EMS medical care provided to cardiac arrest patients has changed dramatically over time. Ambulances initially functioned merely as transport vehicles, while today EMS is an integrated part of the health care system with the ability to provide advanced life support while en route to a hospital. Regional dispatch centers now decide which resources should respond to an emergency call. Dispatchers, trained in emergency medical dispatch (EMD) techniques, have specific protocols to follow to determine whether basic life support (BLS) or advanced life support (ALS) EMS response is required. Prearrival instructions to bystanders can help expedite initial first aid or CPR.1–4 Recently protocols have expanded the role of EMS providers in treating out-of-hospital cardiac arrest (OCHA) patients ranging from beginning therapeutic hypothermia in the field to bypassing hospitals in favor of a specialized receiving facility.
Historically, much of EMS medical care grew out of traditional practice with little scientific basis. Today EMS practice is influenced by evidence-based medicine concepts as more rigorous studies are completed and systems demand proven benefit prior to the introduction of new procedures, drugs, and adjuncts in prehospital patient care. This is particularly true with OHCA patients where ongoing research is continually investigating the best treatment options. The American Heart Association (AHA) periodically scientifically reviews recent literature and makes recommendations for treatment changes based on the strength and results of these studies. Prehospital resuscitations guidelines often follow the AHA recommendations closely due to the rigorous scientific process utilized in their updates. The most recent AHA update occurred in 2010, and many of the studies and papers reviewed for the most recent guidelines are discussed in this chapter.
The Framingham Heart Study reports that from 1950 to 1999, 48% of sudden cardiac deaths (SCD) and 20% of nonsudden coronary heart disease deaths occurred in patients without previously known coronary artery disease.5 Rea et al reported that from 1986 to 1994, the overall incidence of cardiac arrest was 1.89 per 1000 subject years, further breaking this down to an incidence of 0.7, 1.91, and 4.1 per 1000 subject years for the age ranges of 50 to 59, 60 to 69, and 70 to 79, respectively. The incidence of cardiac arrest in males was 2.89 compared to 1.04 for females. Compared to other risk factors, a diagnosis of congestive heart failure carried a cardiac arrest incidence of 21.87 per 1000 subject years, while a history of diabetes mellitus, previous MI, smoking, and hypertension produced incidences of 13.8, 13.69, 9.18, and 7.54, respectively.6 Cardiac arrests, along with myocardial infarction and unstable angina complaints, appear to have a circadian variation with a morning peak shortly after the initiation of daily activities.7 Lower socioeconomic status is associated with higher incidences of OHCA with a 30% to 80% difference between the highest and lowest socioeconomic quartiles. However, this difference tends to equilibrate in those over 65 years.8 Another study demonstrated racial differences in OHCA rates with black Americans having a much higher frequency than white or Hispanic Americans.9
Initial presenting rhythm in OHCA has been reported to be ventricular tachycardia, ventricular fibrillation, or a shockable rhythm as determined by an AED in approximately 23% of patients. Another 9% had nonshockable rhythms per an AED, 40% had asystole, 20% had PEA, and the final 9% were unknown or not determined. Looking at multiple American and Canadian cities when examined regionally, there appears to be a wide variation in rates of OHCA ranging from 71.8 per 100,000 subject years in Ottawa, Canada, to 159 in Dallas, Texas. Oddly though, variations are often not consistent within geographical regions such as Seattle, Washington’s 144 per 100,000 subject years compared to Portland, Oregon, and Vancouver, Canada’s 77.5 and 75.9, respectively. Taken together, the overall incidence of OHCA is calculated to be 95 per 100,000 subject years.10
Rates of witnessed OHCA and bystander CPR vary widely among localities. Studies in Arizona and New York have reported a 45.1% to 46% incidence of witnessed arrests, respectively.11,12 A meta-analysis of 79 studies involving 142,740 patients showed 53% of OHCA were witnessed by a bystander with 32% of patients receiving bystander CPR.13 However, other studies have reported bystander CPR rates as low as 19%.10 Some of these differences may be due to OHCA location (eg, home, public place, medical facility), but factors such as race and socioeconomic status are important as well. In Los Angeles, only 13% of Black or Latino patients received bystander CPR compared to 24% of white patients.14 In Arizona, non-Hispanics are more likely to received bystander CPR relative to Hispanics (41.5% vs 32.2%; P < 0.0001).15 Higher socioeconomic groups tend to have higher rates of bystander CPR.16,17 Knowledge of these disparities can help target certain groups for public education programs as well as strategically placing EMS response units in certain areas to minimize response times.
The EMS system must be activated for appropriate treatment. Thus, the patient or a bystander must recognize the problem.18–20 Delays are often frequent as presentations are varied and denial of symptoms can delay summoning help. If a sudden cardiac arrest is witnessed by a bystander or occurs in a public area, EMS is accessed faster than in an unwitnessed event.21 Arrests outside the home are twice as likely to get bystander CPR compared with residential arrests.22 A Swedish study reported that a delay of less than 4 minutes from time of cardiac arrest to EMS activation resulted in an increased 1-month survival of 6.9% as opposed to 2.8% (P < 0.0001).23 Quicker EMS response times24 and shorter delays to CPR initiation increase overall survival. These items can be extrapolated to traumatic cardiac arrests as well.
Delays in recognition and access of care vary in precardiac arrest presentations. One of the largest randomized trials to date addressing delays in treatment to patients experiencing chest pain is the European Myocardial Infarction Project (EMIP).25 Data were collected from 15 European countries and Canada between 1988 and 1992. This study found the longest delays occurred in female patients, those over age 65 years, those who had experienced chest pain in the previous 24 hours, and those with pulmonary edema. Characteristics of patients with the shortest delay in summoning an ambulance included those with a history of previous myocardial infarction (MI), those in shock, and those experiencing ventricular fibrillation. Numerous smaller studies have also reported similar findings, with the addition of lower socioeconomic status, family member being present at symptom onset, and a belief that symptoms are not severe enough also causing a delay in access of care. Gender differences are not as well defined as some studies point toward increased frequency of atypical symptoms in females, causing a larger delay between symptom onset and access of care,26 whereas others show no gender difference.27
Some populations can present without chest pain at all. Elderly and diabetic patients can have atypical presentations of acute MI with nonspecific symptoms such as dizziness, syncope, malaise, nausea, vomiting, or abdominal pain. Patients experiencing a significant event can also be in congestive heart failure, hypotensive, or in severe respiratory distress. Depending on the significance to the patient, this can cause delays in access of care or even mislead health care personnel in the wrong direction, causing another delay to proper diagnosis and treatment.
A subset of trauma patients can also decline into cardiac arrest. The mechanism of injury can range from blunt injury in car accidents to penetrating insults from stabbings or gunshot wounds. The arrest may be as a result of the traumatic injury or secondary producing a myocardial infarct due to stress. The time to access EMS care varies considerably based on the circumstances surrounding the traumatic event, the ability of the patient to initiate calling for help, and the presence of witnesses willing to intervene.
Public awareness campaigns have been shown to increase bystander CPR rates and recognition of serious symptoms. Frequent public service announcements to inform the public should continue to be encouraged, emphasizing the benefits of bystander CPR, rapid EMS activation, and the importance that time plays in a patient’s outcome.28
Calling 9-1-1 enters the caller into the system by accessing a public safety answering point (PSAP). Trained dispatchers using prearrival instructions not only dispatch EMS and first responders expeditiously, but also give explicit instructions to bystanders to render care prior to arrival of help. Outcomes are better when dispatchers receive more frequent cardiac arrest calls and when proper units are dispatched faster.29 Recognition of cardiac arrest is faster when dispatchers query callers regarding the absence of consciousness and quality of breathing.30 However, any sign of breathing, including agonal breathing, decreases the chances a dispatcher will recognize a cardiac arrest.31 Teaching dispatchers to recognize agonal breathing increases the detection of cardiac arrests,32 and also the frequency with which CPR instructions are given.33 Computer systems have been developed to assist dispatchers in detection of these events. The Medical Priority Dispatch System (MPDS) in Melbourne, Australia, was shown to have a sensitivity of 76.7% and a specificity of 99.2% at detecting a cardiac arrest.34 Likewise, the Advanced Medical Priority Dispatch System (AMPDS) in London increased the number of people accurately identified as being in cardiac arrest compared to dispatchers alone.30 While it is clear that these computer systems are helpful, they do miss patients, and there is still room to improve in dispatcher detection of cardiac arrests.
Once a cardiac arrest is identified, a dispatcher can give CPR instructions to a bystander until further help can arrive. Telephone instructions have been shown to increase the rates of bystander CPR35 and improve outcomes.29 Using simulation, volunteers who have never been trained to do CPR do compressions just as well with phone instructions as a previously trained person without directions. In any event, any compressions are better than no compressions. Only 2% of witnesses to a cardiac arrest given telephone instructions refuse to do CPR31 with reasons attributed equally to a person not wanting to do harm to a patient, fear of performing CPR improperly, concern regarding legal consequences, aversion to mouth-to-mouth contact, and being physically unable to perform CPR.36 When giving instructions, directions to put the phone down during chest compressions do not improve CPR quality.37 With the advent of cell phones with video streaming capabilities, research in the use of video calls to help dispatchers is currently underway. Dispatchers feel this may help them better assess patients and direct bystanders, but to date there is no evidence supporting the use of this technology.38
The elderly have slightly faster times to CPR initiation if given hands-only instructions (ie, no rescue breaths) rather than standard CPR instructions.39 Given the possibility that compressions-only CPR (COCPR) could increase outcomes, two recent articles in the New England Journal of Medicine compared this new technique to standard CPR with breaths. In one study, there was a trend toward increased rates of hospital discharge with COCPR in the subgroups with a cardiac cause of arrest (15.5% vs 12.3%; P = 0.09) and a shockable rhythm (31.9% vs 25.7%; P = 0.09), but there was no statistical difference between the two groups.40 In the other study, there was a nonstatistically different trend in hospital discharge (19.1% vs 14.7%; P = 0.16) but no change in 30-day mortality (8.7% vs 7.0%; P = 0.26).41 Given the relative ease of guiding someone through these steps, the lack of apparent harm, and the possibility of benefit in giving compressions-only CPR, the American Heart Association in their 2010 guidelines recommends COCPR for untrained laypersons and trained laypersons if they are unable to deliver breathing maneuvers. However, they note that at the present time there is insufficient evidence to support or refute COCPR for trained professional rescuers.42
Because of the time sensitivity to first defibrillation, various methods have been developed to decrease the time to defibrillation in a patient with a shockable rhythm. Development of automated external defibrillators (AEDs) has given the lay public access (public access defibrillation [PAD]) to easy-to-use machine that can perform this lifesaving intervention.43–45 Strategically placed in public locations, they can facilitate faster defibrillation of the arresting patient.46–48 Additionally, law enforcement officers, firefighters, and other first responders often carry AEDs. Approximately 80% of police departments are used as first responders to medical events and of these 39% carry AEDs.49
Some EMS agencies have increased overall outcomes using a two-tiered response system in which BLS first responders, with or without defibrillation capabilities, are dispatched at the same time as ALS units. Because there are often more BLS-trained providers in a given region, including firefighters and police, an EMS system can strategically place BLS first responders to minimize response times in a given area to begin care while ALS resources are en route. In one meta-analysis, survival to discharge was greater in two-tiered systems (10.5% vs 5.2%) relative to one-tiered systems.50 Another study found no statistical difference in outcomes when a BLS squad with defibrillation capabilities arrived prior to ALS. This study reported survival to admission decreased from 26.8% to 19.6% but survival to discharge increased from 8.0% to 8.7%.51 Cost analysis shows a trend to a two-tiered system being more cost-effective.52 This study reported that to improve mean response times by 48 seconds through adding more EMS providers, a one-tiered system cost would be $368,000 per quality-adjusted life year (QALY), while a two-tiered system cost would be $53,000 or $159,000/QALY. Changing from a one-tiered to a two-tiered system also has costs with one study reporting $40,000 or $94,000/QALY for pump vehicles or ambulances, respectively.52 With possible financial and patient outcome benefits, two-tiered EMS systems seem appealing. However, there is no definitive evidence favoring one system over the other. Two-tiered systems if functioning appropriately should at the very least allow for quicker response times and faster times to intervention which are two core measurements of system performance.
In 1979, Eisenberg et al published the landmark paper that defined the 8-minute response interval for cardiac arrest. In this Seattle-based study, if CPR was initiated within 4 minutes and if definitive care was provided within 8 minutes, a survival to discharge rate of 43% among witnessed cardiac arrests patients resulted. Definitive care was defined as defibrillation, intubation, or emergency medication given by paramedics or hospital personnel.53 From that point forward everyone strove to achieve similar survival rates and thus began the 8-minute response time as a national benchmark of system performance. It is important to remember that this study was done in a selected group of patients (ie, witnessed cardiac arrests), and when paramedic response times were investigated in an unselected population there were benefits if response times were less than 4 minutes, but there was no benefit when times were modeled as a continuous variable or dichotomized at an 8-minute mark.54 A Scottish study calculated that if response times were decreased from 14 minutes to under 8 minutes or 4 minutes, predicted survival would increase from 6% to 8% or 10% to 11%, respectively.55
The race to beat the clock still continues as people try to find novel ways to shave minutes off response times. Compared to using standard maps, GPS units decrease response times from 14.6 to 13.5 minutes.56 The use of geospatial time analysis can also help strategically place EMS units to decrease overall response times from a median 10.1 to 7.1 minutes.57 Urban EMS systems have reported that lights and sirens on average reduce response times by 1 minute 46 seconds.58 However, getting to the scene is only half the problem in urban areas. In New York City 28% of the actual response time was the interval from scene arrival to actually reaching the patient. This interval appears to be able to be decreased by having the dispatcher instruct the caller to send someone as an escort for EMS if the situation allows.59
The American Heart Association resuscitation guidelines are the basis for treatment of OHCA. The newest 2010 revisions of the guidelines recommend the following treatment regimens.60 Once the OHCA patient is reached CPR should be immediately begun and continued while the cardiac rhythm is analyzed using an AED or ECG monitor.61 The patient should be classified into either a shockable rhythm (ie, ventricular fibrillation or tachycardia) or a nonshockable rhythm (ie, PEA or asystole). If the rhythm is shockable, a single shock should be administered and CPR restarted immediately and continued for 2 minutes prior to another pulse check. If the patient remains in a shockable rhythm, the cycle may be repeated. Drugs may be administered to help increase the odds of a successful resuscitation. Epinephrine 1 mg and amiodarone 300 mg may be given intravenously every 4 to 5 minutes. The first or second dose of epinephrine may be replaced by 40 units of vasopressin if desired. If the rhythm is nonshockable, CPR should be continued with pulse checks every 2 minutes. Epinephrine 1 mg may be given every 4 to 5 minutes, but Atropine is no longer recommended. If the rhythm is PEA/asystole, one should search for a reversible cause. They are easily remembered by the “Hs and Ts” shown in Box 33-1. A definitive airway may be necessary for prolonged resuscitations but at the current time there is not enough data to support a specific timing or type of airway.62

Full access? Get Clinical Tree
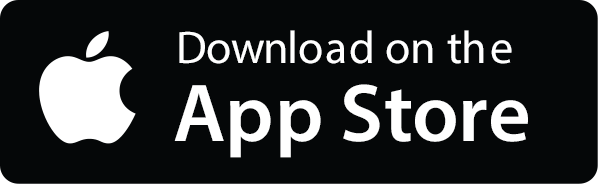
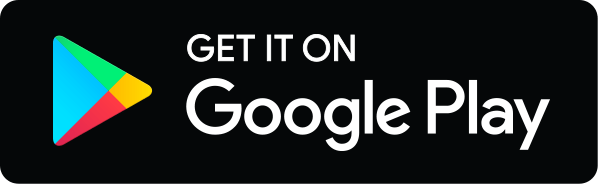