Heart rate
Colloquial
Pulse rate, heartbeats per minute
Clinical
Periodicity or frequency of contraction
Fundamental
Intactness of nodal function and innervation
Monitoring methods
ECG, pulse waveforms
Rhythm
Colloquial
Beat pattern, ECG tracing
Clinical
Regularity of contraction
Fundamental
Intactness of cardiac conduction system
Monitoring methods
Peripheral pulse, ECG, pulse waveforms
Contractility
Colloquial
Heart function, ejection fraction
Clinical
Magnitude of contraction, change in pressure
Fundamental
Increase in intraventricular pressure during contraction, change in myocyte length
Monitoring methods
TEE, pulse pressure, cardiac contractions on surgical field
Preload
Colloquial
Ventricular volume, dilation, volume status
Clinical
Chamber volume at end diastole
Fundamental
Maximum myocyte stretch
Monitoring methods
TEE, venous distension, distension of heart on surgical field
Afterload
Colloquial
Arterial squeeze, vascular tightness
Clinical
Resistance faced by myocardium
Fundamental
Work performed by myocyte
Monitoring methods
PA catheter, TEE (by excluding other causes of hypotension)

However, at high heart rates, changes in the shape of the arterial pulse pressure curve cause MAP to approach the mean of SBP and DBP. Systemic blood pressure depends on a contribution from the heart, cardiac output (CO), and a contribution from the systemic vasculature, systemic vascular resistance (SVR):


Cardiac Output
Cardiac output is the volume of blood pumped by the heart into the peripheral circulation every minute. Normal CO is approximately 5–6 L/min in a 70 kg adult male. Cardiac index (CI), equal to CO divided by body surface area (BSA), is a normalized value that allows comparison of CO among people of differing body habitus (normal CI = 2.5–4.2 L/min/m2). CO is normally identical between the right and left sides of the heart, but certain congenital abnormalities and traumatic injuries can cause the two sides of the heart to eject different amounts of blood per cardiac cycle. CO is equal to the product of heart rate (HR) and stroke volume (SV):




Heart Rate
Heart rate represents the periodic impulses from the native pacemaker function of the heart’s conduction system. Spontaneous, rhythmic depolarization of cells in the sinoatrial (SA) node generates impulses that are conducted through the atrioventricular (AV), the bundle of His, and the network of Purkinje fibers in the ventricles, thus spurring a coordinated cardiac contraction (Fig. 26.1). The spontaneous nodal function of the heart is modulated by the autonomic nervous system. Sympathetic stimulation (β-receptors) from upper thoracic spinal nerves increases HR, while parasympathetic stimulation (cholinergic receptors) from the vagus nerve (cranial nerve X) decreases HR. A mild reduction in HR can improve CO by providing more diastolic time for greater ventricular filling, but more significant decreases in HR will lead to a decrease in CO.


Fig. 26.1
Conduction system of the heart
Heart Rhythm
While heart rate measures the periodicity or frequency of cardiac contraction, rhythm measures the regularity or pattern of contraction. Abnormal conduction leads to irregular heart rhythms, or arrhythmias. Irregular rhythms can decrease CO by reducing diastolic filling time or by impeding the ability of the heart to contract in an efficient, coordinated fashion. Overall, then, HR represents the intactness of nodal function and autonomic innervation of the heart, while rhythm represents the intactness of the cardiac conduction system.
Stroke Volume
Stroke volume is the net amount of blood ejected by the heart per cardiac cycle, equal to the difference between end-diastolic volume (EDV) and end-systolic volume (ESV). During systolic contraction, shortening of cardiac myocytes generates a force that increases pressure inside the left ventricle. Once this pressure exceeds DBP, the aortic valve opens, allowing ejection of blood from the left ventricle into the aorta. The force of this myocardial contraction is called contractility. The percentage of ventricular blood volume that is ejected during a single contraction, an indirect yet clinically useful measure of contractility, is called the ejection fraction (EF). Unlike SV, EF does not change with body habitus. Healthy individuals typically have an EF of 55–70 %. Stroke volume is affected by preload, afterload, contractility, valvular dysfunction, and wall-motion abnormalities.


Preload
EDV (or preload) is the maximum volume of the heart during the cardiac cycle. It is the point at which the myocardium is maximally stretched prior to contraction and sarcomeres in the cardiac myocytes are the longest. The amount of muscle stretch in the myocardium at EDV is called preload. Surrogate measures of preload include central venous pressure (CVP), pulmonary capillary wedge pressure (PCWP), and left atrial pressure (LAP). According to the Frank–Starling mechanism, small increases in preload can improve the contractile function of the heart, resulting in increased SV with relatively little change in EF (Fig. 26.2). Preload is dependent upon venous return, the blood volume, and the distribution of blood volume (posture, intrathoracic pressure).


Fig. 26.2
Relationship between stroke volume and end-diastolic volume (Frank–Starling law)
This is appreciated clinically as a “volume responsive” heart, a situation in which volume administration improves forward blood flow and systemic blood pressure. As intraventricular volume increases further, additional increases in preload cause smaller increases in stroke volume. Changes in ventricular compliance affect the end-diastolic pressure (EDP). A poorly compliant (“stiff”) ventricle will not expand easily with increased preload, leading to increased EDP and potentially detrimental venous congestion. On the other hand, in a very compliant ventricle, as in a patient with dilated cardiomyopathy, increases in preload do not lead to appreciable increases in EDP and may fail to improve SV adequately.
Afterload
The resistance that must be overcome by the ventricle with each contraction is called afterload. On a fundamental level, afterload is the work performed by the myocardium, or the force the myocardium must generate to propel blood a certain distance. CO is inversely related to afterload. Clinically, SVR is the principal determinant of afterload (Fig. 26.3). SVR (normal 900–1,500 dyn/s/cm5) can be calculated from other hemodynamic measurements:



Fig. 26.3
Relationship between stroke volume and afterload

According to the Hagen–Poiseuille law, laminar blood flow through vessels is inversely proportional to the fourth power of vessel radius. Although capillaries are the narrowest vessels in the entire circulation, the presence of millions of capillaries in parallel minimizes their aggregate contribution to SVR. Instead, the compliance of large arterioles plays the largest role in determining ventricular afterload.
Coronary Circulation
The heart is supplied by two coronary arteries, left and right, arising from the aorta (Figs. 26.4 and 26.5). They run on the surface of the heart and are, therefore, called epicardial arteries. The right coronary artery (RCA) branches into the right marginal artery and the posterior descending artery and supplies the right atrium, right ventricle, bottom portion of both ventricles, and back of the septum. The left main coronary artery (LCA) branches into the circumflex artery and the left anterior descending artery (LAD) and supplies:



Fig. 26.4
Blood flow through the heart

Fig. 26.5
Coronary circulation
Circumflex artery (CA)—supplies blood to the left atrium and side and back of the left ventricle
Left anterior descending artery (LAD)—supplies the front and bottom of the left ventricle and the front of the septum
In people in whom the posterior descending artery arises from the RCA are right dominant (65 %), from the CA are left dominant (25 %), and both from the RCA and CA are codominant (10 %). Deoxygenated blood is returned to the chambers of the heart via coronary veins. These veins converge to form the coronary venous sinus, which in turn drains into the right ventricle. The anatomic region of heart most likely associated with the specific coronary arterial supply is:
Inferior-Right coronary artery
Anteroseptal-Left anterior descending artery
Anteroapical-Left anterior descending (distal) artery
Anterolateral-Circumflex artery
Posterior-Right coronary artery
The two coronary arteries are end arteries, and because they are narrow are prone to atherosclerosis. Average coronary blood flow is 250 ml/min. Myocardial blood flow is closely linked with oxygen demand, which is about 8–10 ml of O2/min/100 g. The myocardium extracts about 65 % of oxygen in the arterial blood compared with other tissues (25 %). The coronary arteries autoregulate coronary blood flow between perfusion pressures of 50–120 mmHg.
Increases in heart rate cause decreased coronary perfusion. This is because the heart gets its blood supply during diastole, and any increase in heart rate decreases diastolic time. Coronary perfusion pressure (CPP) is a balance between the diastolic blood pressure and the left ventricular end-diastolic pressure and can be calculated as:
CPP = Diastolic blood pressure – LVEnd diastolic pressure
Preoperative Management
Patient Assessment
Typically in elective cardiac surgery, and even in many emergency cases, the surgical diagnosis and operative plan have been established in advance by history, physical examination, and diagnostic testing. The patient presenting for heart surgery, by definition, has compromised cardiopulmonary function and has probably already suffered some degree of damage to other organs. The fundamental paradox of cardiac surgery is that the planned operation increases the risk of further damage to other organ systems, yet the operation itself presumably represents the best chance to “optimize” the patient’s overall condition. The substantial logistical and economic resources called upon by a cardiac operation impose additional pressure to develop a perioperative risk management strategy without postponing or canceling surgery.
Therefore, the goal of preoperative evaluation should be to clarify any preexisting conditions known to be associated with an increased risk of perioperative morbidity and mortality. Among these are:
Age greater than 60 years
Previous cardiac surgery
Significant obesity (body mass index greater than 35 kg/m2)
Systemic or pulmonary arterial hypertension
Acute coronary syndrome (ACS)
Congestive heart failure (CHF)
Diabetes mellitus
Peripheral vascular disease
Acute or chronic renal insufficiency
Chronic pulmonary disease
Neurological disease
History and Physical Examination
As with any other procedure, preoperative assessment for cardiac surgery begins with a careful history and physical examination. The patient should be asked about any past or current symptoms of chest pain, fatigue, shortness of breath, orthopnea, nocturnal angina or dyspnea, light-headedness, syncope, or palpitations. The time course and progression of symptoms should be determined, with particular emphasis on whether symptoms occur at rest or with exertion. It can be especially illuminating to identify symptoms in the context of the patient’s baseline lifestyle and level of activity. For example, a patient may struggle to identify symptoms in unambiguous clinical terms but may easily describe related lifestyle changes, such as a reduced capacity to perform required job duties or abandonment of a favorite recreational activity.
Physical examination should obviously include auscultation of the heart for rhythm and the presence of any murmurs. Consultation with the primary physician or cardiologist can help delineate the progression of valvular lesions and decide if further evaluation is needed. Softer midsystolic murmurs (grade 2 or lower) that are asymptomatic and are not associated with other findings are generally thought to reflect increased flow velocity and require no further workup. However, echocardiography is recommended in patients with louder or symptomatic midsystolic murmurs. Other systolic murmurs, diastolic murmurs, and continuous murmurs reflect pathology and require echocardiography.
The degree of CHF should be assessed in terms of both American Heart Association (AHA) objective criteria as well as New York Heart Association (NYHA) functional capacity (Table 26.2). The patient’s tolerated level of exertion, measured in metabolic equivalents (MET), can provide a relative measure of perioperative risk (Table 26.3).
Table 26.2
New York Heart Association (NYHA) functional classification, and American Heart Association (AHA) objective assessment of heart function
NYHA class | Functional capacity in patients with cardiac disease |
---|---|
I | No symptoms and no limitation of physical activity (ordinary physical activity does not cause undue fatigue, palpitation, dyspnea, or angina) |
II | Mild symptoms and slight limitation of physical activity (comfortable at rest, ordinary physical activity results in fatigue, palpitation, dyspnea, or angina) |
III | Moderate symptoms and marked limitation of physical activity (comfortable at rest, less-than-ordinary activity causes fatigue, palpitation, dyspnea, or angina) |
IV | Severe symptoms and severe limitation of physical activity (inability to carry on any physical activity without discomfort, symptoms of heart failure or angina may be present even at rest, bed-bound patients) |
AHA class | Objective assessment |
A | No objective evidence of cardiovascular disease |
B | Objective evidence of minimal cardiovascular disease |
C | Objective evidence of moderately severe cardiovascular disease |
D | Objective evidence of severe cardiovascular disease |
Table 26.3
Approximate metabolic equivalents of task (MET) for various activities. One MET represents metabolic oxygen consumption of 3.5 ml kg−1 min−1
MET | Functional status | Activity |
---|---|---|
<4 | Poor | Sleeping or sitting stationary Activities of daily living: eating, dressing, bathing, using the toilet Writing, desk work Walking indoors or around the house Light housework: changing bed sheets, dusting, washing dishes |
4–7 | Intermediate | Brisk walking 1–2 blocks on level ground Climbing 1–2 flights of stairs or walking uphill Gardening and lawn work: raking leaves, weeding, pushing a mower Sexual relations Moderate housework: vacuuming, sweeping floors, carrying groceries |
>7 | Good | Heavy housework: scrubbing floors, lifting and moving heavy furniture Jogging or running Swimming, cycling, vigorous sports |
Having cardiac surgery is a major life event by any measure, and caregivers need to be sensitive to the immense emotional burden faced by patients and their loved ones. In the preoperative period, the anesthesiologist must balance the desire for a thorough assessment and honest discussion of perioperative risks with the need to avoid placing undue psychological (and, in turn, physiologic) stress on the patient. A candid explanation of the anesthesia team’s active role in the operating room—monitoring the patient continuously and providing the diagnostic and physiologic support necessary to allow the surgeon the freedom to concentrate on the technical aspects of the operation itself—can be both informative and reassuring. At the same time, many patients view the prospect of heart surgery as a signal to reconsider their own health-related behaviors and commit to improving them afterwards. The preoperative discussion offers a valuable opportunity for the anesthesiologist to reinforce this process by encouraging healthy lifestyle changes that will also reduce future anesthetic risk, such as smoking cessation and weight management.
Concomitant Diseases
Patients scheduled for cardiac surgery frequently present with multiple comorbid conditions, which may arise either independently or as a result of their compromised cardiac status. Reviewing the patient’s list of prescription and over-the-counter medications can quickly reveal coexisting conditions that should be considered when developing a perioperative management plan.
Cardiovascular
Coronary artery disease often occurs in concert with cerebrovascular and peripheral vascular disease. Any history of previous stroke or transient ischemic attack, along with any residual neurologic defects, should be ascertained. Auscultation of the carotid arteries for bruits and review of any carotid Doppler studies can reveal the severity of occlusive disease, which increases the risk of perioperative cerebrovascular complications. Claudication, paresthesias, and venostasis changes suggest the presence of significant peripheral vascular disease. Peripheral pulses should be palpated, particularly in locations where arterial line placement is anticipated.
Pulmonary
The thoracotomy incision incumbent with cardiac surgery, as well as CPB itself, increases the risk of postsurgical pulmonary complications. Thoroughly assessing the patient’s baseline pulmonary status can help predict the need for prolonged postoperative ventilation. Important considerations in patients with asthma or other chronic obstructive pulmonary disease (COPD) include the frequency of symptoms, time since the last attack, compliance with medications, and any previous need for intubation. Preoperative oxygen saturations, blood gases, pulmonary function tests, and chest imaging can be useful. Any history of smoking, current or remote, should be elicited. Even if undiagnosed, some degree of obstructive sleep apnea can be presumed in morbidly obese patients or those who present with snoring or daytime somnolence. Any use of supplemental oxygen or positive airway pressure therapy should be determined to help guide intraoperative and postoperative ventilation strategies.
Airway
If the physical examination suggests a difficult airway, and especially if an awake intubation is anticipated, preparations should be made for adequate topicalization, sedation, and antihypertensive therapy during intubation. If there is evidence of poor dentition or abscesses in a patient scheduled for valve surgery, preoperative dental consultation and tooth extraction may be indicated to prevent the development of prosthetic valve endocarditis.
Diabetes Mellitus
Diabetes mellitus is a major risk factor for coronary artery disease and an independent predictor of perioperative morbidity and mortality. Because of accompanying autonomic neuropathy, diabetics have an increased risk of hemodynamic lability and asymptomatic (silent) myocardial ischemia, thereby increasing overall cardiovascular risk. Delayed gastric emptying, also associated with autonomic neuropathy, can also complicate airway management. A preoperative serum glycosylated hemoglobin (HbA1c) level can help characterize the quality of glycemic control in the months preceding surgery and identify those patients in need of more aggressive perioperative and postoperative glycemic control.
Renal
Patients with even early stages of renal dysfunction experience increased morbidity from cardiac surgery. Many factors incumbent to cardiac surgery, such as large crystalloid fluid loads from CPB, hyperkalemic cardioplegia solutions, and variable or prolonged periods of systemic hypoperfusion, can adversely affect renal function. Severe renal impairment, especially when combined with anemia and metabolic acidosis, can compromise myocardial function when weaning the patient from CPB. Baseline urine production in patients with renal dysfunction should be assessed, as urine output is often used in cardiac surgery as an indicator of renal function.
Liver
Severe liver dysfunction increases the risk of severe bleeding complications from surgery. Any clinical signs of impaired clotting, such as delayed wound healing, epistaxis, or gum bleeding, should raise concern about severely reduced production of clotting factors. Preoperative administration of vitamin K or fresh frozen plasma may be warranted, keeping in mind that the added fluid load can worsen CHF and left ventricular dysfunction. Elective surgery should be delayed in patients with acute hepatitis until serum liver function tests normalize.
Laboratory Tests
Reviewing the cardiac surgical assessment and previous diagnostic tests is essential, both to assess the patient’s overall medical condition and to understand more fully the planned operation. Previous surgical records may provide evidence of potential complicating factors, such as unanticipated difficult airway management or adverse events. Past surgical records are particularly important for repeat cardiac surgery. A chest radiograph can show the distance between the cardiac silhouette and the sternum, which can help judge the likely difficulty of sternotomy and intrathoracic surgical dissection. Other imaging modalities, such as computer tomography (CT) or cardiac magnetic resonance imaging (MRI), can delineate intrathoracic anatomy and highlight potential dangers for sternotomy, such as a dilated aortic root or previous coronary bypass grafts in close proximity or adherent to the sternum.
Important preoperative laboratory tests include serum hemoglobin and hematocrit, platelet count, blood urea nitrogen and creatinine levels, coagulation profiles, and liver function tests. The preoperative electrocardiogram (ECG) should be examined for signs of myocardial ischemia, prior myocardial infarction, and abnormal conduction. Stress echocardiography, myocardial perfusion studies, and cardiac catheterization can provide valuable information about valvular abnormalities, global and segmental left ventricular function, areas of induced ischemia, pulmonary hypertension, and right ventricular dysfunction (cor pulmonale). For patients scheduled for coronary artery bypass grafting (CABG), cardiac catheterization can define coronary anatomy and help determine the number and location of planned bypass grafts.
Preoperative Medications
Antihypertensives
Primary or essential hypertension is common in patients having cardiac surgery and is a major concern for risk assessment and stratification. Chronic hypertension can lead to left ventricular hypertrophy, decreased ventricular compliance, renal insufficiency or failure, and neurologic symptoms progressing to infarction. After excluding secondary causes of increased blood pressure (e.g., renal disease, pheochromocytoma, or certain drugs), one should assess the typical range of blood pressures within the patient normally lives without symptoms. Patients are typically advised to delay elective surgery until blood pressure is controlled to a normal range, but altered cerebral autoregulation may make normotension undesirable. Untreated primary hypertension that appears to resolve spontaneously (“pseudonormotension”) may actually represent myocardial compromise or progression of valvular stenosis and pose a risk of cardiovascular collapse with minimal anesthetic exposure or surgical stress.
In general, patients on antihypertensive medications should continue such medications throughout the perioperative period to maintain blood pressure homeostasis at the time of surgery, though diuretics should not be given the day of surgery to minimize hypovolemia. In particular, withdrawal of β-blockers and clonidine can lead to rebound hypertension. Preoperative nitrates and digoxin should also be continued. Calcium channel blockers may have renal protective effects in patients undergoing surgery involving aortic crossclamping, but their myocardial depressant and vasodilator effects can accentuate hypotension during anesthetic induction. Refractory hypotension during and after CPB can also occur with ACE inhibitors and angiotensin II receptor antagonists. Nonetheless, the apparent renal protective benefit of these agents warrants their continuation perioperatively while treating intraoperative hypotension with appropriate vasoconstrictor therapy
Antidiabetics
Diabetics undergoing cardiac surgery require serial monitoring of serum glucose levels. Patients should be instructed to withhold their usual nutritional insulin on the day of surgery. Similarly, oral diabetes medication should be held in the morning of the surgery. Inpatients awaiting surgery may require scheduled insulin therapy to achieve preoperative glycemic control.
The intraoperative humoral stress response can cause increased cortisol levels and decreased production of insulin, both of which can lead to hyperglycemia. Intraoperative glycemic control is achieved most efficiently with a continuous intravenous infusion protocol rather than intermittent intravenous boluses or subcutaneous injections. An insulin protocol should be started perioperatively for diabetic patients undergoing cardiac surgery, as well as for nondiabetics who have repeated serum glucose values ≥180 mg/dL. Maintaining glycemic control (120–180 mg/dL) prior to and during cardiac surgery is associated with reduced mortality, decreased neurologic injury, lower incidence of wound infections, and decreased length of hospital stay. Tighter glucose control strategies (such as 90–120 mg/dL) have not been demonstrated to lead to superior outcomes. While mild hyperglycemia appears to be well tolerated in most patients, hypoglycemia is an unambiguously undesirable complication of intensive insulin infusion therapy. Accordingly, overly aggressive intraoperative glucose control may be counterproductive, especially if it distracts from other patient care responsibilities.
Anticoagulants
Patients on chronic anticoagulant therapy (e.g., aspirin, heparin, or warfarin) or who have been recently exposed to thrombolytic agents pose a particular challenge. The preoperative evaluation should pay particular attention to the usage, dosage regimen, indications, and cessation intervals of these drugs. Ideally, such medications should be stopped several days prior to surgery to minimize postoperative bleeding complications, but these benefits should be weighed against the patient-specific risks of stopping ongoing anticoagulant therapy, such as in-stent restenosis or thromboembolism. Warfarin (Coumadin) should be stopped 5 days prior to surgery or until a normal or near-normal INR is reached. Similarly, PTT or thrombin clotting time can help verify adequate blood clotting function after discontinuing dabigatran (Pradaxa, a direct thrombin inhibitor) or rivaroxaban (Xarelto, a direct factor Xa inhibitor). Patients at high risk of thrombosis may need to be admitted to the hospital preoperatively for bridging therapy. More urgent surgery may require administration of some combination of vitamin K and fresh frozen plasma, depending on the patient’s level of anticoagulation and the urgency of surgery.
Aspirin irreversibly inhibits platelet cyclooxygenase, rendering platelets inactive. Thienopyridines such as clopidogrel (Plavix) and prasugrel (Effient) also irreversibly inhibit platelet response for the life of the platelet. A newer ADP receptor/P2Y12 inhibitor, ticagrelor (Brilinta), is an allosteric antagonist that provides reversible platelet blockade. Patients with newly diagnosed ACS may be started on dual antiplatelet therapy (aspirin and clopidogrel) to prevent further disease progression. One may suspect that discontinuing antiplatelet therapy would predispose the patient to thrombotic complications, particularly in patients with drug-eluting stents. However, studies suggest that discontinuing antiplatelet therapy a few days before surgery is actually associated with reductions in bleeding, transfusion requirements, and rates of reoperation, with no significant increase in rates of myocardial infarction, stroke, or postoperative death. Preoperative discontinuation of aspirin is also reasonable in high-risk patients, such as those who refuse blood transfusion (Jehovah’s Witnesses) and those with limited sources of allogeneic blood products due to antibodies.
Patients presenting for urgent or emergent cardiac surgery may have received doses of glycoprotein IIb/IIIa receptor antagonists during cardiac catheterization. Antiplatelet effects last approximately 24–48 h for abciximab (ReoPro), 4–8 h for tirofiban (Aggrastat), and 2–4 h for eptifibatide (Integrilin). Even in nonelective surgery, a delay of 1 or 2 days can help reduce intraoperative bleeding risk while minimizing thrombotic risk. Laboratory tests of platelet inhibition, such as PFA-100 or thromboelastography (TEG), can be helpful in deciding whether to delay surgery. If surgery cannot be postponed, the increased intraoperative bleeding may necessitate acute reversal of therapy, alterations in heparin dosing for CPB, large transfusions of blood products (including platelets), or administration of procoagulant agents (such as activated factor VII).
Herbals
The preoperative review of medications should not neglect over-the-counter medications, herbal remedies, nutritional supplements, and other nontraditional therapies, as they can have important implications for anesthetic care. For example, ephedra (ma huang) is a sympathomimetic compound that can complicate hemodynamic management, while ginseng and gingko biloba can inhibit platelet aggregation. Patients may be reluctant to mention taking these substances unless specifically asked about them. Because complementary therapies are not consistently regulated for origin, content, and purity, all such drugs should preferably be stopped at least 7 days prior to surgery.
Cardiac Implantable Electronic Devices
Cardiac implantable electronic devices consist of permanent pacemakers, which supplement or replace the heart’s native conduction system, and implantable cardioverter defibrillators (ICDs), which provide tachycardia therapy. Approximately three million people worldwide currently live with a pacemaker. In the United States alone, roughly one million people have a pacemaker, and nearly 200,000 new pacemakers are implanted annually. Pacemakers and ICDs are implanted for a wide variety of conduction disorders and ischemic conditions (Table 26.4). The increasingly widespread use of these devices presents special challenges for perioperative management.
Table 26.4
Indications for cardiac implantable electronic device implantation
Permanent pacemaker | Implantable cardioverter defibrillator (ICD) |
---|---|
Sinus node disease Atrioventricular node disease Long QT syndrome Hypertrophic cardiomyopathy Dilated cardiomyopathy | Ventricular tachycardia, fibrillation Post-myocardial infarction with EF ≤30 % Cardiomyopathy with EF ≤35 % Long QT syndrome Hypertrophic cardiomyopathy Awaiting ventricular assist device or heart transplant |
In addition to evaluating and optimizing coexisting conditions, preoperative evaluation of a patient with an implanted device should include determining the type of device, indication for placement, and currently programmed settings (Table 26.5). This information can frequently be obtained from the patient (wallet card) or the physician managing the device. A chest radiograph can help determine the device type by showing the number and location of pacing electrodes and shock coils. Also, the generator may also be identified by a radiopaque manufacturer logo and serial number. Locating the coronary sinus lead on a biventricular pacemaker or ICD can help avoid dislodgment during central line placement.
Table 26.5
Generic codes for cardiac implantable electronic devices
Pacemaker | ||||
Position I | Position II | Position III | Position IV | Position V |
Chambers paced | Chambers sensed | Response to sensing | Programmability | Multisite pacing |
O = None | O = None | O = None | O = None | O = None |
A = Atrium | A = Atrium | I = Inhibited | R = Rate modulation | A = Atrium |
V = Ventricle | V = Ventricle | T = Triggered | V = Ventricle | |
D = Dual (A + V) | D = Dual (A + V) | D = Dual (T + I) | D = Dual (A + V) | |
Implantable cardioverter defibrillator (ICD) | ||||
Position I | Position II | Position III | Position IV (or Pacemaker Code) | |
Shock chambers | Antitachycardia pacing chambers | Tachycardia detection | Antibradycardia pacing chambers | |
O = None | O = None | E = Electrocardiogram | O = None | |
A = Atrium | A = Atrium | H = Hemodynamic | A = Atrium | |
V = Ventricle | V = Ventricle | V = Ventricle | ||
D = Dual (A + V) | D = Dual (A + V) | D = Dual (A + V) |
Nonetheless, interrogation with a programming console remains the only reliable means of evaluating assessing device settings and predicted battery life. Under ideal circumstances, all patients with a pacemaker or ICD should undergo preoperative device interrogation, not only to determine proper function but also to facilitate proper intraoperative management by the anesthesia team. However, this may not be possible in all situations, such as in emergency surgery.
Electromagnetic interference (EMI) from surgical electrocautery can be detected by the device and interfere with its normal function. Monopolar electrocautery (Bovie) creates an arc of electrical current from the single handheld electrode to the adhesive return pad; this current can threaten any electrical device or metallic implant in its path. In contrast, bipolar electrocautery confines the current between the two handheld electrodes and is preferable in these patients. If monopolar electrocautery is required for the operation, then the return pad should be placed in a location that prevents the electrical arc from crossing the device generator and leads. All patients with ICDs should have antitachycardia therapy disabled prior to surgery with monopolar electrocautery.
The sheer variety of devices and programming modes currently available makes formulaic preoperative management difficult. For example, it is commonly assumed that a magnet will convert a pacemaker to asynchronous pacing and disable antitachycardia therapy when applied to an ICD. However, magnet effects vary significantly depending on the manufacturer, model, and even specific device settings. Even when indicated, magnet placement is an unreliable technique for changing device therapy. Obesity, perspiration, patient movement, surgical positioning, and other implanted devices can interfere with proper magnet contact; loss of contact may not be readily apparent to the clinician, as the pacing function of the device would not be changed. The anesthesia team should test the magnet’s effect prior to the start of surgery, paying close attention to whether the preprogrammed asynchronous pacing rate is sufficient, particularly in patients with compromised myocardial function.
Postoperatively, any device that was reprogrammed prior to surgery should be interrogated and reset appropriately. Most manufacturers also recommend a postoperative interrogation to confirm proper device function and adequate battery life after exposure to electrocautery or other EMI. In addition, changes in the patient’s functional status after surgery may warrant new device settings to maintain adequate cardiac output and tissue oxygen delivery.
In many centers, anesthesiologists provide perioperative care for patients undergoing pacemaker and ICD placement and other electrophysiology procedures (e.g., catheter ablation of supraventricular arrhythmias). These procedures typically involve superficial tissue dissection with local anesthetic infiltration for pocket formation or catheter placement. The majority of these cases can be performed under deep sedation with spontaneous ventilation, rather than general endotracheal anesthesia. However, specific events during the procedure, such as cryoablation or ICD test shocks, are quite painful, so adequate analgesia and amnesia should be ensured. Because these procedures can be very lengthy, providers should be vigilant for ischemia of dependent body parts, atelectasis, excessive sedation, and airway obstruction. Intravenous lidocaine, often used to reduce the burning associated with propofol administration, has antiarrhythmic effects that may interfere with planned electrophysiologic studies and should probably be avoided. An acute fall in blood pressure may indicate pericardial tamponade resulting from cardiac perforation with a catheter or device lead, a situation that may require emergent surgical intervention.
Transesophageal Echocardiography
Transesophageal echocardiography (TEE) has become an integral tool in the anesthetic management of cardiac surgical patients. Perioperative TEE allows the echocardiographer to diagnose intracardiac pathology (Table 26.6), direct the surgical procedure, and assess results and complications. In addition, TEE allows continuous intraoperative monitoring of cardiac function during cardiac and noncardiac operations. It is particularly useful for intrathoracic surgeries, during which transthoracic echocardiography would not be feasible.
Table 26.6
General indications for transesophageal echocardiography
General indication | Specific examples |
---|---|
1. Evaluation of cardiac and aortic structure and function | (a) Evaluation of prosthetic heart valves (b) Evaluation of paravalvular abscesses (c) Intubated patients (d) Patients with chest wall injuries (e) Patients with body habitus preventing adequate TTE examination |
2. Intraoperative TEE | (a) All open-heart and thoracic aortic surgical procedures (b) Major vascular procedures (c) Noncardiac surgery in patients with known cardiovascular pathology |
3. Guidance of transcatheter procedures | • Septal defect closure • Atrial appendage obliteration • Percutaneous valve replacement |
4. Critically ill patients | • TEE information is expected to alter management |
TEE employs a long probe inserted into the patient’s esophagus (Fig. 26.6). A piezoelectric crystal at the tip of the probe emits a plane of ultrasound waves that reflect off different structures in relation to their tissue densities. The probe detects and processes these reflected waves to acquire an image. The imaging plane can be rotated up to 180° without moving the probe (multiplaning), thus allowing a structure to be imaged from multiple angles. The probe tip can be flexed in different directions, and the probe itself can be rotated or moved to different positions in the esophagus (mid-esophageal and upper esophageal windows) or stomach (transgastric and deep transgastric windows). Manipulating the probe in these ways can produce a comprehensive examination of the entire heart and many other intrathoracic structures (Fig. 26.7). The ascending aorta and transverse arch are not well visualized by TEE because the left mainstem bronchus passes between the esophagus and ascending aorta, impeding penetration of ultrasound waves. Recent technological advances have produced TEE probes capable of real-time three-dimensional imaging.



Fig. 26.6
Transesophageal echocardiography with the probe in the esophagus

Fig. 26.7
Comprehensive TEE examination: various views (20)
Absolute contraindications to TEE include perforations, stricture, or masses that can interfere with or be exacerbated by probe manipulation (Table 26.7). A TEE exam can be performed in a patient with relative contraindications provided the anticipated benefits of TEE monitoring outweigh the risks. For example, in a patient with esophageal varices and bacterial endocarditis, the risk of esophageal bleeding associated with TEE probe placement may be outweighed by the anticipated benefit of assessing the patient for intracardiac vegetations or prosthetic valve dehiscence. Alternatively, in the setting of relative contraindications, TEE can be performed with appropriate modifications (e.g., avoiding transgastric windows in a patient with a subtotal gastrectomy). An epicardial or epiaortic probe can also be handed off onto the sterile surgical field to obtain supplementary images. Epiaortic echocardiography remains the most sensitive and specific modality for visualizing calcifications in the ascending aorta that may preclude cannulation.
Table 26.7
Contraindications to transesophageal echocardiography
Absolute contraindications | Relative contraindications |
---|---|
Perforated viscus | History of radiation to neck or mediastinum |
Esophageal stricture | History of GI surgery |
Esophageal tumor | Recent upper GI bleeding |
Esophageal perforation or laceration | Restricted neck mobility (severe cervical arthritis, atlantoaxial joint instability) |
Esophageal diverticulum | Esophageal varices |
Tracheoesophageal fistula | Coagulopathy or thrombocytopenia |
Active upper GI bleeding | Active esophagitis/peptic ulcer disease |
The overall complication rate of intraoperative TEE is very low (approximately 0.2 %) and can be minimized by careful patient preparation and probe manipulation (Table 26.8). Prior to inserting the TEE probe, an orogastric tube can be passed into the esophagus to determine if there are any strictures or other obstructions that would preclude safe passage of the larger TEE probe. Suctioning the orogastric tube before removing it will also evacuate stomach contents that can interfere with obtaining high-quality TEE images in the transgastric windows. To reduce the risk of esophageal rupture, all other esophageal instruments (e.g., esophageal temperature probes) should be removed before placing the TEE probe. The probe should never be forced blindly against resistance. If necessary, direct laryngoscopy and careful deflation of the endotracheal tube cuff can help facilitate probe placement. A bite block should be used to help protect the teeth and oral soft tissues from damage. The probe should be inspected and moved periodically during the operation to prevent pressure injury to the lips and gums. Inadequate rinsing of cleaning solutions from the probe can lead to chemical burns on oral soft tissues.
Table 26.8
Complications reported with transesophageal echocardiography
Esophageal bleeding |
Esophageal perforation |
Lip/Dental injury |
Tracheal probe placement or laceration |
Pharyngeal trauma or bleeding |
Endotracheal tube malposition |
Laryngospasm/Bronchospasm |
Dysphagia |
Hoarseness of voice |
Arrhythmias |
Stimulation from inserting and manipulating the TEE probe can produce adverse hypertension and tachycardia. Sufficient depth of anesthesia, analgesia, and vasoactive therapy should be ensured to avoid myocardial ischemia or heart failure from probe manipulation. Because the thoracic aorta passes close to the esophagus, large thoracic aneurysms can compress the esophagus, causing dysphagia. Probe insertion in this situation increases the risk of aortic rupture. The preoperative CT scan should be examined in advance for signs of esophageal impingement or deviation.
Perhaps the most underappreciated and dangerous consequence of intraoperative TEE is provider distraction. The eagerness to obtain optimal views and elucidate complex structures on the TEE exam can cause the operator to neglect important changes on patient monitors or the surgical field. The provider should never forget that performing a comprehensive TEE exam is only one aspect of complete anesthetic management for cardiac surgery. For this reason, it is desirable to have one anesthesia provider concentrate on monitoring and tending to the patient while another performs and interprets the TEE exam.
The National Board of Echocardiography (NBE) has developed processes by which anesthesiologists, depending on their level of training and case experience, can work toward certification in perioperative TEE. The recent introduction of basic certification affirms the value of TEE as a useful hemodynamic monitor in the noncardiac operative setting. Basic certification is intended to prepare providers, including those without specialized training in cardiac anesthesiology, to use TEE primarily for intraoperative monitoring of hemodynamic instability and guidance of inotropic and vasoactive support. Global and regional left ventricular function, right ventricular function, hypovolemia, qualitative valvular function, pulmonary embolism, air embolism, pericardial effusions, thoracic trauma, and basic septal defects can be assessed within the scope of the basic TEE examination. Advanced certification expands the scope of training to encompass complex valvular lesions, prosthetic devices, congenital defects, and other complex intrathoracic pathology. Advanced certification also prepares the provider to provide diagnostic guidance for cardiac surgical and transcatheter procedures.
Intraoperative Management
Goals and Preparation
More than in any other intraoperative settings, patients undergoing cardiac surgery have disease conditions that put them at risk of decompensating with very little warning. The overarching philosophy of cardiac perioperative care, then, is to be ready to respond to a sudden decline in the patient’s condition at any time. Anesthesia providers should always be prepared to support any necessary resuscitative maneuvers, including emergency sternotomy and CPB. It is prudent to have bolus and infusion preparations of an inotrope, a vasoconstrictor, and a vasodilator ready to use for every case, plus sufficient heparin to commence bypass (Table 26.9).
Table 26.9
Sample anesthesia setup for cardiac surgery
A. Medications: At least one medication from each category should be readily available. Syringes and infusions do not need to be prepared in advance unless noted below or indicated by the specific physiologic requirements of the patient or planned surgery. It is highly desirable to have bolus and infusion preparations of at least one inotrope, one vasopressor, and one vasodilator ready to deliver | ||
Anticholinergics | Atropine | 0.1 mg/ml syringe ready |
Glycopyrrolate | 0.2 mg/ml syringe ready | |
Inotropes | Epinephrine | 1 mg in 250 ml (4 mcg/ml), 0.01 mg/ml syringe ready |
Dopamine or Dobutamine | Prepare infusion as Dopamine—400 mg in 250 ml, Dobutamine—500 mg in 250 ml | |
Calcium chloride | 10 ml syringe ready | |
Vasopressors | Phenylephrine | Prepare infusion as 10 mg in 250 ml (40 mcg/ml) |
Norepinephrine | Prepare infusion as 8 mg in 250 ml (32 mcg/ml) | |
Vasopressin | Prepare infusion as 100 U in 100 ml | |
Inotrope/vasopressor | Ephedrine | Bolus 5–10 mg syringe ready |
Inotrope/vasodilator | Milrinone | Prepare infusion as 50 ml or mg of drug plus 200 ml = total 250 ml (200 mcg/ml), ready prepacks available |
Vasodilators | Nitroglycerin | Glass bottles available as 200 or 400 mcg/ml |
Nitroprusside | Prepare infusion as 50 mg in 250 ml (200 mcg/ml), protect from light-put opaque cover | |
Nicardipine | Prepare infusion as 10 ml of drug (25 mg) plus 240 ml = total 250 ml (0.1 mg/ml) | |
Anticoagulation for CPB | Heparin | Sufficient extra supply should be available in case patient needs to return to CPB, 300 U/kg for initiation of CPB |
Protamine | Syringe or infusion should be either stowed away until needed or prepared after weaning from CPB to prevent premature administration to patient, 1 mg for every 100 U of heparin | |
Antiarrhythmics | Lidocaine, adenosine, amiodarone, magnesium sulfate | |
Sedatives/induction agents | Midazolam, thiopental, propofol, etomidate, and/or ketamine | |
Inhaled agent (e.g., isoflurane) | ||
Analgesics/opioids | Fentanyl, sufentanil, remifentanil | |
Muscle relaxants | Succinylcholine and a nondepolarizing agent (e.g., pancuronium) | |
B. Equipment: All equipment should be checked for proper function and adequate battery power prior to surgery | ||
Anesthesia machine, monitors, and invasive pressure monitor transducers | ||
Airway equipment: standard equipment and any specialized devices (e.g., double-lumen tubes) | ||
Infusion pump(s) with multiple channels | ||
Defibrillator with external pads | ||
External pacemaker generator | ||
TEE machine and probe | ||
Patient transport equipment: portable monitor, full oxygen cylinder, and bag valve mask |
Preventing adverse hemodynamic responses to anesthetic and surgical interventions, an important goal in any operation, is especially critical in cardiac surgery (Table 26.10). Preoperative sedation and induction of general anesthesia can lead to decreases in myocardial function and peripheral vascular resistance that may be poorly tolerated in cardiac patients. Pain and increased sympathetic stimulation from incision, sternotomy, and aortic cannulation can precipitate tachycardia, hypertension, or dysrhythmias, all of which can lead to ischemia and heart failure in patients with compromised cardiac function. One useful technique is to assess preoperatively the range of vital signs within which the patient is asymptomatic, comfortable, and free of ischemia (e.g., during physical activity or stress testing), and then use this range as a goal for maintaining blood pressure and heart rate in the operating room.
Table 26.10
Common cardiovascular agents and doses
Antihypertensive agents | |
---|---|
Nitroglycerine | 0.25–10 mcg/kg/min, 1–2 ml of 20–40 mcg/ml as bolus |
Nitroprusside | 0.25–10 mcg/kg/min |
Esmolol | 0.5 mg/kg bolus over 1 min, 50–300 mcg/kg/min infusion |
Labetalol | 5–20 mg |
Metoprolol | 2–10 mg |
Hydralazine | 4–20 mg |
Phentolamine | 1–5 mg |
Nicardipine | 0.25–0.5 mg bolus, 2.5–15 mg/h infusion |
Vasopressors | |
Epinephrine | 0.001–0.1 mcg/kg/min, 2–10 mcg bolus |
Norepinephrine | 0.01–0.1 mcg/kg/min or 1–16 mcg/min |
Dobutamine | 2–20 mcg/kg/min |
Dopamine | 2–20+ mcg/kg/min |
Ephedrine | 5–25 mg bolus |
Phenylephrine | 20–60 mcg/min, 40–200 mcg bolus |
Milrinone | 0.375–0.75 mcg/kg/min, 50 mcg/kg bolus over 10 min |
Vasopressin | 0.01–0.04 U/min |
Vasodilators | |
Nitroglycerine | 0.25–10 mcg/kg/min |
Nitroprusside | 0.25–10 mcg/kg/min |
Nicardipine | 2.5–15 mg/h |
Fenoldopam | 0.03–1.0 mcg/kg/min |
Nitric oxide | 10–60 ppm (inhaled) |
Antiarrhythmic agents | |
Lidocaine | 1–2 mg/kg |
Esmolol | 0.5 mg/kg |
Metoprolol | 2–10 mg |
Amiodarone | 150 mg over 10 min, 0.5 mg/min |
Verapamil | 2.5–10 mg |
Diltiazem | 0.25–0.35 mg/kg, 3–15 mg/h |
Digoxin | 0.5–0.75 mg |
Adenosine | 6–12 mg |
Premedication
Patients about to undergo heart surgery are likely to be very anxious, but what may be an appropriate intravenous dose of midazolam or fentanyl for another patient may be excessive for a patient with compromised cardiac function. As with any surgery, clinical judgment rather than just routine practice should be used to decide upon the need for premedication prior to surgery.
Ideally, patients should not be premedicated until they arrive in a location, such as the operating room or a preoperative holding area, where trained anesthesia personnel can monitor them continuously. Slow titration is desirable to prevent large swings in blood pressure and heart rate. Judicious premedication to sedate the patient can reduce the dosage of induction drugs required to achieve general anesthesia. Special care should be taken in patients with severe heart failure or with severe or symptomatic aortic stenosis, as they may be unable to compensate adequately for even small decreases in systemic vascular resistance. Medications that can be used for premedication include midazolam, diazepam (5–10 mg PO, the night before), morphine (0.15 mg/kg) plus scopolamine 0.2–0.3 mg intramuscularly (IM), or hydromorphone 1–2 mg IM. It should be remembered that scopolamine can cause confusion in the elderly, while the combination of a benzodiazepine and an opioid can have synergistic effects, which warrant reduction in dosage.
Intraoperative Monitoring
Standard noninvasive monitors and supplemental oxygen should be applied to the patient both in the preoperative holding area and upon arrival in the operating room. Induction of general anesthesia can cause rapid, detrimental changes in blood pressure. Therefore, continuous blood pressure monitoring is highly desirable, and an arterial line should be placed prior to induction. Before attempting a radial or brachial arterial line in a patient undergoing CABG, the anesthesia provider should confirm that it will not be in the same arm as any planned radial artery graft harvest. Patients undergoing aortic surgery may require multiple arterial lines in different locations (e.g., femoral and right radial arteries) depending on where the crossclamp will be placed during surgical repair.
Central venous access is also useful prior to induction to facilitate fluid management and vasoactive infusions. In the absence of intervening pathology, CVP is equivalent to right atrial pressure and can be affected by circulating blood volume, peripheral venous tone, and right ventricular function. Placing a central line can be deferred until after induction if the patient has an existing large-bore intravenous line or is unlikely to tolerate being conscious and stationary for the procedure. However, for patients in emergency situations with adequate large-bore access, central line placement and monitoring should not delay anesthetic induction, prompt opening of the chest, surgical control of bleeding, or initiation of bypass.
Traditionally, cardiac surgery was considered an indication in itself for the placement of a pulmonary artery (PA, or Swan-Ganz) catheter. The PA catheter allows measurement of pressures in the right ventricle and pulmonary artery, transvalvular pressure gradients across the tricuspid and pulmonic valves, and calculation of systemic and pulmonary vascular resistance. These values can help assess filling pressures throughout the heart and assist in the diagnosis and treatment of right heart failure and pulmonary hypertension. Thermodilution catheters also allow measurement of cardiac output, either intermittently or continuously, and sampling of mixed venous blood to assess total body oxygen extraction.
Several recent studies have questioned the utility of routine PA catheterization, suggesting an association with worse outcomes and even increased mortality. PA catheter data can greatly assist in hemodynamic management, especially in the postoperative setting without ready access to TEE equipment or trained operators, but this data should be evaluated in the context of the patient’s overall clinical status. TEE and other less invasive methods of cardiac output monitoring can be valuable adjuncts or, in many cases, alternatives to the PA catheter. In low-risk patients with well-preserved left ventricular function undergoing CABG, the PA catheter is unlikely to provide sufficient benefit to outweigh the risks of insertion. Placing a PA catheter is likely to yield more benefit in patients with known or anticipated right heart failure, pulmonary hypertension, severe valvular abnormalities, or contraindications to TEE.
Induction and Maintenance of Anesthesia
Anesthetic induction in cardiac patients requires navigating a careful balance between avoiding hypotension and attenuating responses to laryngoscopy, TEE probe insertion, and surgical incision. Over the past several years, a variety of anesthetic techniques have been used successfully. As with premedication, choosing among different anesthetic regimens should not be a rote practice but rather a thoughtful consideration of their individual benefits and disadvantages in the context of the patient’s own physiologic profile.
Induction with high doses of opioids became popular in the 1970s and 1980s because of their hemodynamic stability and excellent attenuation of stress response when compared to the inhaled agents of the time (e.g., halothane). Induction can be achieved with large doses of morphine (1–2 mg/kg), fentanyl (50–100 mcg/kg), or sufentanil (10–25 mcg/kg). The accompanying vagotonic effects and chest wall rigidity can be counteracted to some extent with timely administration of pancuronium. However, pure high-dose opioid induction without an accompanying amnestic agent (such as midazolam or scopolamine) is associated with an unacceptably high occurrence of intraoperative awareness and postoperative recall. Also, prolonged postoperative respiratory depression lasting up to 12–24 h delays weaning from mechanical ventilation. Although high-dose opioid induction has been mostly supplanted by other techniques, it is still a useful option in high-risk patients who are expected to require prolonged postoperative ventilation.
The impetus to reduce the duration of postoperative ventilation has increased interest in combined intravenous-inhaled anesthesia techniques. Anesthesia for cardiac surgery can be induced with nearly any intravenous amnestic agent, such as etomidate (0.1–0.3 mg/kg), propofol (0.5–2 mg/kg), thiopental (1–2 mg/kg—no longer available), or ketamine (1–2 mg/kg). Etomidate is commonly used for cardiac inductions because myocardial contractility and preload remain relatively well preserved compared to other agents. Propofol can significantly reduce cardiac output and systemic vascular resistance, while thiopental can reduce cardiac preload via increased venous pooling. Nonetheless, propofol and thiopental are useful induction agents in hemodynamically robust patients, provided they are titrated slowly to achieve the desired effect with less medication. Ketamine stimulates the sympathetic nervous system, increasing heart rate and blood pressure. This makes ketamine the favored induction agent in patients with cardiac tamponade or severe hypovolemia, but the increase in heart rate can worsen myocardial ischemia. Ketamine can also depress myocardial function in patients with depleted catecholamine levels.
Anesthesia can be maintained with an inhalational agent, continuous opioid or sedative infusions, or a combination of these techniques, depending on hemodynamic stability and expected time to extubation postoperatively. If total intravenous anesthesia is used, the infusions should be delivered through a line that will not be obstructed by cannulation snares; alternatively, infusions can be given directly through the CPB machine during bypass. The inspired oxygen concentration can be titrated to oxygen saturation readings from the pulse oximeter or arterial blood gases. Many providers choose to use 100 % oxygen to maximize inspired oxygen tension, particularly in patients with known or evolving ischemic disease. An air–oxygen mixture can help prevent absorption atelectasis and reduce the risk of oxygen free radical toxicity from prolonged ventilation. Nitrous oxide is typically avoided because it decreases the maximum inspired oxygen concentration, stimulates catecholamine release, increases pulmonary vascular resistance, and enlarges air emboli.

Full access? Get Clinical Tree
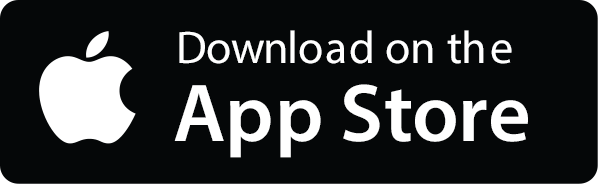
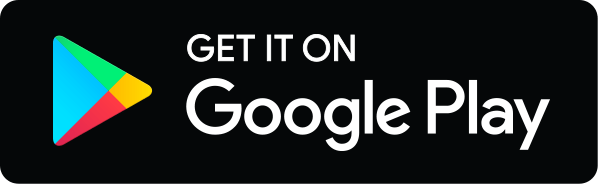