Chapter 26 Cardiopulmonary Interactions
Effects of Ventilation on Circulation
As shown by Cournand1 in his sentinel paper, mechanical ventilation can have important effects on circulation. The magnitude of these effects may be accentuated by factors that compromise cardiovascular adaptability, such as hypovolemia, cardiac dysfunction, or disordered vascular tone.
Right Ventricular Filling and Stroke Volume
Venous Return
The mean systemic pressure of the circulation (Pms) is thought to be the inflow pressure driving blood toward the right ventricle.2 This driving pressure is not measurable in the intact patient, but it can be thought of as the static mean pressure that might exist throughout the circulation if there were no blood flow.3 Pms approximates the weighted average of pressures in venous reservoirs throughout the body during the circulation of blood.4 The backpressure that opposes flow toward the right heart is the right atrial pressure (Pra). The impact of these pressures on return of venous blood to the heart is described by the venous return curve (Figure 26-1, A). Picture the systemic circulation as compliant arteries and veins separated by high-resistance arterioles and a pump that receives venous return and propels it into the arteries (Figure 26-1, B). The faster the pump circulates the blood, the more blood piles up before the arterioles and the higher the arterial pressure will be. In contrast, the faster the pump moves blood from vein to artery, the less blood resides on the venous side of the circuit and the lower the venous pressure will be. As the pump is slowed down, venous pressure rises until flow reaches zero and pressure equilibrates throughout the circulation at Pms. Resistance to venous return (Rvr) is the reciprocal of the slope of the linear part of the venous return curve. Simply stated:
Pms is sensitive to the volume of blood in the circulation and to the capacitance of the venous reservoir. It can be altered by transfusion, volume infusion, hemorrhage, and diuresis. It also is sensitive to changes in venous tone. Pms is an extrathoracic measurement and is less sensitive than Pra to changes in intrathoracic pressure.5 Pra, in contrast, is quite sensitive to changes in intrathoracic pressure.
At functional residual capacity (FRC), the thorax exerts recoil force, tending to spring outward, whereas the lung exerts recoil force (mostly as a result of alveolar surface tension), tending to collapse inward. These forces result in subambient pleural pressure. The cardiac fossa, or juxtacardiac space, which surrounds the pericardium and heart, shares in this balance of forces and has slightly negative pressure at apneic FRC. At any right atrial volume, Pra is influenced by juxtacardiac pressure because these two forces act together to oppose the right atrium’s balloonlike tendency to recoil inward. Therefore it is not surprising that all of these pressures (pleural, juxtacardiac, and right atrial) are influenced by the respiratory cycle.6–8
During spontaneous breathing, lung volume rises from FRC to end-inspiratory volume by expansion of the rib cage and descent of the diaphragm. This reshaping of the thorax stretches the lung, increasing its recoil tension, so pleural pressure and juxtacardiac pressure both become more negative (subambient). At any right atrial volume, this process reduces Pra. Therefore spontaneous inspiration reduces pleural, juxtacardiac, and right atrial pressures. By the mathematical relationship in Equation 1, this augments venous return.9 Over the course of passive spontaneous expiration, all three pressures return toward their values at FRC. It follows that intrathoracic pressure, during relaxed spontaneous breathing, is always least negative at end-inspiration and falls continuously throughout the rest of the respiratory cycle.
Right Ventricular Preload and Stroke Volume
Transmural pressure is the pressure difference across (inside to outside) a hollow structure. This pressure difference and the wall tension of the structure determine its radius. Pra approximates the pressure within the right ventricle during cardiac filling. Juxtacardiac pressure approximates the pressure surrounding the ventricle. During spontaneous inspiration, systemic venous return to the right atrium and ventricle are augmented (Equation 1), and end-diastolic ventricular volume rises. Pra falls, but not as much as juxtacardiac pressure falls. Transmural pressure is, therefore, increased by spontaneous inspiration. Despite the falling Pra, right ventricular stroke volume normally rises during spontaneous inspiration; hence, there is a paradoxical inverse relationship between Pra and right ventricular stroke volume over the spontaneous respiratory cycle (Figure 26-2).10 If transmural Pra is plotted against right ventricular stroke volume during various respiratory maneuvers, the expected positive slope is revealed (Figure 26-3).11
Positive Pressure Mechanical Ventilation and Right Ventricular Preload
During spontaneous inspiration, active reshaping of the thorax by the respiratory muscles and diaphragm inflates the lungs by reducing pleural pressure. In contrast, throughout positive pressure mechanical inspiration, pleural pressure rises because the passive thorax is pushed outward (from FRC to end-inspiratory volume) by the expanding lungs. Passive expiration restores pleural pressure to that of FRC. Averaged over the entire respiratory cycle, pleural pressure is higher during positive pressure breathing than it would be during spontaneous breathing (Figure 26-4). (This elevation of pleural pressure during positive pressure mechanical ventilation is generally called transmission of airway pressure to the pleural space.) Positive pressure ventilation, therefore, reverses the effects of spontaneous breathing on venous return12 and right ventricular transmural pressure.
Positive pressure inspiration impedes venous return by raising Pra. Right ventricular stroke volume declines over positive pressure inspiration because right ventricular transmural pressure is reduced. Averaged over the entire respiratory cycle, Pra is raised and right ventricular stroke volume and cardiac index are reduced by positive airway pressure relative to their expected values during spontaneous breathing (Figure 26-5).
Critical Illness and Effects of Positive Pressure Breathing on the Right Heart
Among the effects of critical illness are capillary leak, chest wall edema, pulmonary edema, surfactant dysfunction, abnormal blood volume, and abdominal distension. Each modifies the effects of positive pressure breathing on the right heart. Capillary leak alters the compliance of the atrial and ventricular chambers, modifying the responsiveness of the heart to changes in preload. Sepsis and inflammation decrease cardiac contractility, directly altering the way the heart responds to changes in preload. Chest wall edema, pulmonary edema, surfactant dysfunction, and abdominal distension alter thoracic and pulmonary compliances, which in turn alter pleural and juxtacardiac pressures.
Reduced respiratory system compliance diminishes the transmission of alveolar pressure to the juxtacardiac space.13 The change in intrathoracic pressure that occurs with a change in static airway pressure is essentially the same as the change observed in pulmonary artery wedge pressure,14 which is readily measured. Recognizing this relationship in adults has made it possible to estimate percent transmission of airway pressure to the juxtacardiac space by measurement of respiratory system compliance (Figure 26-6).
Pulmonary Circulation
Lung Volume
The alveolar septae are highly vascular (Figure 26-7). More than 90% of the alveolar surface makes contact with alveolar capillaries. These vessels can be separated into two categories according to their location or their response to lung inflation. Most alveolar vessels are capillaries and lie in septa, which separate adjacent alveoli. Other alveolar vessels are termed corner vessels because they are located at the intersection of alveolar septa. These corner vessels are generally larger and most likely will divide later in their course to become alveolar capillaries located in septa between adjacent alveoli. When the lung is stretched, either by spontaneous inspiration or by positive pressure distension, corner vessels are pulled open by radial traction and their resistance to blood flow is reduced. When alveolar septa are stretched, alveolar capillaries thin and restrict flow. The net effect of these factors is a U-shaped relation of PVR to lung volume (Figure 26-8)15–18; PVR is least at FRC and rises with either atelectasis or overdistension.
Alveolar Pressure
When alveolar pressure is greater than ambient, as it is during positive pressure ventilation, the vessels that course through alveolar septae between adjacent alveoli can be compressed.19,20 This behavior is akin to that of a Starling resistor, a collapsible tube traversing a rigid housing (Figure 26-9). Flow (Q) is propelled through the tube by inflow pressure (Pi) and is opposed by outflow pressure (Po). The tubing has some intrinsic resistance (R). If the housing is pressurized to a surrounding pressure (Ps), flow through the tube is determined as follows:
Except when Ps < Po, alveolar pressure appears to modulate local pulmonary blood flow as though it surrounds the pulmonary capillary (Figure 26-10).
From this discussion, the degree to which Ps affects pulmonary blood flow is influenced by the magnitude of inflow pressure, which, for the pulmonary capillary, must be adjusted for vertical height. Alveolar pressure causes greater reduction in flow at low pulmonary artery pressure than it does at high pressure. Pulmonary hypertension (high Pi) dampens this cardiopulmonary interaction, whereas hypovolemia (low Pi) accentuates it.
Hydrostatic pressure in the lung is a function of vertical height (see Chapter 20).21 To estimate the hydrostatic inflow pressure of a pulmonary capillary, a pressure equivalent to that exerted by a water column extending from the left atrium to the capillary must be subtracted from the pressure within the main pulmonary artery. The greater the vertical height of the pulmonary capillary, the lower its inflow pressure and the greater the attenuation of flow by alveolar pressure. This can produce areas of no flow, especially at peak inspiration, high in the supine lung. These high ventilation/perfusion ratio (Va/Q) alveolar capillary units waste ventilation and can cause hypercarbia (see Chapter 40). Vertical height (h) of the capillary also alters the backpressure to flow. At a left atrial pressure of 10 cm H2O (7 mm Hg), for example, there is (10 − h) cm H2O opposing flow up to a vertical height 10 cm above the heart. Above that, there is no back pressure, and Pi and Ps determine flow through the capillary. Compression of pulmonary capillaries is a local phenomenon. It can divert pulmonary blood flow away from normal lung segments toward consolidated or atelectatic lung segments whose airways do not effectively transmit airway pressure to the alveolus.22 By this mechanism, the application of high PEEP in the presence of lobar pneumonia may increase blood flow through unventilated lung and worsen hypoxemia (Figure 26-11). From a more positive perspective, PEEP may relieve atelectasis and improve ventilation, thereby relieving alveolar hypoxic vasoconstriction. Whether PEEP benefits or impairs pulmonary blood flow may depend on the balance of its effect on atelectasis and its effect on alveolar capillaries.
Regulation of Pulmonary Vascular Resistance
The resistance to flow through a vessel is described by the following:
where η is viscosity, l is length, and r is radius. It follows that PVR can be effectively controlled by active changes in vessel radius. Mechanical ventilation may alter blood pH and alveolar oxygen tension (pO2), both of which influence vessel tone and radius (Figure 26-12).23,24
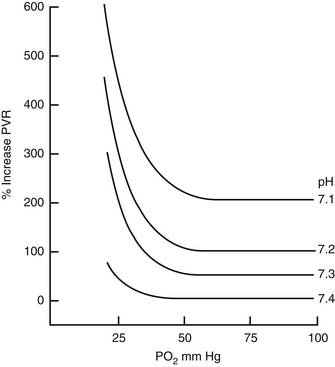
Figure 26–12 Pulmonary vascular resistance (PVR) is a function of both pH and arterial pO2.
(From Rudolph AM, Yuan S: Response of the pulmonary vasculature to hypoxia and H+ ion concentration changes, J Clin Invest 45:399, 1966.)
Hypoxic pulmonary vasoconstriction is a powerful mechanism for sustaining systemic oxygenation in the face of lung disease.25–28 Relief of atelectasis and restoration of segmental ventilation not only increases the fraction of the lung that is ventilated but also restores blood flow to those segments by several mechanisms. Segmental alveolar hypoxia is relieved. Segmental volume is restored, which returns segmental vascular resistance toward its volume-dependent nadir. Gas exchange is also improved, favorably altering pH, pCO2, and pO2, and thereby reducing global PVR.
Direct Effects of Airway Pressure on Pulmonary Vascular Tone
Pulmonary vessels are stretched by lung inflation. The lung of infant lambs responds to abrupt changes in airway pressure with changes in vascular tone. Abrupt distension of one lung of the intact infant lamb increases the PVR of that lung alone.29 The resistance change is sensitive to the waveform of the lung distension30 and persists for some time after relief of distending pressure and return of lung volume to baseline.31 This effect is calcium channel dependent32 and resembles a myogenic reflex whereby direct vessel stretch causes constriction.

Full access? Get Clinical Tree
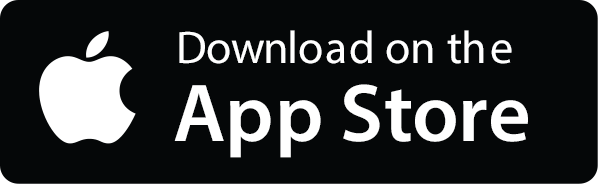
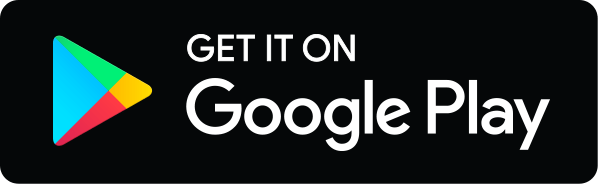