterior (PNS) and posterior (SNS) hypothalamus and is modulated by the cardiac acceleration and cardiac slowing centers in the medulla prior to peripheral distribution. Sympathetic preganglionic fibers arising from T1 to T4 spinal levels enter the nearby paravertebral sympathetic chain, inferior cervical (stellate) ganglion, and middle cervical ganglion. They synapse with postganglionic SNS neurons that directly innervate the SA node, AV node, and myocardium via β1-adrenergic norepinephrine receptors. PNS preganglionic fibers to the heart arise from the brainstem and are carried in the vagus nerve. Both the right and left vagus nerves exit the jugular foramena, traverse the neck within the carotid sheaths posterior to the carotid arteries. They course directly to the heart where they synapse with short postganglionic PNS neurons that moderate the SA and AV nodes via muscarinic acetylcholine receptors. The opposing effects of the SNS (tachycardia) and PNS (bradycardia) on the SA node normally favor vagal inhibition. As a result of this vagal predominance, load-induced increases in heart rate are first achieved by release of PNS tone and thereafter by SNS activation. Several neural reflex mechanisms can also affect heart rate, including the baroreceptor response, atrial distention response (Bainbridge reflex), carotid chemoreceptor reflex, Cushing reflex, and oculocardiac reflex (Table 3-1).
Table 3-1 Cardiac Reflexes that Affect Heart Rate
Humoral factors (e.g., circulating catecholamines) also influence heart rate independent of the SNS and PNS. For example, the denervated heart following heart transplantation responds to exercise load with tachycardia owing to increased circulating catecholamine levels. Myocardial β1-adrenergic receptors can also be activated and heart rate is increased by direct pharmacologic agonists (isoproterenol, epinephrine), agents that indirectly cause release of endogenous catecholamines (ephedrine), or drugs that impair catecholamine metabolism or reuptake (cocaine).
IV. Coronary Physiology
Resting coronary blood flow is approximately 250 mL/min (~5% of total cardiac output) and can be increased up to fivefold during strenuous physical exercise. Coronary blood flow is influenced by physical, neural, and metabolic factors. The primary physical factor is coronary perfusion pressure—the difference between aortic pressure and either LV pressure (left coronary artery) or RV pressure (right coronary artery). Extravascular coronary artery compression (due to contracting myocardium), heart rate (altering the duration of diastole), vessel length, and blood viscosity also impact coronary perfusion. The primary neural factor is SNS tone to the heart, which increases coronary blood flow when increased aortic pressure outweighs reduced coronary flow associated with stronger myocardial contraction and shortened diastolic filling time (tachycardia). Active vasodilation of coronary arteries is limited because vagal stimulation has no apparent effect on vessel caliber and, unlike skeletal muscle vasculature, sympathetic cholinergic innervation is not present in coronary arteries. However, β2-adrenergic receptor–mediated vasodilation can occur in small coronary arterioles and accounts for ~25% of coronary vasodilation observed during exercise-induced hyperemia. Lastly, increased myocardial metabolism is associated with the bulk of coronary vasodilation through the action of yet-to-be-defined local metabolic factors.
Because the subendocardium is exposed to higher pressures during systole than the subepicardial layer, the former is more susceptible to ischemia, particularly in settings of coronary stenosis, ventricular hypertrophy, or tachycardia.
Coronary blood flow varies with the cardiac cycle, and is determined by the difference between aortic pressure and tissue (wall) pressure. LCA flow is highly variable—it peaks during early diastole when perfusion pressure is highest and approaches zero in early systole when LV contraction (and coronary compression) is greatest. In contrast, RCA flow is more constant throughout the cardiac cycle and peaks during systole due to the lesser muscle mass and contraction of the RV. Because the subendocardium is exposed to higher pressures during systole than the subepicardial layer, the former is more susceptible to ischemia, particularly in settings of coronary stenosis, ventricular hypertrophy, or tachycardia. However, subendocardial ischemia is partially offset by enhanced capillary anastomoses and local metabolic vasodilation in this layer.
VIDEO 3-5
Coronary Perfusion
The heart has the highest oxygen extraction ratio of any organ (~70%); as a result, under normal conditions the venous oxygen saturation of blood in the coronary sinus (~30%) is lower than that in the right atrium (~70%). Myocardial oxygen consumption is determined by heart rate, myocardial contractility, and ventricular wall stress (including preload and afterload), with the major determinants being the heart rate and the magnitude of LV pressure developed during the isovolumic contraction period. Because of this high extraction ratio, increased myocardial oxygen demand can only be met through increased coronary blood flow. Thus, the dominant controller of coronary blood flow is myocardial oxygen consumption. The coronary circulation is ideally constructed for this purpose, as its myocardial capillary density is approximately eight times greater than that of skeletal muscle (approximately one capillary for each cardiac muscle fiber). When myocardial oxygen supply is unable to meet increases in myocardial oxygen demand (e.g., coronary artery stenosis), myocardial ischemia occurs. Ischemia is first clinically manifested by increased LV end-diastolic volume and decreased LV compliance and can progress to wall motion abnormalities, decreased ejection fraction, ECG abnormalities (ST-segment changes), congestive heart failure (CHF), and ultimately cardiogenic shock.
VIDEO 3-6
Myocardial Oxygen Supply-Demand
V. The Pressure-Volume Diagram
The mechanical events in the LV cardiac cycle depicted in Figure 3-7 can also be presented graphically as the LV pressure-volume (P-V) diagram, shown in Figure 3-8

Full access? Get Clinical Tree
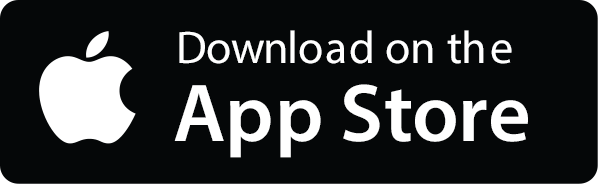
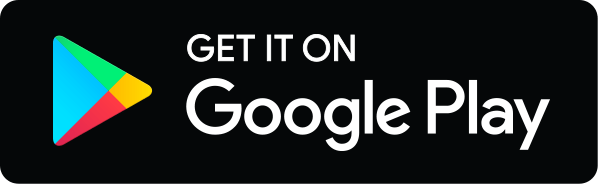