Perfusion science is a unique discipline unto itself and a full discussion of its many intricacies is far beyond the scope of this introduction to cardiac anesthesia and echocardiography. Still, much that is unique to cardiac anesthesia care can be in some degree related to the use of cardiopulmonary bypass (CPB). At the start it is important for the practitioner new to cardiac anesthesia to establish a close working partnership with their perfusionist colleagues. Perfusionists are certified healthcare professionals who devote their careers to the management of circulatory support. In most institutions they work under the direct authority of the attending surgeon; however, from time to time they are under the medical direction of the anesthesiologist. At no times must they be considered a substitute for an appropriately qualified anesthesia practitioner in the operating room. Hence, during the “bypass run” a member of the patient’s anesthesia team must be physically present in the operating room. During CPB, the anesthesiologist and the perfusionist work together to bypass the functions of the heart and the lungs so that cardiac surgery may proceed. The pump’s flow becomes the patient’s cardiac output (CO). The oxygenator of the CPB machine provides gas exchange. Simply put, the hemodynamic principles, which guide normal patient management, are operative when the bypass machine is in use. Blood pressure is still the product of cardiac output and systemic vascular resistance—except that the CPB machine provides the cardiac output.
Figure 17–1. Venous blood is drained from the patient, flows through the pump oxygenator, and is returned oxygenated to the arterial system. Other functions of a simple CPB circuit is to deliver cardioplegia solution to the heart as well as suction blood from the surgical field to be oxygenated, filtered, and returned to the patient.
THE PLUMBING OF CPB
CPB has been in use in cardiac surgery for more than 50 years. It is likely that over that time successive generations of anesthesia trainees have been initially overwhelmed by the complexity of the bypass machine. Nonetheless, the basic circuit is straightforward enough (Figure 17–1). Anticoagulated venous blood is drained from the right atrium or directly from the superior and inferior venae cavae through the venous cannula and associated tubing to the pump reservoir. The deoxygenated venous blood is returned to the patient after passing through a gas exchanger or oxygenator as well as a heat exchanger to control the desired blood and hence patient temperature. In the oxygenator, carbon dioxide is swept away by the oxygen gas flow across a gas permeable membrane. Oxygenated blood is then returned to the patient through a cannula most often placed in the ascending aorta or occasionally in a femoral artery. Along the oxygenated blood’s course there are a number of filters and alarms to prevent the perfusionist from pumping air or clot into the aorta resulting in a perioperative embolic catastrophe.
Of course, the pump looks more formidable than the simple description given above and to be sure, it is. One very useful exercise is to visit with the perfusionist with whom one works and trace and examine the flow of blood to and from the patient with the particular equipment employed.
BYPASSING THE HEART
Before initiation of CPB, the CPB machine is “primed” with a volume of crystalloid, colloid or blood. Following anticoagulation and cannula placement, release of clamps on the venous cannula commences CPB. Blood drains from the patient into the bypass machine and the perfusionist starts the pump flow. Assuming the aortic valve is competent, following institution of CPB, the heart will be drained of blood by the venous cannula and becomes empty. At the same time, the normal arterial waveform loses pulsatility as the main driver of aortic blood flow becomes the non-pulsatile pump of the bypass machine. Consequently, full bypass flow is non-pulsatile. However, it is important to remember that the presence of a pulsatile aortic pressure wave does not preclude the patient’s circulation being sustained by the CPB machine. As long as the heart has an electrical rhythm that can sustain a coordinated contraction, it will continue to beat and will eject any blood delivered to it. At times, the venous cannulae do not sufficiently drain venous blood into the bypass machine resulting in some venous return going to the heart—producing partial bypass. At other times, the surgeons will reduce venous return to the bypass machine intentionally leaving the patient on partial bypass. Patients with aortic insufficiency often fill their heart when on bypass and may develop distended hearts during CPB due to the incompetent aortic valve. Therefore in these patients, until the aorta is cross-clamped, it is important to keep the heart ejecting in order to prevent the heart from overdistention and ischemic injury. If the heart is ejecting, the patient will have both a pulsatile waveform and be supported by partial bypass. Pulsatility on the arterial pressure trace during CPB can also be observed when an intra-aortic balloon pump is triggering. When unsure whether the patient is or is not on full or partial bypass ask the perfusionist and surgeon to avoid serious management errors.
Hemodynamic basic principles apply equally to the patient on or off bypass support.
Recall,
BP = CO × SVR
where,
CO = SV × HR
When the patient is placed on CPB, CO is no longer provided by the patient’s heart but by the bypass machine. If all of the venous blood is delivered through the venous cannulae to the bypass machine, the patient is on total or “full flow” bypass. If on the other hand, some venous blood returns to the patient’s heart through the caval veins then the patient is on “partial” bypass. It is important to note that when the patient is on full flow all of the blood flow will pass through the CPB machine’s oxygenator and hence all of the blood will be oxygenated. On the other hand, that part of the patient’s blood flow that returns to the patient’s heart will be oxygenated by the patient’s own lungs. Since, the lungs are deflated during bypass it is important to remember to ventilate and oxygenate the patient if partial bypass is employed.
For example, if the patient’s total bypass flow is 5 L/min this would mean that the CPB machine is doing the work of a cardiac output of 5 L/min. If however, 2.5 L/min of venous blood flow returns to the patient’s heart then the machine only would pump 2.5 L/min of flow and the heart would provide the other 2.5 liters of cardiac output. Now if the 2.5 L/min of cardiac output were to pass through lungs that were not ventilated this would mean that the patient when on CPB would have a 50% pulmonary shunt—and a greatly reduced PaO2. Therefore, it is always important to make sure that ventilation is ongoing when the patient is on partial bypass.
Blood pressure when on CPB is the product of CO and SVR just as in any patient. The cardiac output when on total bypass or “full flow” is the pump flow—namely how many liters per minute the bypass machine is delivering to the patient. When on partial bypass, the CO is the sum of flow from the pump and the flow ejected by the native heart. As in any patient whether on or off CPB, SVR is determined by many factors including sympathetic tone, temperature, inflammatory mediators, and blood viscosity. SVR can be adjusted on CPB by the use of vasodilators and anesthetic agents to reduce tone or vasoconstrictors to increase tone.
The amount of flow that the perfusionist can deliver to the patient is dependent upon the venous return to the reservoir. If the patient is vasodilated, venous return can be reduced and the perfusionist will need to add volume to the reservoir to maintain flow. Restoring vascular tone will increase venous return and increase blood pressure for the same pump flow.
Thus, when on CPB the perfusionist can increase blood pressure by either increasing pump flow and/or SVR. Conversely, lowering pump flow or vasodilating the patient can reduce BP.
But what is the optimal blood pressure and flow when on CPB?
There are no nationally agreed standards as to what constitutes optimum perfusion; however, Murphy et al have recently attempted to provide some evidence-based guidance on best perfusion practices.1 Some have advocated lower mean aortic pressure on CPB of 50 to 60 mm Hg. This has been suggested because a mean arterial pressure (MAP) of 50 mm Hg is thought to be the lower limit of cerebral autoregulation. Others have suggested that a MAP of 70 to 80 mm Hg be employed.2 In particular, the elderly patients now routinely being operated for cardiac disease often have hypertension and preexisting cerebral vascular disease. Such patients might do better with relatively higher MAPs during bypass. Although, there are no definitive studies to suggest an optimum MAP, while on CPB there is limited data to suggest that elderly, diabetic, and hypertensive patients might do better with higher bypass MAP during CPB.1
Similarly there are no established guides as to what constitutes an “ideal” pump flow during CPB. Generally, flow is aimed at approximately 2.2 to 2.5 L/min/m2 since this is similar to the normal cardiac index of a normal patient. Of course, oxygen delivery to the tissues is dependent on the pump flow and also on the blood oxygen carrying capacity and tissue oxygen utilization. Reduced flows can be employed during hypothermic bypass. Moreover, deep hypothermic cardiac arrest permits periods of surgery with no pump flow at all. At present there is no standard flow that has been demonstrated as “ideal.” Rather, anesthesiologists and perfusionists must work together to establish for the individual patient an appropriate blood pressure and pump flow during CPB.
Delivery of oxygenated blood to the tissues is the main function of the pump. Tissue oxygen delivery is dependent not only upon pump flow but also upon hematocrit. However, on CPB hemodilution occurs due to the priming volume in the bypass reservoir. In patients who are profoundly anemic the reservoir is primed with blood. Nonetheless, as was discussed in the last chapter, even the use of one unit of homologous red cells can worsen perioperative outcomes. Of course, anemia during CPB and cardiac surgery can also lead to adverse outcomes as well. Hemodilution has benefits during CPB in that it can decrease blood viscosity and improve microcirculatory blood flow and oxygen delivery.3 There are no clear guidelines as to what constitutes an appropriate hematocrit during CPB. Ideally, blood transfusion should be avoided if possible and all efforts undertaken to minimize red cell loss perioperatively. As with many decisions, the risks and benefits of transfusion during CPB must be determined on an individual basis.
Calculation of the systemic delivery of oxygen (DO2) has also been suggested as a guide to determine the best combination of pump flow and blood’s oxygen carrying capacity during CPB.1
DO2 = Pump flow × (Hemoglobin concentration × Hemoglobin saturation × 1.36) + (0.003 × Arterial saturation)1
Normal DO2 is 350 to 450 mL/min/m2. A “critical” DO2 of 330 mL/min/m2 has been suggested in anesthetized patients as the point where maximal oxygen extraction occurs prior to the development of anaerobic metabolism.1 However, there is no established DO2 during CPB.1 If oxygen delivery does not meet oxygen consumption, patients develop tissue acidosis.
Perfusionists frequently monitor venous oxygen saturation during CPB to determine if there is adequate delivery of oxygen globally to the tissues. A decrease in venous oxygen saturation is indicative of either a decrease in the oxygen-carrying capacity or an increase in oxygen utilization by the tissues.
BYPASSING THE LUNGS
The pump oxygenator membrane provides the surface area over which gas exchange occurs during CPB. The oxygenator becomes the lungs of the patient when on CPB. Much as hemodynamic principles are constant for the patient on or off bypass, so too the basics of oxygenation and ventilation hold true for the patient on CPB. As with any patient, an increased PaCO2 can only occur because of either increased production or decreased ventilation. A low PaO2 only occurs secondary to absolute shunt or ventilation perfusion mismatch. It is important to keep these principles in mind when considering how the pump oxygenator allows bypass of the lungs.
The initial oxygenators delivered oxygen by bubbling oxygen into the venous blood. The bubble oxygenators produced red cell hemolysis and gas emboli and have been replaced by the membrane oxygenators.
The membrane oxygenator employs a microporous membrane that separates the ventilating gas in the bypass machine from the venous blood. Gas exchange occurs by the diffusion across the membrane. The ventilating gas mixture of oxygen, air, and inhalational anesthetics is delivered to the membrane. Increasing the gas mixture’s FiO2 will increase the PaO2 of the blood following passage through the oxygenator. Thus, oxygenating blood on the pump is easy and blood returned to the patient should be well oxygenated. Ventilation (CO2 removal) on the pump is dependent on the total gas flow in the oxygenator (the “sweep rate”). CO2 is thus blown away from the oxygenator depending on the flow of gas. Increase the “sweep rate” and the PaCO2 will decrease. This parallels the patient’s lungs—increasing ventilation decreases PaCO2.
Although oxygenating the blood is straightforward, the perfusionist’s main challenge is to ensure an adequate DO2. Generally, perfusionists monitor the saturation of the venous return. They aim for a venous oxygen saturation SvO2 of 65% to 80%. Anemia, reduced bypass flow, and increased oxygen consumption can all reduce SvO2. Decreasing temperature and increasing bypass flow can improve SvO2 by decreasing oxygen consumption and improving oxygen delivery. Increasing the patient’s hemoglobin will improve hematocrit; however, the reduced 2,3-DPG found in stored blood may not readily lead to increased delivery of oxygen to the tissues due to reduced tissue release of O2.
Hypothermia to 28°C is frequently employed in CPB to reduce tissue oxygen consumption. However, although hypothermia will decrease global oxygen consumption the overall effect on tissue oxygen delivery can be clouded as hypothermia shifts leftward the oxygen-hemoglobin dissociation curve meaning that the hemoglobin has a greater avidity for the oxygen it carries. Both normothermic bypass and hypothermic bypass are employed depending upon individual practice preferences. There is currently no established norm for the ideal temperature during bypass.1 Should hypothermic bypass be used the rate and degree of rewarming prior to separation from CPB has been associated with postoperative cognitive dysfunction.4 Limiting the temperature of the arterial blood returned from the pump to 37°C may reduce the incidence of neurological injury. Often “mild” hypothermic bypass to 32°C is currently employed in many centers.
Temperature during CPB also impacts the management of CO2 during bypass. PaCO2 during bypass is regulated in the same manner as CO2 in the breathing patient. An increased PaCO2 generally results from either the increased production or decreased ventilation of the gas. Causes of increased CO2 production during CPB are the usual culprits: malignant hyperthermia, hyperalimentation, thyrotoxicosis, and any hypermetabolic state. Ventilation as previously mentioned is dependent on the rate of sweep gas over the oxygenator to carry away CO2.
When hypothermia is used, however, PaCO2 can be influenced by the temperature-dependent change in carbon dioxide solubility. During hypothermia, the solubility of oxygen and carbon dioxide in blood is increased reducing the partial pressure of the gas. The impact of temperature upon CO2 solubility is at the center of the issue surrounding what is known as pH stat and alpha stat bypass management.
The increased solubility of CO2 causes a reduction of PaCO2 in the blood resulting in alkalosis. Since cerebral blood flow increases with increased PaCO2 and decreases with decreased PaCO2, many perfusionists have been concerned that the reduction in CO2 gas tension can cause a deleterious decrease in cerebral blood flow during CPB. Consequently, when pH stat management is employed, the perfusionist cools the patient on CPB and determines what the pH and PaCO2 are for the temperature measured. Since cooling causes the PaCO2 to be reduced, the perfusionist adds CO2 to the gas mixture to increase CO2 to correct for the effect of temperature on carbon dioxide solubility.
During alpha stat management, the perfusionist similarly cools the patient as indicated but in this instance does not supplement the gas flow with additional carbon dioxide. Alpha stat management is based on the concept that the homeostatic mechanisms of the body adjust for changes associated with decreasing body temperature.5
There is much debate in the literature as to which CO2 management scheme to employ in various patients. Alpha stat management has been suggested to be beneficial because it preserves autoregulation of cerebral blood flow and prevents cerebral hyperfusion. pH stat management provides for more global cooling of the brain and for less desaturation of jugular venous blood during rewarming. However, since most neurologic injuries are of an embolic nature, it is possible that pH stat management by increasing cerebral blood flow merely increases the opportunity for emboli to be delivered to the brain. There remains much uncertainty as to which pH management scheme if any offers improved neurological outcomes following CPB.5
PRESERVING THE HEART
Once the patient is placed on CPB, the heart is routinely isolated from the circulation by placement of the aortic cross clamp. The aortic cross clamp is placed below the aortic perfusion cannula. Once the cross clamp is positioned across the aorta, oxygenated blood no longer passes through the right and left coronary arteries. Without myocardial protection, the heart would become ischemic and die. However, myocardial preservation techniques permit the blood flow to the heart to be arrested and the myocardium protected permitting cardiac surgery to proceed. Close coordination between the perfusionist and surgeon is necessary to successfully deliver myocardium protecting cardioplegia solution to the patient’s heart. Although at most medical centers anesthesiologists have little to do with the delivery of cardioplegia solution, the results of inadequate cardioplegia are of great concern. Failure to adequately protect the heart during the period of aortic cross clamp can produce ventricular dysfunction and extreme difficulty in separating the patient from CPB.
Cardioplegia solutions usually contain a relatively high potassium concentration to produce electrical silence of the heart and are administered cold to reduce myocardial metabolism. Various surgical teams incorporate other electrolytes and blood into the solution, which can be administered in an anterograde manner via the coronary arteries or retrograde via the coronary sinus.
Anterograde cardioplegia is administered via a small cannula placed by the surgeon between the aortic cross clamp and the aortic valve. After application of the aortic cross clamp, anterograde cardioplegia is administered under considerable pressure using a separate pump on the CPB machine. Assuming the aortic valve is competent, pressure builds in the aortic root between the cross clamp and the aortic valve. Cardioplegia solution then flows through the left and right coronary arteries perfusing the myocardium in solution and arresting the heart. If the patient has significant aortic regurgitation, the heart will distend and solution will not pass via the coronary arteries. Rather, it will leak into the left ventricle where a venting catheter under mild suction will eliminate it to prevent the heart from distending. In patients with severe coronary artery disease, blockages can impede delivery of cardioplegia solution and hinder myocardial protection during the aortic cross clamp. In procedures where the aorta is going to be opened, the surgeon can directly perfuse both the right and left coronary arteries with cardioplegia.
Retrograde cardioplegia is given via a cannula placed in the coronary sinus (Video 17–1). The coronary sinus drains venous blood from the heart. A balloon cuff at the distal end of the retrograde cardioplegia cannula seals the outflow from the coronary sinus. Cardioplegia solution is delivered and a pressure builds in the sinus as cardioplegia flows back through the venous system of the heart to bathe the myocardium in cardioplegia solution. Pressure in the coronary sinus is measured so as not to exceed 45 mm Hg at the tip of the balloon catheter. Cold cardioplegia will produce myocardial cooling to 8°C to 10°C. Surgeons from time to time add cooled or frozen saline to the pericardium to further chill the heart externally.
Not all centers use cold cardioplegia and hypothermic bypass. Various combinations of temperature and solution mixtures are used. Clearly, one must learn the operating paradigm of their institution. Additionally, not all cardiac procedures require the use of CPB and not all patients placed on CPB require cardioplegia. Off-pump surgery for coronary revascularization was previously discussed. Some surgeons place the patient on CPB but do not use cardioplegia. They fibrillate the heart to keep it relatively quiet but do not use the cross clamp. This technique may serve to protect the heart with severely depressed prebypass ventricular function since the heart is not completely arrested and receives blood perfusion at all times during surgery. In patients having vein bypass grafts additional cardioplegia can be delivered via these grafts once the distal anastomosis has been completed.
PROTECTING THE BRAIN
There are countless studies which examine neurologic injuries associated with heart surgery and CPB in general.5 Roach et al in 1996 identified the high incidence of neurological injury associated with cardiac surgery.6 Injuries range from cognitive dysfunction detected by sophisticated neuropsychological studies to frank stroke. Etiologies of neurological injury include: particulate embolism, air embolism, and inadequate perfusion. Genetic factors and their influence upon the inflammatory response may also play a role in the development of neurological injury secondary to CPB.7
Genetic influences upon neurological outcomes are discussed in Chapter 14.
The grade of atherosclerotic plaque in the aorta has been associated with the development of stroke in patients requiring CPB. Patients with highly mobile plaque are at particular risk for the development of stroke in the CPB population.8 Avoiding manipulation of the aorta in patients with aortic plaque can reduce the incidence of stroke.9 Additionally, higher MAP (> 70-80 mm Hg) during CPB has been suggested to reduce the incidence of overt postoperative stroke in CPB patients.
TEE examination of the aorta and epiaortic ultrasound can be employed to guide placement of aortic cannula and aortic cross clamp away from areas of potentially embolic plaque. Epiaortic ultrasound examination is particularly useful in patients as TEE often fails to visualize the ascending aorta in the area of cannula placement. TEE can also be used to diagnose aortic dissections which may occur as complications of aortic cannulation.
Blood glucose control during cardiac surgery has been previously discussed. During hypothermic CPB blood glucose concentration often increases. Some cardioplegia solutions contain glucose making perioperative glucose management difficult. Maintenance of appropriate glucose control is the subject of ongoing debate. Both glucose control that is too tight or too loose has been associated with adverse outcomes in cardiac surgical patients.10 Glucose control during CPB can be difficult; however, regular insulin should be infused to maintain glucose according to those protocols locally operative.
Rewarming temperature and rate of rewarming have similarly been associated with adverse neurological outcomes.4 Arterial temperatures not greater than 37°C have been suggested to avoid thermally mediated cerebral injury.
There are various methods suggested to monitor the brain during CPB. Cerebral saturation monitors provide a global measure of brain tissue saturation. Likewise, monitoring of jugular venous bulb saturation has been used as a measure of the adequacy of cerebral perfusion during CPB. Decreased cerebral or jugular saturations could be suggestive of higher oxygen extraction or be secondary to insufficient oxygen delivery to the brain. However, since many neurological injuries are thought to be secondary to embolic processes rather than inadequate perfusion, the role of such monitors and how they should or should not be incorporated into routine patient management remains subject to debate and institutional preferences. Various neuroprotective schemes have appeared over the years to mitigate adverse neurological outcomes associated with CPB.5 Efforts have been directed to decrease cerebral oxygen consumption during CPB through pharmacologic means such as suppressing the EEG with propofol or treatment with barbiturates. These efforts have not affected the incidence of adverse neurological outcome following CPB.5 Investigations into the use of anti-inflammatory agents to reduce the incidence of adverse neurological events are likely to continue to be forthcoming as are investigations which attempt to identify genetic factors which predispose to a worsened outcome following CPB.
ELECTROLYTES AND CPB
Monitoring of blood gases and electrolytes during CPB is dependent upon institutional protocols. Point of care blood gas analyses generally provide information in a timely fashion. Most frequently hyperkalemia secondary to cardioplegia delivery can complicate the restoration of sinus rhythm prior to separation from CPB. Often a potassium concentration between 5 and 6 mEq/L is well tolerated. Patients with elevated potassium concentrations prior to separation from CPB may need to be treated with regular insulin and dextrose to drive potassium intracellularly. Furosemide can be given to augment a potassium losing diuresis. A mild respiratory alkalosis may similarly help to transiently lower elevated potassium concentrations prior to separation from CPB. Patients with renal failure may be acidotic at baseline and may require dialysis immediately postoperatively to eliminate potassium acquired in the cardioplegia solution.
Sodium concentration is usually well maintained during CPB and rarely requires treatment. Serum calcium is often low during bypass secondary to the dilution of albumin. Some blood gas machines measure ionized calcium; however, calcium administration during CPB or as an adjuvant to aid in separation from bypass is controversial. Although ionized calcium concentration may fall at the outset of CPB this is usually transient. Calcium chloride administration may impair the action of inotropes and its hemodynamic effects are short-lived.11 Nonetheless, cardiac surgery patients are often administered supplemental calcium chloride in lactated Ringer solution used for fluid maintenance. When a low ionized calcium is thought to contribute to a patient’s hemodynamic instability, 200 to 300 mg boluses of CaCl2 can be given. Routine administration of 1000 mg of CaCl2 prior to bypass separation is not recommended.
Occasionally patients develop a low-ionized magnesium concentration. Decreased ionized magnesium can lead to dysrhythmias and as such supplemental magnesium (1-2 g) can be given prior to separation from bypass if arrhythmias are present. Measurements of ionized magnesium are not routinely a part of point of care laboratories available in the operating suite.
Acid/base balance during CPB is largely dependent upon the delivery of suitable amounts of oxygen to the tissues. As discussed above what constitutes adequate delivery is predicated upon both flow, hemoglobin concentration, hemoglobin’s release of oxygen to the tissues, and tissue oxygen consumption. If oxygen delivery is not sufficient the patient will develop a metabolic acidosis. Management of metabolic acidosis on CPB is centered at correcting the underlying cause rather than simply giving the patient sodium bicarbonate. Should profound acidemia occur when on CPB it is important to consider not only malignant hyperthermia in the differential diagnosis but also the possibility of ischemic mesenteric organs. Emboli associated with CPB institution can produce multiple areas of organ ischemia. Patients with vascular disease may have inadequate mesenteric perfusion leading also to ischemia, inflammation, and vasodilatory states.
ANTICOAGULATION AND CPB
As mentioned in Chapter 4, anticoagulation is essential to prevent the development of clot as blood is exposed to the non-physiologic surfaces of the bypass machine. Both coagulation and inflammatory cascades are activated by the interface of blood with the bypass circuit. Some bypass circuits have attempted to attenuate the interface between blood and CPB machine by using heparin-bonded materials. Heparin coating of the bypass circuit appears to reduce both coagulation and inflammatory processes; however, the absolute benefit of these circuits has not as of yet been established.12 Additionally, heparin bonding to the CPB circuit does not eliminate the need for systemic anticoagulation.
Delivery and confirmation of adequate anticoagulation is essential before initiating CPB.
Generic, unfractionated heparin is most frequently used to achieve anticoagulation for CPB. Unfractionated heparin in the range of 3 to 4 mg/kg is administered intravenously (or by the surgeon directly into the right atrium) when requested. An activated clotting time and a heparin concentration assay are obtained following circulation of the heparin. The activated clotting time (ACT) is obtained by mixing a blood sample with a small amount of kaolin or diatomaceous earth. Contact initiates clotting that is timed. The normal ACT is 110 to 120 seconds. The acceptable ACT adequate for initiation of CPB is debatable but generally is considered to be 400 to 480 seconds. Heparin potentiates the action of antithrombin III more than 1000-fold and inhibits thrombin and factor Xa. Patients who are deficient in antithrombin III may become resistant to the action of heparin. Supplemental antithrombin III can be given through the transfusion of 2 to 4 units of fresh frozen plasma or if available an antithrombin III concentrate. If the initial ACT measure is too low, an additional heparin dose (1 mg/kg) may provide for adequate anticoagulation. However, it is reasonable to consider administration of supplemental antithrombin III if more than 6 mg/kg heparin has been administered in order to reach the target ACT.
Heparin can reduce ionized calcium concentration transiently following administration and as such at times patients vasodilate and become relatively hypotensive. This can be occasionally useful since surgeons often request that blood pressure be reduced at the time of aortic cannulation. Once the aortic cannula is placed and its correct position confirmed by the surgeon, the cannula can be a source of rapid fluid transfusion if necessary to compensate for transient hypotension. Restoration of vascular tone at this point with small amounts of vasoconstrictors such as phenylephrine can also be used.
With the increased use of heparin in the preoperative period not only do patients present with antithrombin deficiency but from time to time manifest heparin-induced thrombocytopenia.13 Unfractionated, generic heparin while inexpensive can induce thrombocytopenia via both immune- and nonimmune-mediated mechanisms. Nonimmunologically mediated heparin-induced thrombocytopenia (HIT) occurs secondary to the direct activation of platelets by heparin.14 Immune-mediated HIT is far more severe and occurs when primarily IgG antibodies are directed against platelet factor 4 (PF4-heparin) complexes. The binding of heparin to PF4 results in the expression of antigens which result in an immune response.14 Ultimately, the antibody response destroys and activates the platelets releasing prothrombic particles placing the patient at risk for systemic thrombocytopenia and thrombosis. HIT usually becomes manifest 5 to 10 days following heparin exposure heralded by an unexpected decrease in platelet count. Although a declining platelet count can be secondary to hemodilution, immunoassays can be used to detect the presence of immunoglobulins directed at the PF4/heparin complex. Not all patients who are exposed to heparin develop antibodies. Of those that do develop antibodies not all develop clinical HIT.
Management of CPB for the HIT patient requires the use of alternative anticoagulation approaches. Consultation with the patient’s hematologist and discussion of these options with the surgeon and the perfusionist should be undertaken in advance of bringing the patient to the operating room. There are a number of alternatives to heparin to provide for anticoagulation; however, none possesses the simplicity of delivery, ease of monitoring, and swiftness of reversal as done by heparin. Direct thrombin inhibitors such as bivalirudin or lepirudin can be used in the HIT patient. These agents directly inhibit the active site of thrombin as well as its binding to fibrinogen. Bivalirudin has a relatively short half-life but none of the direct thrombin inhibitors have a specific reversal agent similar to protamine. Consequently, patients can have prolonged bleeding following completion of the bypass run. Cardiac surgery is ideally avoided in patients with acute HIT. However, if necessary there are numerous guidelines that can assist in guiding management.15 All patients with previous or current HIT should be carefully reviewed with the surgeon and hematologist to outline a best plan for perioperative anticoagulation and the presence or absence of HIT antibodies determined.
Reversal of heparin anticoagulation with protamine was discussed in Chapter 4.
SYSTEMIC INFLAMMATION AND CPB
In addition to activating the coagulation cascade, CPB also contributes to the perioperative inflammatory response including complement activation, cytokine release, and leukocyte activation16 (Figure 17–2). The inflammatory response may contribute to the development of various postoperative complications including respiratory failure, renal dysfunction, neurological injury, and hemodynamic instability.
The contact of blood with the materials of the bypass circuit leads to activation of the inflammatory response. The coagulation, inflammatory, complement, and fibrinolytic systems are all closely linked and activated with CPB.16 Although anticoagulation inhibits clot formation the entire cascade is nonetheless active. Complement activation in and of itself can produce tissue injury secondary to neutrophil activation as well as C5a-mediated increases in capillary permeability and loss of vascular tone. CPB may also contribute to inflammation through the release of endotoxin from the patient’s gut. Additionally, ischemic/reperfusion to the heart following release of the aortic cross clamp may contribute as well to the perioperative inflammatory response in patients managed with CPB.
A variety of strategies have been suggested to mitigate the inflammatory response to CPB.14 Aprotinin, complement inhibitors, phosphodiesterase inhibitors, steroids, statins, and the avoidance of homologous blood products have all been employed to affect the inflammatory response to CPB. It is likely that efforts will be ongoing to further understand the genetic influences that contribute to the inflammatory response to CPB and its consequences.
ECHOCARDIOGRAPHY AND CPB
Echocardiography is useful in the CPB patient in two ways. First, echocardiography can help to determine where and if the aorta should be cannulated. Second, echocardiography can assist the surgeon in confirming the placement of various catheters as well as the adequacy of ventricular de-airing.

Full access? Get Clinical Tree
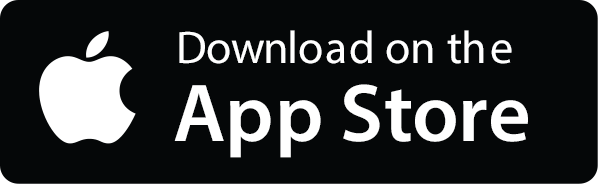
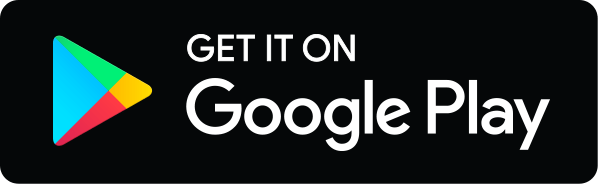