Cardiotoxins
BACKGROUND
Numerous therapeutic options exist for medical management of dysrhythmia, heart failure, and hypertension. In 2011, more than 42.4 million patients received therapy for hypertension alone, and prescriptions for ACE inhibitors, beta-adrenergic antagonists (BAAs), calcium channel antagonists/“blockers” (CCBs), and angiotensin II inhibitors (alone or in combination) exceeded 509 million.1 Cardiotoxicity may occur in this setting due to intentional overdose, unintentional therapeutic misadventure, or interaction with other medications.
Cardiotoxicity may also result from noncardiovascular medications with associated sodium or potassium channel antagonism or muscarinic effects; from a variety of systemic pharmaceuticals; and from natural, occupational, or environmental exposures. In the pediatric population, there is risk of unintentional exposure to caregivers’ medication or when visiting other households.2 More than 90,000 incidents involving cardiovascular drugs are reported to poison control centers each year—5.7% and 2.2% of adult and pediatric exposure calls, respectively—accounting for a disproportionate 11% of fatalities.3 This chapter offers a guide to the diagnostic evaluation and treatment of the cardiotoxins most commonly encountered in the emergency department (ED), namely CCBs, BAAs, cardiac glycosides, and renin–angiotensin system antagonists (RASAs).
HISTORY AND PHYSICAL EXAM
The initial presentation of the cardiotoxic patient may range from asymptomatic to critically ill. Although not always available or reliable, information regarding details of the exposure (agent, intent, dose, formulation, and coingestants), as well as patient-specific factors (age, comorbidities, unique susceptibilities, etc.), should be sought. An appropriate history will consider pertinent cardiovascular symptoms (chest pain, dyspnea, palpitations, etc.) and their onset, as well as any symptoms attributable to other relevant organ systems (central nervous system [CNS], pulmonary, etc.), and more insidious complaints (weakness, nausea, anorexia, fatigue, etc.).
Physical exam should prioritize repeated assessment of vital signs, particularly heart and respiratory rate, blood pressure, and pulse oximetry. The CNS should be assessed for direct or indirect signs of poisoning and/or perfusion abnormalities (agitation, delirium, depression, coma) and seizures (e.g., from bupropion, local anesthetics, methylxanthines, sedative–hypnotic withdrawal, or sympathomimetics). Of note, CCB-poisoned patients may preserve their mental status despite severe hypotension and bradycardia. Rigorous cardiac, pulmonary, vascular, and organ system examinations should evaluate perfusion, failure, and potential reserve. Special attention should be paid to identifying specific patterns of cardiovascular and associated systemic anomalies suggestive of a particular toxidrome, including cholinergic (bradycardia with bronchorrhea, bronchospasm, diaphoresis, urination, miosis, lacrimation, emesis), antimuscarinic (tachycardia, tachypnea, mydriasis, flushed skin, urinary retention, delirium), and sympathomimetic (tachycardia, hypertension, tachypnea, hyperthermia, mydriasis, psychomotor agitation). In addition to a focused physical exam, bedside glucometry (the “sixth vital sign”) should be rapidly obtained and addressed. Hyperglycemia may suggest CCB exposure and severity, while hypoglycemia may be seen with BAAs.4
DIFFERENTIAL DIAGNOSIS
The number of substances capable of exerting cardiotoxic effects is myriad. Cardiotoxicity is a well-described attribute of abused substances (ethanol, nicotine, etc.), biologicals (aconitine, colchine, ricin, venoms, veratrine, etc.), chemotherapeutics (alkylators, anthracyclines, antimetabolites, monoclonal antibodies, taxanes, vinca alkaloids, etc.), environmental exposures (carbon monoxide, fluorocarbons, particulate air pollution, etc.), metals (arsenic, cadmium, cobalt, lead, etc.), nutritional toxins (thiaminases), and numerous other agents. Toxins that produce acid–base disturbances, autonomic nervous system dysfunction, electrolyte derangement, hematologic dyscrasias, or hypoxia (hypoxemic, histotoxic, or other) may secondarily compromise cardiovascular function. Cardiovascular instability may also accompany toxicologic-associated hyperthermias (anticholinergic crisis, malignant hyperthermia, neuroleptic malignant syndrome, mitochondrial uncoupling, sedative–hypnotic withdrawal, serotonin syndrome, sympathomimetic toxicity, thyroid toxicity, etc.).
Pharmaceuticals that produce hypotension and/or bradycardia include antidysrhythmics, cardiac glycosides (digoxin), BAAs, CCBs, imidazoline derivatives (clonidine, dexmedetomidine, guanfacine, guanabenz, oxymetazoline, and tetrahydrozoline), nitrates, and RASAs. Although uncommon, anticonvulsants (carbamazepine, phenytoin, etc.), barbiturates, and opioids may also cause bradycardia and hypotension. Cardiotoxicity may also arise from medications with sodium channel antagonism (carbamazepine, cocaine, cyclic antidepressants [CAs], local anesthetics, diphenhydramine, lamotrigine, venlafaxine, and others with Vaughan-Williams class IA and IC effects), potassium channel antagonism (antipsychotics, methadone, selective serotonin reuptake inhibitors, etc.), or direct or indirect cholinergic effects.
Sympathomimetics, including adrenergic reuptake inhibitors, amphetamines and their derivatives, cathenones and their analogs, cocaine, phencyclidine (PCP), and piperazines may produce tachycardia and/or hypertension. Methylxanthines (caffeine, theophylline, theobromine), cannabinomimetics, and monoamine oxidase inhibitors may present similarly. Substances with antimuscarinic properties may antagonize vagal effects on cardiac pacemakers, for example, antihistamines, CAs, and scopolamine. Vasodilators such as alpha-blockers, peripherally acting CCBs, and nitrates can result in reflex tachycardia as a compensatory response. Finally, withdrawal from sedative–hypnotics (barbiturates, benzodiazepines, ethanol, gamma hydroxybutyrate [GHB]), opioids, and antihypertensives (clonidine, BAAs, and others) can induce a presentation consistent with sympathomimetic toxicity.
DIAGNOSTIC EVALUATION
An electrocardiogram (ECG) should be rapidly obtained for all cardiotoxic patients. Agents that delay atrial electrical transmission (e.g., quinidine) may produce notched P waves. BAAs, cardiac glycosides, CCBs, cholinergics, and magnesium impair nodal conduction leading to variable blocks. Sodium channel antagonists, such as CAs, and others that compromise myocyte depolarization and disproportionately affect right ventricular depolarization, produce a classic ECG pattern of QRS widening and terminal rightward depolarization of the last 40 milliseconds of the QRS complex, giving rise to an R wave in lead AVR and/or an S wave in leads I and AVL. Hyperkalemia and hypermagnesemia may also cause widening of the QRS complex. Potassium channel antagonism may give rise to an increased QT/QTc interval, yielding a myocardial substrate vulnerable to afterdepolarizations, ventricular tachycardia, and ventricular fibrillation. Although not necessarily indicative of toxicity, digoxin and other cardiac glycosides cause repolarization abnormalities that appear as scooping of the ST segment, or “dig effect.” Premature ventricular contractions, representing myocardial irritability, are more ominous and merit consideration for digoxin-specific Fab fragment (DSFab) administration.
Bedside ultrasound is another valuable tool in the hemodynamic status assessment of the cardiotoxic patient.5 Negative inotrope toxicity may result in global myocardial dysfunction and impaired cardiac output, while a hyperdynamic heart may suggest adrenergic stimulation or represent a reflex to peripherally acting agents. Contemporaneous ultrasound assessment of volume status can also be performed.6
Laboratory testing should include serum electrolyte concentrations—particularly sodium, potassium, chloride, and calcium—to help guide repletion or interventions. In acute digoxin ingestions, prior to the availability of DSFab, elevations in serum potassium concentrations (a manifestation of sodium–potassium-ATPase inhibition) between 5 and 5.5 mEq/L were associated with 50% mortality; potassium concentrations above 5.5 mEq/L were associated with 100% mortality.7 BAA overdose may also elevate serum potassium.8 Assessment of renal function with urea nitrogen and creatinine may inform anticipated toxicity or duration of effect for renally cleared medications (e.g., atenolol, digoxin). Specific cardiotoxin concentrations are generally unavailable except for digoxin and theophylline, although evaluation of common coingestants (acetaminophen, salicylates, and ethanol) is often warranted.
MANAGEMENT GUIDELINES
Management of cardiotoxic patients requires vigilance on several fronts. Ensuring adequate oxygenation, ventilation, and intravascular volume expansion is essential. Serum glucose levels also require close observation; during periods of stress, myocardial metabolic substrate preference may shift from glucose to fatty acids, which can compromise contractile performance.9 Toxin-induced hemodynamic instability is not uncommon. Hypertension should be managed with short-acting agents such as nitroglycerine, nitroprusside, or phentolamine. Hypotension should be corrected with titratable, direct-acting inotropic or vasoactive agents, such as norepinephrine, phenylephrine, and epinephrine; these have been proved more effective than indirect agents like dopamine.10,11
Decontamination
If not already administered, more definitive antidotal therapy should be considered following hemodynamic stabilization. A risk–benefit assessment should guide the decision to employ adjuncts that alter toxicant pharmacokinetics (e.g., orogastric lavage, activated charcoal, whole-bowel irrigation, urinary alkalinization, extracorporeal removal), particularly in cases of significant gut burden, ongoing absorption, sustained release products, or antimuscarinic or opioid coingestants. For certain potentially severe ingestions, aggressive decontamination with gastric lavage within 1 hour,12 activated charcoal (1 g/kg),13 and whole-bowel irrigation (polyethylene glycol electrolyte lavage solution at 1 to 2 L/h until clear rectal effluent)14 may significantly reduce systemic absorption and alter the disease course.
Adjunct Therapies: Cardiac Pacing, IABP, and ECMO
While atropine and transcutaneous pacing may be employed in the initial management of toxic bradycardias, results are generally unsatisfying due to persistent negative inotropy. As transvenous pacing may actually worsen cardiac glycoside toxicity,15 this particular condition should be excluded or empirically treated with DSFab prior to any attempts at transvenous pacing. While insufficient data exist regarding the role of intra-aortic balloon pump (IABP) counterpulsation in cardiotoxicity, small series suggest that IABP may be used to support hemodynamics and vital organ perfusion in patients refractory to pharmacologic interventions while toxin metabolism occurs.16–18 Similarly, case studies have documented successful application of extracorporeal membrane oxygenation (ECMO) cardiopulmonary bypass to treat drug-induced cardiogenic shock and cardiac arrest refractory to traditional interventions.19
Specific Antidotes
Relative to the near-inexhaustible list of cardiotoxins, few specific antidotes exist; those that do rarely contain a specific FDA-approved toxicologic indication (e.g., only DSFab of the antidotes below). Given the ethical and practical difficulties in performing randomized or case-controlled trials in overdose victims, high-level evidence is often unavailable. Specific antidotal strategies represent a careful consideration of the medical literature, textbooks, expert opinion, and practice guidelines, although individual and institutional practices vary considerably.
Calcium
Based on anticipated digoxin-induced elevated intracellular Ca2+ concentrations and calcium-associated adverse events in digoxin-poisoned canines,20 calcium should be avoided in hyperkalemia treatment in digoxin toxicity (managed with DSFab). Intravenous calcium is indicated to overcome the direct or indirect antagonism of L-type calcium channels induced by CCB and BAA poisoning. Animal models demonstrate calcium salt benefit in CCB- and BAA-induced deficits in contractility, blood pressure, and cardiac output.21–23 Human case series and case reports generally support these findings, although conduction deficits and bradycardia may persist, and data for BAA toxicity are less robust.24,25 Calcium is provided intravenously as 10% calcium gluconate (4.3 mEq elemental calcium per 10 mL), or via a central vein as 10% calcium chloride (13.6 mEq elemental calcium per 10 mL). Both regimens liberate Ca2+ rapidly.26 A reasonable starting dose is 1 g (10 mL) of 10% calcium chloride or 3 g (30 mL) of 10% calcium gluconate, although some practitioners initiate higher doses.27,28 To sustain therapeutic response, redosing every 20–60 minutes may be required. Ongoing diligent monitoring of calcium, serum phosphate, and hydration status is necessary to mitigate the adverse consequences of hypercalcemia.
Digoxin-Specific Fab Fragments (DSFab)
DSFab is a safe and effective antidote for cardiac glycoside toxicity, and prior to its availability, digoxin toxicity carried a mortality rate exceeding 23% in patients requiring cardiac pacing.15,29,30 Indications for DSFab therapy include27
- Adult ingestions of ≥10 mg of digoxin (4 mg by a child)
- Serum digoxin concentrations ≥15 ng/mL, or concentrations ≥10 ng/mL beyond 6 hours after acute ingestion
- Shock or hemodynamic instability
- Progressive dysrhythmia, bradydysrhythmia refractory to atropine, or evidence of new ventricular ectopy (e.g., PVCs)
- Serum potassium ≥5.0 mEq/L in acute poisoning
- End-organ manifestations (e.g., altered mental status)
- Significant gastrointestinal symptoms or renal impairment in chronic poisoning
DSFab dosing is based either on the digoxin quantity ingested or on postdistributional serum digoxin concentrations:

The number of vials is rounded up and administered IV over 30 minutes in nonemergent scenarios. Empiric dosing of 10 to 20 vials in acute ingestion, or 3 to 6 vials (1 to 2 vials in children) in chronic ingestion, may be utilized when information is limited or in unstable patients. The rebound in free digoxin concentrations following therapy31 likely mitigates the exacerbation of heart failure, seen in approximately 3% of patients, due to the sudden lack of inotropic support provided by digoxin.29 Continued monitoring of electrolytes during DSFab therapy is important, as hypokalemia can develop.
Glucagon
Glucagon binds to G protein receptors, facilitating the production of cyclic adenosine monophosphate (cAMP) and enhancing cardiac inotropy and chronotropy. An increase in cAMP following administration of glucagon occurs independently of beta-adrenergic or calcium channel blockade. In volunteers, glucagon increased heart rate, cardiac index, and mean atrial pressure, but not systemic vascular resistance.32 Evaluation of glucagon efficacy in BAA or CCB overdose is primarily limited to case series.33 Dosing strategies typically employ an initial IV bolus of 50 μg/kg (maximum dose, 10 mg), repeatable after 3 to 5 minutes.28 A continuous infusion of the reversal dose is then provided per hour (e.g., 2 to 5 mg/h to a maximum of 10 mg/h). Nausea and vomiting should be anticipated and mitigated.
High-Dose Insulin Euglycemia (HIE)
Myocardial fuel for oxidative metabolism includes free fatty acids (FFA) at rest, glucose after meals, and lactate and FFA during exercise. During myocardial ischemia, an increase in catecholamine release results in stimulation of adipose release of FFA and decreased excretion of insulin from pancreatic beta cells. Experimental findings correlate elevation of plasma FFA with increased arrhythmias and mortality; insulin and glucose infusion has been found to mitigate this effect by decreasing the uptake of FFA by the myocardium and by enhancing glucose uptake.34 In dogs poisoned with BAAs or CCBs, HIE outperformed glucagon and epinephrine in mortality benefit and enhanced myocardial contractility.35,36 Higher insulin dosing (up to 10 units/kg/h) improved mortality and cardiac output in BAA-poisoned pigs compared with placebo or vasopressin plus epinephrine.37,38 In five patients with refractory, CCB-induced cardiogenic shock, an insulin bolus, followed by infusion at 0.1 to 1.0 units/kg/h, resulted in vasopressor sparing and survival in all.39 In a prospective observational study of seven patients with severe CCB toxicity given an insulin maintenance infusion of 0.5 to 2.0 units/kg/h, six survived; of these six, those that received an initial insulin bolus also showed improved hemodynamic parameters.40 A series of patients with cardiogenic shock from BAAs, CAs, and CCBs (alone or in combination) who were treated with HIE (up to 16 units/kg/h) showed survival in 92%, with sufficient hemodynamic improvement to permit vasopressor weaning.41
An initial insulin bolus of 0.5 to 1 unit/kg IV with dextrose supplementation as needed is typically followed by an insulin infusion of 1 unit/kg/h; maintenance of euglycemia with a 5% dextrose solution; careful potassium supplementation; and fingerstick glucose measurement every 30 minutes.40 Titration up to 10 units/kg/h has been described in clinical practice.42 Insulin’s inotropic effect is delayed (approximately 15–45 minutes); therefore, early initiation and concurrent inotrope/vasopressor therapy, with subsequent weaning as tolerated, are recommended. Emergency Department initiation of HIE should be followed by prompt patient transfer to an intensive care setting for rigorous and potentially protracted glucose administration and monitoring.39
Intravenous Lipid Emulsion
Intravenous lipid emulsion (ILE) (typically a 20% solution) has emerged as a novel therapy for toxic exposures. ILE’s mechanism of action is uncertain, and there are several competing theories to explain its efficacy. These include: creation of an ILE “lipid sink” or “lipid conduit” that isolates xenobiotics (nonnative chemical substances) within the plasma, thereby establishing a gradient that promotes diffusion from target organs and/or enhances elimination or redistribution;43,44 alteration of myocardial metabolism resulting in increased cardiac FFA metabolism to ATP; or activation of ion channels responsible for cardiac contractility. In vitro models predict ILE efficacy in drugs with more positive partition coefficients (logP) and greater volumes of distribution,45 although the distribution coefficient (logD, which accounts for ionization, particularly at physiologic pH) is a better descriptor of lipophilicity. Animal studies of ILE demonstrate mortality benefits in rats and dogs exposed to lethal doses of bupivacaine,43,46 verapamil,47 and selected other cardiovascular toxins. ILE rescue was first clinically used successfully in a case of seizures and refractory cardiac arrest due to intravenous local anesthetic exposure.48 Other adult and pediatric case reports suggest benefit in cases of cardiovascular toxicity due to antipsychotics, BAAs, bupropion, CAs, CCBs, cocaine, and local anesthetics.49–52
Experts advocate ILE use in bupivacaine toxicity, including in the cases associated with seizures and hemodynamic instability. An ILE bolus of 1.5 mL/kg IV over 1 minute, repeated every 3 to 5 minutes in persistent cardiovascular collapse, is followed by an infusion of 0.25 mL/kg/min (which may be doubled in persistent hypotension) until hemodynamic recovery.53 This regimen has been generalized for treatment of severe poisoning with other local anesthetics and cardiovascular toxins.
Of note, ILE administration too early after oral poisoning has the potential to facilitate gastrointestinal tract drug absorption or redistribution, exacerbating toxicity and, in theory, compromising the efficacy of lipid-soluble antidotes and concomitant therapies.54,55 Until further evidence demonstrates ILE superiority, it is used as a last resort when conventional therapies have failed. Potential adverse effects of ILE include hypersensitivity reactions (from egg or soybean allergies), fat embolism and pulmonary toxicity, hyperamylasemia and pancreatitis, acute myocardial infarction, altered coagulation, and laboratory interference with normal serum laboratory testing.
Naloxone
Naloxone has been employed to reverse the effects of clonidine overdose. Naloxone antagonizes the effects of endogenous opioids, particularly in patients with higher baseline concentrations.56 Although less than half of clonidine-intoxicated patients will respond to naloxone,57,58 given the potential CNS and hemodynamic benefits, a trial dose may be warranted. High doses may be necessary and should be followed by a continuous infusion.56 Double-blind controlled studies demonstrate that naloxone pretreatment or coadministration mitigates acute captopril-induced hypotension.59,60 However, in cases of acute RASA ingestion or in patients with chronic hypertension, naloxone has shown inconsistent efficacy.61–63
Sodium Bicarbonate
Sodium bicarbonate exerts two therapeutic effects on the combined sodium channel blockade and QRS widening associated with CAs and other toxins. First, supplemental sodium mitigates channel blockade (as does hypertonic saline). Second, the bicarbonate-induced alkalemia increases the nonionized drug fractions, leading to a decrease in toxin–sodium channel binding.64 The use of sodium bicarbonate may also mitigate hypoperfusion or seizure-induced acidemia, which can worsen channel blockade. An intravenous bolus of 1 to 2 mEq/kg of 7.5% to 8.4% sodium bicarbonate is followed by an infusion of 132 to 150 mEq in 1 L D5W at a rate of 150 to 200 mL/h, with the goal of narrowing the QRS complex to 100 milliseconds or less and targeting a serum pH 7.45 to 7.55. Rebolusing may be required for recurrent QRS widening. Alkalemia-associated hypokalemia should be anticipated with bicarbonate therapy, and treated appropriately.65,66
Experimental Antidotes
Methylene blue, presumably functioning as a nitric oxide scavenger, has anecdotally been employed to reverse refractory vasodilatory shock from BAAs and amlodipine. Dosing has ranged from 1 to 2 mg/kg over 10 to 20 minutes, followed by an infusion of 1 mg/kg/h.67,68
CONCLUSION
The treatment of critically ill cardiotoxic patients attempts to identify and reverse the effects of the responsible agent(s), to decontaminate as appropriate, and to provide aggressive supportive care with pharmacologic or adjunctive therapies. Targeted antidotal strategies include calcium, DSFab, glucagon, HIE, ILE, naloxone, or sodium bicarbonate, based on suggestive history or clinical, ECG, or laboratory findings. After initial decontamination and stabilization, patients should be admitted to an intensive care setting for continued monitoring and therapy.
LITERATURE TABLE

Full access? Get Clinical Tree
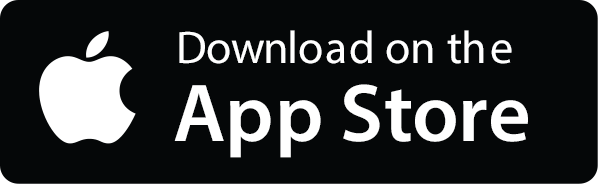
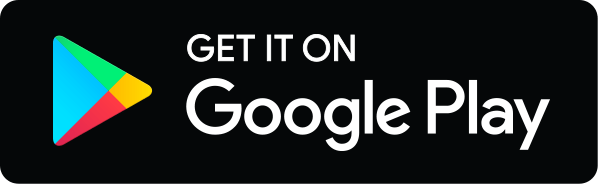