Chapter 11
Broad Principles of Antibiotic Usage
and Surgical Antimicrobial Prophylaxis
Jameel Ali and Addison K. May
Chapter Overview
Antibiotic usage is very common in the surgical intensive care unit (ICU) both for management of infection as well as prevention of anticipated infection. Rational use of antibiotics requires an understanding of their role and limitations in preventing infections, antibacterial spectrum of the various antibiotics, and their potential risks, particularly their contribution to the development of resistant infectious complications. In this chapter, we discuss some broad general principles of antibiotic usage, followed by principles of surgical antimicrobial prophylaxis to guide the intensivist in the rational effective use of these agents.
A Listing And Brief Considerations of Broad General Principles of Antibiotic Usage
A. Rational use of antibiotics is important not only for treating and preventing infection but also to avoid complications, including antibiotic resistance.
B. Generally, broad spectrum antibiotics should be avoided when a narrower spectrum more specific agent is available.
The selective influence of exposure to broad spectrum antibiotics on subsequent resistant pathogens is demonstrated in a study by May et al. Prior to 2002, Vanderbilt University Medical Centre, a Level 1 Trauma Centre with over 3,000 adult trauma admissions per year, utilized broad spectrum antibiotics (ceftriaxone plus vancomycin plus/minus metronidazole) for intracranial pressure monitor prophylaxis during the course of monitor placement. In April 2002, this practice was changed to narrow spectrum antibiotic prophylaxis with cefazolin at the time of insertion. The overall broad spectrum utilization decreased from 100% in 2002 to less than 20% in 2003. No significant change in the rate of CNS infections occurred as result, although the absolute percent was lower in the narrow prophylaxis group (1.8% with narrow spectrum coverage and 4.0% with broad spectrum coverage, p > 0.05).1 However, patients receiving broad spectrum antibiotics are more likely to be associated with subsequent infections with resistant pathogens. Patients receiving broad versus narrow prophylaxis had a significant increase in the frequency of infections with Acinetobacter species (21% versus 13% of pathogens), an increase in resistance of gram-negative pathogens (average resistance to gram-negative classes — 47% in the broad group versus 20% in the narrow group, p < 0.01), and a very significant increase in multidrug resistant pathogens (72% versus 23%, p < 0.01).
Recent systematic reviews and meta-analyses2 have allowed examination of important questions regarding combination versus monotherapy. These reviews have clarified the following issues:
(1) In patients who have sepsis, beta-lactam-aminoglycoside combination therapy does not improve patient outcome including mortality, when compared with beta-lactam monotherapy.
(2) In patients with sepsis, broad spectrum beta-lactam monotherapy is associated with improved outcomes when compared with similar spectrum beta-lactam-aminoglycoside combination therapy.
(3) Clinical evidence is lacking for patients who have serious gram-negative and Pseudomonas aeruginosa infections; currently there is no evidence to show that combination therapy improves patient related outcomes in these infections.
(4) Beta-lactam aminoglycoside combination therapy has not been shown to prevent the development of resistance that will have clinical implications for the individual patient.
(6) Combination therapy for gram-positive infections and endocarditis is unsupported currently by evidence from randomized trials or prospective studies. The few existing data do not point to an advantage for combination therapy.
Mechanisms of antimicrobial resistance for gram-negative bacteria include:
• Beta-lactamase production;
• Outer membrane impermeability and changes in porins;
• Efflux pumps;
• Degrading and antibiotic-altering enzymes;
• DNA gyrase mutations.
Antibiotic exposure is, however, the single strongest risk factor for subsequent antibiotic-resistant bacterial infection as indicated in the following table:
Certain classes of antibiotics have a greater likelihood of selecting for resistance as follows:
Broad spectrum cephalosporins:
Methicillin-resistant Staphylococcus aureus (MRSA), Vancomycin-resistant
Enterococcus (VRE), C. difficile, ESBLs, Acinetobacter
Quinolones:
MRSA, multiply resistant gram-negatives
Vancomycin:
MRSA, VRE
Clindamycin:
C. difficile
C. Timing of initiation and duration of antibiotics are important factors.
Kumar et al.3 demonstrated in 2,731 patients with septic shock and a mortality rate of 56% that time to antibiotic treatment was strongly associated with outcome. With each half hour delay there was a 12% increase in risk of death.
In a prospective, randomized, double blind clinical trial including 401 patients with VAP, Chastre et al.4 compared 197 patients assigned to eight days and 204 to 15 days of antibiotic treatment. There was no difference in mortality (18.8% versus 12.2%) or recurrent infections (28.9% versus 26.0%) but the eight day group had more antibiotic free days (13.1 versus 8.7). Among patients who developed recurrent infections multi resistant pathogens emerged less frequently in the eight day treated group.
In intra-abdominal infection, risk of therapeutic failure is increased when there is:
• Inadequate source control;
• Low albumin;
• High severity of illness (APACHE 11–15);
• Need for vasopressors;
• Diffuse peritonitis;
• Nosocomial origin;
• Comorbidity;
• Advanced age.
The Surgical Infection Society (SIS) guidelines for duration of antimicrobial therapy5,6 are:
• Antibiotic should be limited to no more than 5–7 days with the decision based on operative findings or upon resolution of fever or leukocytosis.
• Continued clinical evidence of infection at the end of antibiotic treatment should prompt a search for residual infection rather than prolonged therapy.
• In the absence of adequate source control, prolonged antibiotic therapy is warranted.
At the Vanderbilt University Medical Centre Critical Surgical Care Unit our approach to antibiotic therapy is as follows:
• Prophylaxis should only be instituted if there is proven efficacy.
• Broad empiric coverage when necessary should be based on unit specific criteria.
• The narrowest spectrum agent with proven efficacy (de-escalation) should be used.
• The least number of agents and least number of doses with equal efficacy, and dosed aggressively should be used.
• The shortest course with equal efficacy should be used.
If there are signs of infection, search aggressively for site and culture with ‘gold standard’ techniques.
• If empirical treatment is required before cultures, treat suspected infected site based on most common pathogens for that site.
• If the signs/symptoms are inadequate to suggest the site of infection, do not treat.
In an attempt to promote rational use of antibiotics in the ICU and prevent complications such as the development of antibiotic resistance, Antibiotic Stewardship programs such as those at Vanderbilt University Centre have been initiated. The Stewardship protocols include components on:
• Antibiotic prophylaxis regimes;
• Diagnosis and treatment of pneumonia utilizing quantitative broncho alveolar lavage;
• Diagnosis and treatment of sepsis;
• Antibiotic de-escalation and defined antibiotic courses;
• Hand hygiene program;
• Transfusion guidelines;
• Intensive insulin control;
• Critical care nutrition guidelines;
• VAP bundle (head of bed elevated, oral hygiene, daily spontaneous breathing trials, ICU;
• Sedation/analgesia-scale);
• Stress ulcer/DVT prevention;
• Central line insertion and management;
• Lung protective ventilator protocol.
The antibiotic rotation strategies taught in the Stewardship program have been shown to contribute to a reduction in gram-negative resistant pathogens7 with a relative change in infection rate from 67–90%. These stewardship programs can be associated with reduced use of broad spectrum antibiotics and a decline in resistant infections over time with significant impact on patient care and outcome.
D. When there is a choice between a bacteriostatic and bacteriocidal agent, the choice should be bacteriocidal unless issues such as toxicity dictate otherwise.
E. One of the first questions that should be answered early in the decision to initiate antibiotic therapy is whether antibiotics are required. Fever may be due to non-infectious causes and when present the infection is frequently non-bacterial and antibiotics could prove harmful.8 In the presence of a drainable source of infection such as an abscess, prompt drainage would usually avoid antibiotic treatment.
F. Choice of antibiotics is important and depends primarily on etiology of the infection but other patient factors and even cost may influence the choice. The etiology is frequently suggested by clinical presentation including site of the infection even before availability of laboratory culture results. Laboratory reports must be interpreted cautiously to rule out normal flora or contaminants. A positive clinical response is more important in deciding to continue the antibiotics than reliance on sensitivity results. The antibiotic’s ability to be absorbed orally, its ability to be concentrated in specific tissue and to cross the blood brain barrier are all important features to be considered. For instance, an antibiotic with a high urinary concentration would be more appropriately used to treat a urinary tract infection than one which has a lower urinary concentration.
G. The route of administration is determined by the Gastrointestinal (GI) absorption of the drug and the ability to use the gut. Frequently, even when oral absorption is possible, initial doses should be given parenterally for rapidity of onset and reliability of blood levels especially in urgent cases with findings such as hyper or hypothermia tachycardia and hypotension suggesting systemic sepsis. Drug–drug interaction with non-antibacterials may alter the blood level of antibiotics and drug dosage adjustment may be required.
H. Cost also is an important determining factor when not only cost per dose but for the entire duration of treatment should be considered.
I. Antibiotics all have variable toxicity profiles which are dose related. Choice of antibiotics and manner of administration must be taken into account in relation to toxicity particularly in patients with comorbidities most notably renal and hepatic disorders. Close monitoring of drug levels is essential to limit toxicity.
J. Patient factors must also be considered when administering antibiotics e.g., age and genetic abnormalities such as the administration of sulfonamides in patients with glucose-6-phosphate dehydrogenase deficiency.
K. In approaching patient factors, two concepts, among others, are worth considering: pharmacokinetics and pharmacodynamics.9,10
Pharmacokinetics relates to the time course of drug absorption, distribution, metabolism and excretion and its principles are applied to optimize drug therapy in an individual patient. Pharmacodynamics refers to the relationship between drug concentration and effect or toxicity. Each antibiotic has its own pharmacokinetic profile. Each class of antimicrobials has its own unique kill or inhibitory characteristics on bacteria. Dosing regimens should use these characteristics to optimize outcomes and minimize antimicrobial resistance recognizing that these profiles change over time particularly in critically ill patients warranting frequent reassessment of dosing regimens. Although these pharmacokinetic changes are highly variable in the ICU patient they may be more predictable if basic antibiotic characteristics (e.g., lipophilic or hydrophilic) are known and changes in patient characteristics such as volume status, end organ perfusion or function and pathophysiologic characteristics related to systemic inflammation and change in hemodynamics are considered. For instance, hydrophilic antibiotics such as beta-lactams, aminoglycosides, vancomycin, linezolid, and colistin have a low volume of distribution with predominantly renal clearance with associated low intracellular penetration, whereas lipophilic antibiotics such as fluoroquinolones, macrolides, clindamycin, and tigecycline have high volume of distribution, with predominantly hepatic clearance and good intracellular concentration. In critically ill patients the variability in pharmacokinetics leads to variability in antimicrobial concentration making it less likely to reach pharmacodynamics targets with resultant risk of treatment failure and increased risk of antimicrobial resistance. This is compounded by the effect of critical illness on volume of distribution and clearance of antibiotics. The septic patient is particularly vulnerable to changes in volume of distribution. For example, aggressive fluid resuscitation, the increased extravascular volume from systemic inflammation and associated capillary leak, third spacing of albumin and high output fistulas through postsurgical drains can result in an increase in volume of distribution with associated decreasing antibiotic serum concentration particularly with hydrophilic drugs such as aminoglycosides and beta-lactams. Other characteristics of the septic patient may also affect drug clearance. For example the hyper dynamic state can increase both creatinine clearance and hepatic perfusion leading to increased drug clearance. End organ dysfunction can reduce metabolism and elimination of drugs leading to decreased clearance. In addition, adaptive mechanisms in MOF can increase clearance in the presence of renal failure e.g., gastrointestinal clearance of ciprofloxacin is increased in renal failure. Also, biliary clearance of piperacillin increases in renal failure.
The relationship between half-life of the drug (T½), volume of distribution (Vd) and drug clearance (Cl) is T1/2 = 0.693 × Vd/Cl. From this equation there is the expected prolonged half-life accompanying decreased clearance but unexpected prolongation of half-life also results from increased volume of distribution.
Protein binding is also a potential source of variability in both clearance and volume of distribution. However, this is only relevant for highly protein bound drugs. Most antibiotics have low protein binding. Notable exceptions are: ceftriaxone (95% bound), ertapenem, teicoplainin, aztreonam, and daptomycin.
The above discussion emphasizes that there are huge inter patient and intrapatient variability in pharmacokinetics which is exaggerated in the ICU patient making our dosing strategy frequently unreliable with frequent failure to reach pharmacodynamic targets. A strategic approach to these problems includes: recognizing their presence; monitoring patients’ clinical response and changes in pathophysiologic status that would prompt changes in antibiotic dosing strategies; frequent reevaluation of antimicrobial dosing as clinical changes result in changes in volume of distribution and clearance over time.
L. As pointed out above, duration of treatment is an important factor and a good principle is to limit duration to the period by which the signs and symptoms of infection have resolved and avoid prolonged administration in order to limit the emergence of resistant organisms or super infection. Certain infections require longer duration e.g., endocarditis — 4 weeks, tuberculosis — 4–6 weeks, and osteomyelitis — 4 weeks.
M. Monitoring response to treatment allows determining whether to (a) increase the level by changing routes from oral to intravenous, increasing dosage or spectrum, (b) continue with the chosen regime, (c) decreasing level of treatment-intravenous to oral, decreasing dose or spectrum, and (d) stop antibiotics when the infection has resolved, or the diagnosis has changed.
N. Causes of non-response to antibiotics include:
(1) Resistant organism;
(2) Incorrect diagnosis;
(3) Dose, choice or route is inappropriate;
(4) Inability to reach the infection site;
(5) Undrained pus;
(6) Foreign body or devitalized tissue that requires removal;
(7) Secondary infection;
(8) Non-compliance.

Full access? Get Clinical Tree
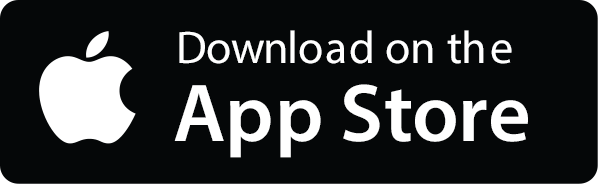
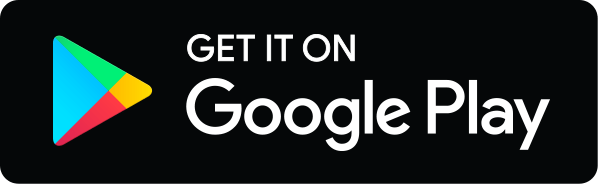