The subvalvular apparatus consists of chordae tendineae and the papillary muscles. The chordae tendineae attach to the edges of mitral leaflets or to its ventricular surface. The left ventricle has two papillary muscles: the anterolateral papillary muscle and the posteromedial papillary muscle. The chordae tendineae arise from the papillary muscle and attach to the ipsilateral half of anterior and posterior mitral leaflets.1,4
The left ventricle is a cone-shaped structure that is longer and narrower than the right ventricle. In the long axis, it descends forward and to the left from its base at the atrioventricular groove to form the cardiac apex. Its walls are normally about two to three times thicker than the right ventricle. It consists of the inlet region, the apical trabecular component, and the smooth-walled outflow tract. Unlike the valvular orifices of the right ventricle, the orifices of the aortic and mitral valve are in fibrous continuity. The left ventricular outflow tract ends at the aortic valve. The aortic valve consists of three semilunar cusps that are supported within the three aortic sinuses of Valsalva. The cusps and the sinuses are called left, right, and noncoronary.1,4
The Coronary Circulation
A unique feature of the coronary circulation is that the heart requires a continuous delivery of oxygen by coronary blood flow to function. At rest, the myocardium extracts about 75% of the oxygen delivered by coronary blood flow more than any other tissue in the body. So whenever myocardial oxygen demand increases, as with exercise, the coronary arteries must dilate to increase blood flow and oxygen delivery to meet the demand, or ischemia results. This coronary artery dilation is mediated through the local release of vasodilator substances within the myocardium.6,7
The right (RCA) and the left (LCA) coronary arteries arise respectively from the upper part of the right and the left coronary sinus of Valsalva and are the first branches of the aorta (Fig. 15-2). The RCA usually supplies the most of the right ventricle, a small part of the diaphragmatic aspect of left ventricle, the right atrium, part of left atrium, and posteroinferior one-third of interventricular septum. The first segment of the RCA gives rise to right ventricular and atrial branches. The right ventricular branches are the right conus artery or the infundibular artery and the acute marginal arteries. The atrial branch in majority of the population is the artery to the sinoatrial node. The RCA passes directly into the right interventricular groove and descends to the right cardiac border, where it curves posteriorly and approaches the junction of interatrial and interventricular grooves (the crux of the heart) and gives rise to the posterior descending artery (PDA). Coronary artery dominance is defined by the artery that supplies the PDA. In 70% of the population, the PDA is supplied by the RCA, coronary circulation referred to as right dominant. In 10% of the population, the PDA is supplied by circumflex artery (CxA) and is left dominant. In the remainder, the PDA is supplied by both the RCA and the CxA and the coronary circulation is considered codominant.1,6

The left main coronary artery usually supplies the free wall of the left ventricle, a narrow strip of the right ventricle anteriorly, the anterior two-thirds of ventricular septum, and most of left atrium. After arising from the left coronary sinus, the left main coronary artery passes to the left atrioventricular groove, where it branches into left anterior descending artery (LAD) and the CxA. The LAD, also known as anterior interventricular artery, runs in the anterior interventricular groove and gives off right and left diagonal and septal branches. The right diagonal branches are small and rare; the left diagonal arteries can vary in number anywhere from two to nine and cross the anterior surface of the left ventricle. The septal branches supply most of the interventricular septum. The first septal branch is usually targeted for ablation in interventional treatment for hypertrophic cardiomyopathy. The CxA curves left in atrioventricular groove giving rise to obtuse marginal branches extending over the posterolateral wall toward the apex. The CxA continues into the posterior part of the atrioventricular groove, usually terminating prior to the crux of the heart, but in 10% of individuals, it continues to supply the PDA.1,6
The cardiac veins that run in atrioventricular and interventricular grooves drain a large part of coronary arterial blood into the right atrium via the coronary sinus (CS). The great cardiac vein that accompanies the LAD is joined by the oblique vein of the left atrium in the left atrioventricular groove to become the CS. Prior to draining into the right atrium, the middle cardiac vein that runs in the inferior interventricular groove and small cardiac vein that runs in right atrioventricular groove drain into the CS.1,6
The Cardiac Conduction System
The cardiac conduction system consists of the sinoatrial (SA) node, the atrioventricular (AV) node, the AV bundle also known as the bundle of His, the bundle branches, and the Purkinje fibers (Fig. 15-3). The cardiac impulse is normally initiated by the SA node. This causes the atria to contract, first the right atrium followed by the left atrium. The blood supply to the SA node may be from either the RCA or the CxA.

From the SA node, the impulse is conducted to the AV node via the atrial myocardium. The AV node is located at the apex of region formed by the opening of the CS, the septal leaflet of the tricuspid valve, and the atrial septum, called the triangle of Koch. The AV node is supplied by the RCA in a large majority of population, in the rest by the CxA. The cardiac electrical impulse is delayed in the AV node about a fifth of a second before being conducted to the bundle of His and on to the ventricles, so that the atria contract just before the ventricles to augment end diastolic filling. The bundle of His branches into right and left bundle branches. The left bundle splits further into anterior and posterior fascicles. The bundle branches descend through the ventricular septum, where they continue on as the Purkinje fibers that end up directly stimulating the myocardium to contract. The bundle of His and bundle branches are insulated from the myocardium by a fibrous sheath, thus forming specialized network of conduction tissue. This functional organization results in a coordinated, synchronized contraction of the atria and ventricles, improving the efficiency of the heart.1,6,8,9
Clinical Electrophysiology and Electrocardiogram
Body fluids are good electrical conductors, making it possible to record the sum of the action potentials of the cardiac cells on the surface of the body. Continuous monitoring of this electrocardiogram (ECG) during anesthesia is considered to be a standard of monitoring for all patients under the anesthesiologist’s care. It is an essential tool for detecting myocardial ischemia, arrhythmias, and conduction system abnormalities.
Electrocardiogram Leads
The cardiac electrical activity is usually measured by electrodes placed on the skin. Bipolar leads consist of two electrodes, one positive and one negative. Unipolar leads consist of one positive electrode (exploring) and a composite pole that averages electrical activity from a number of other leads to zero potential, referred to as the indifferent electrode. Depolarization directed toward the positive electrode produces a positive deflection, whereas directed away from it produces a negative deflection. When the depolarization wave is perpendicular to the lead, a biphasic deflection is recorded (Fig. 15-4). The 12-lead ECG consists of three bipolar standard limb leads, six unipolar precordial leads, and three unipolar augmented limb leads. The standard limb leads and the augmented limb leads record electrical impulses that flow in the frontal plane, whereas the precordial leads record impulses in the horizontal plane.

Standard Limb Leads
The standard limb leads, named I, II, and III, record the potential difference between two points of the body (Fig. 15-5). In lead I, the electrodes are placed on the left shoulder (positive) and right shoulder (negative). In lead II, the positive electrode is on the left leg and the negative is on the right shoulder. In lead III, the positive electrode is on the left leg and the negative on the left arm. The limb leads form the triangle of Einthoven, which is used together with the augmented limb leads to calculate the electrical axis of the heart in the frontal axis. The direction of the depolarization of the atria parallels lead II, resulting in a prominent P wave in this lead.

Augmented Limb Leads
Augmented leads are similar to the standard limb leads but are unipolar. Linking the three limb leads through equal resistances (5,000 Ω), a central terminal is created with zero potential (Wilson’s central terminal). This is based on Einthoven’s theory that the R wave in lead II equals the sum of the R waves in leads I and III. The central terminal presents a stable reference potential point that is used to measure the varying potential at the exploring electrode (Fig. 15-6). Goldberger modified the central terminal by removing its link from the exploring electrode, achieving augmentation on the ECG deflections.10 The positive (exploring) electrode for augmented voltage right arm (aVR) is on the right shoulder, for augmented voltage left arm (aVL) on the left shoulder, and for augmented voltage foot (aVF) on the left leg.

Precordial Leads
The precordial (V1 to V6) are unipolar leads that are placed on the chest wall, with the exploring electrode over one of six separate points (Table 15-1). The “indifferent” electrode is represented by the Wilson’s central terminal, which is the average of the standard limb leads and normally has zero potential. The proximity of the heart surface to the electrode allows for detection of relatively small abnormalities in the ventricles without augmentation. Electric current flow is normally from the base to the apex of the heart. Therefore, leads V1 and V2, being near the base, record a negatively deflected QRS. Conversely, V4 to V6, being nearer the apex, record a positively deflected QRS.

Electrocardiographic Axis of the Heart
The axis represents the overall direction of the electric impulse in the heart and is created by averaging all the action potentials. It is biased toward the left because of the larger muscle mass of the left ventricle compared to the right. The standard limb leads combined with the augmented limb leads form the hexaxial diagram, which is used to calculate the electrical axis of the heart on the frontal plane (Fig. 15-7). This axis normally ranges between −30 and 90 degrees. Hypertrophy of the left ventricle shifts the axis to the left, and hypertrophy of the right shifts it to the right. Left axis deviation is defined as an axis less than −30 degrees and right axis deviation as more than 90 degrees. Abnormalities in the normal conduction pathway (blocks) in the heart also cause changes in the electrical axis.

Electrocardiogram Lead Systems
The three-lead system is the most basic ECG system. It consists of three electrodes, placed on the right arm, left arm, and left leg. It monitors electrical activity recorded by the bipolar standard limb leads (I, II, and II). Only one lead at a time is available. Two electrodes are used to form the selected lead and the third becomes the ground. Although the three-lead ECG provides adequate monitoring for arrhythmias, its use for detection of myocardial ischemia is limited.
In the modified three-lead bipolar standard limb lead system, the electrodes are placed in different locations on the chest wall. This allows for improved detection of arrhythmias (taller P waves) and monitoring for ischemia of the heart surface closer to each exploring pole. In the central subclavicular lead system (SC5), one electrode is placed under the right clavicle, one in the V5 position, and the ground electrode on the left leg. Other modified systems include the modified central leads (MCL), central manubrial (CM5), central back (CB5), and central cardiac 5 (V5) lead systems (Fig. 15-8).

The five-lead system uses five electrodes placed on the right arm, right leg, left arm, left leg, and one on the chest wall in any one position from V1 to V6. Seven leads at a time may be monitored, six bipolar (I, II, III, aVR, aVL, aVF) and one unipolar (usually V5.) The 10-lead system allows monitoring of 12 leads simultaneously, the 3 standard limb leads (I, II, III), the 3 augmented limb leads (aVR, aVL, aVF), and 6 precordial leads (V1 to V6). The electrodes are placed on the right arm and leg, left arm and leg, and on the anterior and anterolateral chest wall. This allows for monitoring of specific areas of the heart. Leads V1 to V4 monitor the anterior wall; leads I, aVL, V5, and V6, the lateral wall; and II, III, and aVF, the inferior wall.
London and colleagues11 examined lead sensitivity for detecting intraoperative myocardial ischemia. They concluded that using single-lead monitoring, V5 had the greatest sensitivity, 75%, whereas V4 had 61%. V4 and V5 combined had 90%. Sensitivity for the standard combination used in the clinical setting, II and V5, was 80%. Adding V4 to II and V5 increased the sensitivity to 96%. Although lead V4 is more sensitive in detecting ischemia, lead II is superior in monitoring atrial arrhythmias.11
Invasive ECG may monitor the cardiac electrical activity using leads placed in the trachea, the esophagus, in the cardiac cavities, or the coronary vessels but are not used in the routine anesthesia practice. The His bundle ECG uses an electrode on a catheter in the heart placed near the tricuspid valve (Fig. 15-9). It records the activation of the AV node (A), the spreading of the electrical activity through the His bundle (H), and the ventricular depolarization (V). Information from the standard ECG and the His bundle ECG can be used to measure the time required for the impulse to travel between the sinus node and the AV node (AH) and through the AV node to the bundle of His and the ventricles. This allows detection of the site of a conduction delay, important for prognosis and treatment.

Decreased voltage on the ECG may be caused by multiple small myocardial infarcts, which prevent generation of large quantities of electrical currents or abnormal conditions around the heart that impede current conduction from the heart to the skin, such as pericardial fluid and pulmonary emphysema.
It is important to differentiate artifacts from real ECG findings. Artifacts may be caused by malfunctioning ECG system (cables, connections, etc.), improper skin preparation (oil, hair), lead misplacement/poor contact, patient’s tremor/shivering, or muscular activity. External sources emitting electrical fields (electrocautery, 60 Hz power lines/light fixtures), cardiopulmonary bypass, somatosensory-evoked potential monitoring, and stimulators may also interfere with ECG recordings. All modern ECG monitors have incorporated filters for signal processing to minimize the presence of electrical artifacts.
Recording of the Electrocardiogram
The ECG is recorded on a graph paper consisting of 1-mm squares with every five squares separated by a darker line. Each 1-mm horizontal line represents 0.04 second and each 1-mm vertical line represents 0.1 mV, assuming proper calibration 1 cm/1 mV and standardized paper speed of 25 mm per second. Therefore, the distance between two darker lines represents 0.2 second and 0.5 mV on the horizontal and the vertical axes respectively. One minute (60 seconds) corresponds to 1,500 small or 300 big squares. The heart rate in beats per minute can be calculated by dividing 300 by the number of large boxes (or 1,500 by the number of small boxes) counted between two beats. Another method of calculating heart rate in beats per minute is to divide 60 by the number of seconds (= 0.04 second × number of small boxes) between two consecutive beats.
The electrical activity that activates the cardiac contraction is observed on a monitor display as a graph of voltage change through time. Modern monitors also have paper recorders allowing more thorough analysis of the ECG in complex situations. The Holter monitor is a small portable digital recorder that enables recording of the ECG for prolonged periods in ambulatory individuals to detect infrequent events.
Normal Electrocardiographic Deflections
Normally, the cardiac electrical impulse is initiated at the SA node, which is located at junction of the superior vena cava to the right atrium. Any part of the conduction system can spontaneously depolarize and initiate an impulse, but the SA node normally has the highest rate of depolarization and is the pacemaker of the heart. The impulse is transmitted through the right and left intraatrial pathways to the AV node and then to the bundle of His and through the Purkinje fibers to the ventricular subendocardium, which causes the myocardium to depolarize from the endocardium to the epicardium. Repolarization happens in the reverse direction, from the epicardium to the endocardium. The speed of conduction is different between the various areas of the system, with the slowest through the AV node producing AV synchrony for optimal end diastolic filling from atrial contraction. The normal ECG consists of a P wave (atrial systole), a QRS complex (ventricular systole), and a T wave (ventricular repolarization). The atrial repolarization wave is obscured by the larger QRS complex (Fig. 15-10).

P Wave
The P wave represents the atrial depolarization and has a normal duration and amplitude of 0.08 to 0.12 second and less than 2.5 mm in the limb leads. Morphologically, it is positive in the standard limb leads and negative in aVR. Enlarged P wave signifies atrial enlargement.
P-R Interval
The P-R interval corresponds to the time from the beginning of the atrial depolarization to the beginning of the ventricular depolarization. It is measured from the start of the P wave to the start of the QRS and should not be confused with the PR segment, which is measured from the end of the P wave to the start of the QRS. The normal duration of the P-R interval is 0.12 to 0.2 second. Prolonged P-R interval is called first-degree AV block. In pericarditis, the P-R interval is depressed in most leads (whereas ST is elevated) and elevated in aVR (knuckle sign) (Fig. 15-11).

Q Wave
The Q wave is defined as an initial negative deflection of the QRS and is usually absent in leads aVR, V1, and V2. Normally, its duration is shorter than 0.04 second and its amplitude less than 0.4 to 0.5 mV. A Q wave whose amplitude is more than one-third of the corresponding R wave, duration is longer than 0.04 second, and depth is greater than 1 mm, is indicative of myocardial infarction (MI). The Q wave occurs because there is no electrical activity in the affected area. Therefore, the direction of the sum of the action potentials in the specific plane changes and is recorded accordingly. In the presence of anterior wall MI, Q waves develop in leads V2 to V4; in anteroseptal MI, in leads V1 to V3; in anterolateral MI, in leads V4 to V6 and I and aVL; in lateral MI, in leads V5, V6, I, and aVL; and in inferior MI, in leads II, III, and aVF.
QRS Complex
The QRS complex is caused by the depolarization of the ventricles and normally has a duration of less than 0.10 second. A prolonged QRS duration may be due to left ventricular hypertrophy (LVH), impaired ventricular conduction (bundle branch block), beats initiated outside the conduction system (ectopic or paced beats), and beats passing through abnormal conduction pathways (Wolff-Parkinson-White syndrome). Normally, the QRS amplitude gradually increases in the precordial leads from V1 to V5, a phenomenon called R wave progression. The R wave is usually upright in the limb leads and downwardly deflected in aVR and V1. Various criteria exist for defining LVH using the QRS amplitude. According to the Cornell criteria, LVH is present when the sum of the R wave in aVL and S wave in V3 is greater than 28 mm in males and 20 mm in females. Based on the Sokolow-Lyon criteria, LVH is present when SV1 + (R in V5 or R in V6) is greater than 35 mm or R in aVL is greater than 11 mm.
ST Segment
The ST segment starts when all myocardial cells are depolarized (end of QRS) and ends when ventricular repolarization begins (T wave). It is normally isoelectric and elevation or depression more than 1 mm from the baseline may indicate myocardial ischemia. Hyperkalemia and pericarditis may also cause ST elevation. J point elevation (where QRS and ST segment connect) and normal variants of early repolarization are nonpathologic findings that must be excluded (Fig. 15-12).

T Wave
The T wave is caused by the repolarization of the ventricles, and its normal amplitude is less than 10 mm in the precordial leads and 6 mm in the limb leads. Usually, in any lead, the T wave is deflected in the same directions as the QRS. Delay of conduction of cardiac impulses through the ventricles (prolonged depolarization), as occurs with myocardial ischemia, bundle branch blocks, or ectopic ventricular beats, may result in T-wave polarity opposite the QRS complex. Symmetrical or deeply inverted T waves may be indicative of myocardial ischemia. Inverted T waves in V1 to V3 in children (juvenile T waves) and occasionally in women can be a normal variant. Peaked T waves may be present in hyperkalemia, LVH, and intracranial bleeding.
U Wave
The U wave, when present, follows the T wave and it probably represents part of the ventricular repolarization. It is most prominent in V2 and V3. Causes of increased U wave amplitude (>1.5 mm) include LVH, bradycardia, ischemia, electrolyte abnormalities (low potassium, magnesium, calcium), and medications.
QT Interval
The QT interval includes both ventricular depolarization and repolarization. It is measured from the beginning of the QRS to the end of the T wave and varies in duration according to heart rate. The QT interval corrected for heart rate (QTc) is calculated by dividing the QT interval by the squared root of the RR interval. Prolonged QTc (>0.44 second) may be associated with ventricular arrhythmias. Causes of prolonged QT include hypocalcemia, hypomagnesemia, medications, severe bradycardia, hypothermia, ischemia, intracranial hemorrhage, and myxedema of hypothyroidism.
Cardiac Physiology
Myocardium
The myocardium is the involuntary, striated muscle tissue in the heart between the epicardium and the endocardium; its cells are called cardiomyocytes. The primary structural proteins of the cardiac muscle are actin and myosin filaments, which interdigitate and slide along each other during contraction in a manner similar to skeletal muscle. But unlike the skeletal muscle in which the actin and myosin filaments are linear and longitudinal, in cardiomyocytes, they are branched (Fig. 15-13).12 Also, the cardiac T tubules are larger and broader and fewer in number than skeletal muscle. Cardiac muscle T tubules form diads with the sarcoplasmic reticulum intercalated discs with permeable junctions that allow rapid diffusion of ions so that action potentials travel easily from cell to cell. Thus, cardiomyocytes are functionally interconnected in a syncytium, so that activation of one cell results in the spread to all connected cells. The atrial syncytium is separated from the ventricular syncytium by the fibrous tissues around the AV valves, and the cardiac action potential is normally only conducted from the atria to the ventricles by a specialized conduction pathway through the AV node.13

Cardiac Action Potential
At the initiation of an action potential in a cardiomyocyte, the cell membrane rapidly depolarizes as the transmembrane potential rises from −85 mV to +20 mV. The membrane remains depolarized for about 0.2 second, the plateau phase, which is then followed by rapid repolarization. This plateau causes the contraction of a cardiomyocyte to last much longer than a skeletal muscle cell and is due to the slow calcium channels, which open after the sodium channels and remain open several tenths of a second. Depolarization of the cardiomyocyte is also prolonged by a decrease in permeability of the potassium channels after initiation of the action potential, another difference from skeletal muscle. During the plateau phase, the cardiomyocyte cannot be restimulated for about 0.25 to 0.3 second, called the refractory period. This is followed for additional 0.05 second by the relative refractory period, when the myocardium can only be stimulated by a strong excitatory signal.12,14
Excitation–Contraction Coupling
Excitation–contraction coupling in both cardiac and skeletal muscle occurs when the action potential spreads into the cell through transverse tubules (T tubules). Depolarization of the T tubule causes influx of calcium into the sarcoplasm, which binds to troponin activating the contraction of actin and myosin filaments. In the cardiomyocyte, however, the initial influx of calcium ions is just a small fraction of the amount needed for contraction, and it triggers an additional release of calcium from the sarcoplasmic reticulum into the sarcoplasm. The structural differences between cardiac and skeletal muscle reflect the difference in coupling mechanism. Myocardium has sparser and less developed sarcoplasmic reticulum and the T tubules are larger and store more calcium.15,16
Control of Cardiac Function
Neural Control
Heart function is controlled by the autonomic nervous system by both adrenergic and muscarinic acetylcholine receptors, modulating the cardiac output by influencing heart rate and myocardial contraction. The sympathetic nervous system, through adrenergic receptors with the neurotransmitter norepinephrine, has positive inotropic, chronotropic, and lusitropic effects on the heart. The parasympathetic nervous system, acting through muscarinic receptors with the neurotransmitter acetylcholine, has a more direct inhibitory effect on the heart through the vagal nerve reducing heart rate, AV node conduction, and cardiac contractility. The atria are innervated by both the sympathetic and parasympathetic nervous system, but the ventricles are supplied principally by the sympathetic nervous system (Fig. 15-14).12,17

Sympathetic nervous system fibers to the heart continually discharge at a slow rate maintaining a strength of ventricular contraction about 20% to 25% above the unstimulated state. Maximal sympathetic nervous system stimulation can increase cardiac output by about 100% above normal. Conversely, maximal parasympathetic nervous system stimulation decreases ventricular contractile strength only by about 30%, as the vagal innervation is sparse in the ventricles. Strong stimulation of the parasympathetic system can result in a period of asystole, followed by an escape rhythm between 20 and 40 beats per minute.12,18
Hormonal Control
Many hormones can have direct and indirect action on the heart during normal or pathophysiologic conditions. These hormones are produced within cardiomyocytes or by other tissues in the body. The hormones secreted by cardiomyocytes include natriuretic peptide, adrenomedullin, aldosterone, and angiotensin II.19–21 Atrial (ANP) and B-type natriuretic protein (BNP) are released from atria and ventricle in response to increased stretch of the chamber wall. Both ANP and BNP participate in homeostasis of body fluids, in regulation of blood pressure, and in growth and development of cardiac tissue. In chronic heart failure, high ANP and BNP levels have been associated with increased mortality.19 Adrenomedullin is a peptide hormone that increases level of cyclic adenosine monophosphate (cAMP) and has positive inotropic and chronotropic effects on the heart and is a vasodilator. The renin-angiotensin system is an important regulator of the cardiovascular system. Angiotensin II stimulates AT1 receptors with positive inotropic and chronotropic effects. It also mediates cell growth and proliferation of cardiomyocytes, thus playing an important role in development of remodeling during cardiac hypertrophy and heart failure.21
Cardiac Cycle
Electrical and Mechanical Events
The cardiac cycle is a series of coordinated electromechanical events that result in the ejection of blood from the heart into the great vessels. Ventricular systole is defined as the period of myocardial contraction when the aortic and pulmonic valves are open and diastole as the period of relaxation and ventricular filling when the mitral and tricuspid valves are open. Each mechanical event is preceded by an electrical depolarization that generates an action potential and subsequent contraction.
Carl J. Wiggers22 elegantly illustrated the mechanical, electrical, and acoustic events of the cardiac cycle. His diagram depicts the aortic, ventricular, and atrial pressure tracings with concomitant ECG, ventricular volume, and auscultatory findings22 (Fig. 15-15). Systole begins with isometric contraction (A to C), followed by opening of the aortic valve and ejection of blood into the aorta, with a period of maximum ejection (C to D) and reduced ejection (D to F). Isometric contraction starts with closure of the mitral valve and ends with opening of the aortic valve, during which time no volume enters or leaves the ventricle. Approximately two-thirds of stroke volume is ejected during the period of maximum ejection and one-third during the period of reduced ejection. The ventricular volume curve that coincides with this event is inversely related to the aortic and ventricular pressure curves. Simultaneous electrical depolarization corresponds with the QRS complex of the ECG.

Diastole follows systole and is divided into prodiastole (F and G), isometric relaxation (G and H), rapid ventricular filling (H and I), diastasis (I and J), and filling by atrial contraction (J and K). During prodiastole, the semilunar, aortic, and pulmonic valves close. This is followed by isometric relaxation, the time between closure of the aortic valve and opening of the mitral valve. Ventricular filling depends on both the relaxation of the myocardium and chamber compliance.23 There is a period of diastasis with minimal flow during which the ventricular volume remains relatively constant. This is followed by atrial contraction during late diastole, synchronous with the P wave of atrial depolarization on the ECG.
The atrial pressure tracing, or central venous waveform, begins with an “a” wave that corresponds to atrial contraction at end diastole. The “c” wave represents ventricular systole during which time the right atrial pressure increases slightly as the right ventricle contracts against a closed tricuspid valve. The subsequent downward slope in the waveform, the “x descent,” corresponds to atrial relaxation. The “v” wave occurs during venous filling of the right atria towards the end of systole. The “y descent” represents a fall in right atrial pressures as the tricuspid valve opens and the right ventricle fills in diastole24 (Fig. 15-16).


Full access? Get Clinical Tree
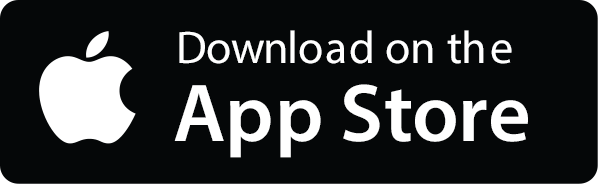
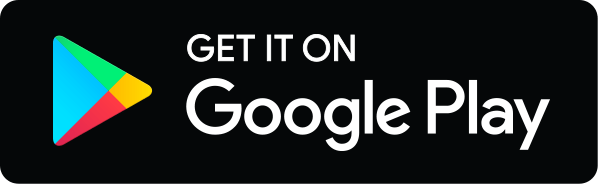