Body fluid compartments as percentage of body weight
The variations of TBW (% body weight) and ECF (% body weight) with age are shown in Figure 11.2.
Age | TBW (% body weight) | ECF (% body weight) |
---|---|---|
Neonate | 80 | 45 |
6 months | 70 | 35 |
1 year | 60 | 28 |
5 years | 65 | 25 |
Young adult | 60 | 22 |
Elderly | 50 | 20 |
In the young adult male total body water (TBW) forms approximately 60% of body weight.
In the young adult female TBW forms approximately 50% of body weight.
In the elderly TBW may decrease to 45% of body weight.
In the neonate TBW may be 80% of body weight.
Intracellular fluid (ICF)
The ICF compartment contains approximately 66% of TBW, equivalent to 40% of body weight. The water content and composition of ICF varies according to the function of the tissue. Water moves freely across, but the cell membrane is only selectively permeable to the ions. Water movement across the cell membrane helps to maintain osmolalities of ICF and ECF at equilibrium.
Extracellular fluid (ECF)
Extracellular fluid is about one-third of total body water (or 20% of body weight). Approximately 25% of ECF is in the vascular system and the remainder (75%) is interstitial fluid. Although ECF as a percentage of body weight varies with age (Figure 11.2), when expressed as an index of body surface area it remains relatively constant throughout life. ECF is composed of several components (Figure 11.3).
Fluid | % body weight | Volume (litres) |
---|---|---|
Interstitial fluid | 15 | 10.5 |
Plasma | 5 | 3.5 |
Transcellular fluid | 1 | 0.7 |
Total ECF | 21 | 14.7 |
Capillary endothelium is permeable to water, ions, and soluble substances such as urea and glucose, but impermeable to proteins. Therefore, the solute composition of plasma and interstitial fluid is similar except for the protein content. Because of the higher concentration of protein in the plasma, electrical neutrality across the two compartments is maintained by a slightly higher concentration of Cl− in interstitial fluid.
Plasma
Plasma volume equates to the intravascular component of ECF and amounts to about 5% body weight. The total blood volume is composed of plasma and the red blood cell volume. Plasma volume (VPL) can be measured using a dilution technique. The fractional red blood cell volume is readily available as the haematocrit (%). Given these values, the total blood volume (VBL) can be calculated:
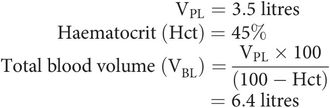
Transcellular fluid
The transcellular fluid compartment is composed of fluids that have been secreted but are separated from the plasma by an epithelial layer. These include:
Cerebrospinal fluid
Intraocular fluid
Gastrointestinal fluid
Bile
Pleural, peritoneal and pericardial fluid
Sweat
The composition of transcellular fluid differs from both plasma and interstitial fluid, since it is controlled by the secretory cells.
Measurement of fluid compartment volumes
Compartment volumes are estimated by radioactive dilutional techniques. In these methods, an indicator dye that is freely distributed (but contained) within the compartment being estimated is injected into the compartment. The mass of indicator used and the concentration in the fluid are measured. Then the size of the compartment can be determined using the formula:

Indicator methods used to estimate the volume of various compartments are summarised in Figure 11.4.
Compartment | Indicator | Comments |
---|---|---|
Total body water (TBW) | Antipyrine D2O | Tendency to underestimate uniform distribution |
Extracellular fluid (ECF) | Radioisotopes of Na+, Br−, Cl− | These enter cells and so overestimate |
Saccharides (mannitol, inulin) | Incomplete distribution and so underestimate | |
Plasma volume (VPL) | Radioisotope 131I albumin | Total blood volume (VBL) may be derived from VPL and haematocrit (Hct) |
Red cell volume (VRBC) | Red cells tagged with radioisotope 51Cr | Measures fraction of red blood cells tagged to determine VRBC |
Measures concentration of tagged cells to determine VBL | ||
Intracellular fluid (ICF) | Derived from: ICF = TBW – ECF | |
Interstitial fluid volume (VINT) | Derived from: VINT = ECF – VPL |
Solutions and semipermeable membranes
Concentration of a solution
The concentration of a solution is usually expressed in terms of the amount of solute present in a given amount of solvent. In the body, concentrations can be varied in fluid compartments by the movement of solute or solvent (water) into a compartment. The concentrations of various substances can be critical for normal function (e.g. extracellular potassium). Several units are used to express concentration, and the following definitions should be noted.
Amount of solute
A given amount of any substance can simply be measured by its mass. In chemical reactions, activity of a substance is related to the number of molecules present, and it is more useful to use a unit of mass that relates to the number of molecules present, the mole (symbol = mol), rather than a unit of absolute mass such as the kilogram.
One mole of a substance is defined as the mass of substance containing 6.022 × 1023 (Avogadro constant) molecules.
Chemical and electrochemical activity of a solution
The effects exerted by a solution are related to concentration. This is most commonly expressed as mass per unit volume (mg mL−1, g L−1, kg m−3). However, the chemical and electrochemical activity of a solution is more closely related to the number of molecules present in a given amount of solution. Concentration of a solution is thus better expressed in terms of its molarity or its molality.
Molarity – moles of solute per litre of solution (solute plus water) (mol L−1)
Molality – moles of solute per kilogram of solvent (water) (mol kg−1 H2O)
Equivalent weight
Chemical reactions between elements occur with fixed proportions of different elements by weight.
A gram equivalent weight can be defined for each element. This is the weight of an element that reacts with 8.000 g O2.
An electrical equivalent weight can also be defined for an ion, which is equal to the atomic weight divided by its valency.
Thus, the electrical equivalent weight of Na+ (atomic weight = 23) is 23/1 = 23, while the electrical equivalent weight of Ca2+ (atomic weight = 40) is 40/2 = 20.
Movement of water across a membrane
The membranes separating the fluid compartments generally allow the free passage of water, but not solutes, across them. Such membranes are known as semipermeable membranes. If a semipermeable membrane separates two aqueous solutions of different concentrations, water molecules will diffuse across the membrane to equalise the concentrations. Osmosis describes this diffusion process. The osmotic activity of the solutes is dependent on the number and not the type of free particles in solution.
Osmotic pressure
The osmotic activity of solute particles in an aqueous solution can be visualised as exerting an osmotic pressure, which would potentially draw water into the solution. This can be demonstrated as a hydrostatic pressure difference between two compartments separated by a semipermeable membrane, one containing solution and the other containing water alone.
Osmotic pressure is defined as the pressure required to prevent osmosis when the solution is separated from pure solvent by a semipermeable membrane (Figure 11.5).
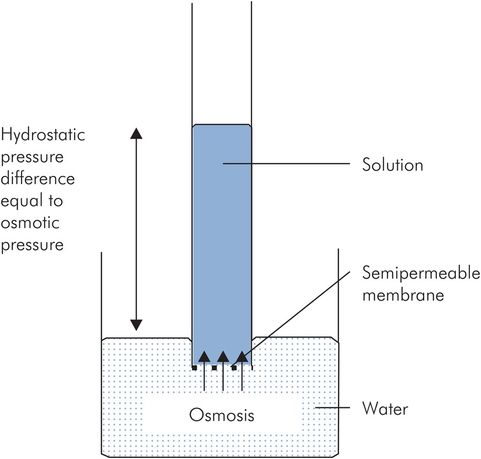
Definition of osmotic pressure
Calculation of osmotic pressure from molality of a solution
The osmotic pressure of a solution can be calculated from the solution molality. In a dilute solution, with a solute which does not dissociate or associate, osmotic pressure (π) is dependent on temperature and molal concentration. This can be expressed as:
Also:
In 1877, van’t Hoff noted the similarity of behaviour between dilute solutions and gases. Combining the two relations above gives the van’t Hoff equation for the osmotic pressure exerted by a dilute solution as:
osmotic pressure (π) = RTC (pascals)
where: R = universal gas constant (= 8.32 J K−1)
T = absolute temperature (K)
C = osmolality (mOsm kg−1 H2O)
This can be applied to find the osmotic pressure of plasma at body temperature (T = 307 K, C = 290 mOsm kg−1 H2O), thus:

The osmole
The concentration of solute particles in solution can be expressed in osmoles (Osm), to reflect the osmotic activity of the solution, where:
For a substance that does not associate or dissociate in solution (e.g. glucose),
For a substance which dissociates into two osmotically active particles (e.g. NaCl → Na+ + Cl−),
Osmolality and osmolarity of a solution
Osmolarity is the concentration of a solution expressed in osmoles of solute per litre of solution (solute plus water). The units of osmolarity are thus osmoles per litre (Osm L−1) or milliosmoles per litre (mOsm L−1).
Osmolality is the concentration of a solution expressed as osmoles of solute per kilogram of solvent (water alone). The units of osmolality are thus osmoles per kg water (Osm kg−1 H2O) or milliosmoles per kg water (mOsm kg−1 H2O). Osmolality is thus independent of temperature and the volume occupied by the solute.
As osmolality is independent of temperature, and independent of the volume taken up by the solutes within the solution, it is the preferred term in most physiological applications.
Osmolality of body fluids
The osmolality of a body fluid is usually higher than its osmolarity because of protein and lipid content, which occupy a small but finite volume. In practice this difference is insignificant except in cases of gross hyperproteinaemia or hyperlipidaemia.
Plasma osmolality ranges from 280 to 295 mOsm kg−1 H2O, and is maintained constant at about 290 mOsm kg−1 H2O throughout the body. The similar osmolality of all major body fluids is due to the free permeability to water of the endothelium and plasma membranes, which separate the various fluid compartments. The distribution and number of osmotically active particles contained by each primarily determine the size of these compartments.
Regulation of body fluid osmolality
The regulation of body fluid osmolality is inextricably linked to the control of total body water (TBW). It involves the secretion of vasopressin (antidiuretic hormone, ADH) in response to an increase in osmolality or a decrease in TBW. Although osmoreceptors appear sensitive enough to respond to small changes in osmolality, their response can be overridden by the haemodynamic response to changes in effective circulating volume. An outline of these responses is shown in Figure 11.6.
Measurement of osmolality
The osmolality of a solution can be estimated in practice by measuring depression of freezing point of the solution, when compared with the pure solvent. Plasma osmolality can also be estimated from the molality of the major solutes: sodium, chloride, urea and glucose:

Tonicity of a solution
Tonicity describes the relative osmolality between two fluid compartments. All solutes in ECF contribute to osmolality; however, only solutes which do not cross the cell membrane contribute to the tonicity.
Tonicity determines movement of water between two compartments. If one compartment contains a solution of lower osmolality, it is hypotonic compared with the higher-osmolality compartment. Usually this term is used to describe the effective osmotic pressure of a solution relative to that of plasma.
In an isotonic solution cells can be suspended without a change in cell volume. Cells placed in a hypertonic solution will shrink in volume, and in a hypotonic solution they will increase in volume.
A 0.9% solution of NaCl is approximately isotonic (308 mOsm kg−1 H2O).
Plasma colloid osmotic pressure
The capillary endothelium is impermeable to the plasma proteins, albumin and globulins. The retained plasma proteins raise the plasma osmotic pressure above that of the interstitial fluid by an amount referred to as the colloid osmotic pressure (or oncotic pressure). Quantitatively, the most important protein contributing to the colloid osmotic pressure is albumin, which is responsible for up to 75% of the total 25 mmHg colloid osmotic pressure.
Distribution of a solute across a membrane
Solute particles are distributed between fluid compartments according to the permeability of the separating membrane to each type of particle.
Both passive and active mechanisms determine the movement of solutes across a membrane. Active processes such as carrier proteins or ion channels selectively transport or allow the diffusion of substances across a membrane. Passive movement of particles can occur through pores or fenestrations, or even through the membrane, depending on lipid solubility.
Movement of solute across a capillary wall generally occurs in the filtrate as it passes from the capillary lumen into the interstitium. Large protein molecules remain in the capillary plasma unable to be filtered out because of their size. This capillary filtration process, and the balance of forces (Starling forces) driving it, is described in Chapter 15.
The main transport mechanisms involved across capillary endothelium are:
Filtration, which describes the action of hydrostatic pressure forcing fluid out of the capillaries. It is opposed by the plasma colloid osmotic pressure.
Diffusion, which is the passive movement of substances under the influence of concentration gradients, and occurs through fenestrations and intercellular junctions. The main diffusion barrier is the basement membrane.
Transcytosis, which is the active transfer of a substance by endocytosis from the capillary lumen, followed by exocytosis out of the endothelial cells. It represents only a small fraction of the total transport across capillary endothelium.

Full access? Get Clinical Tree
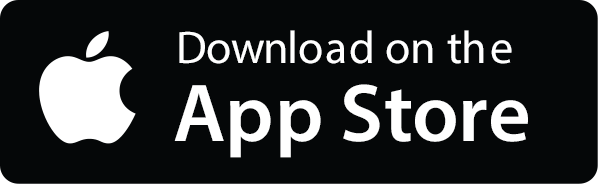
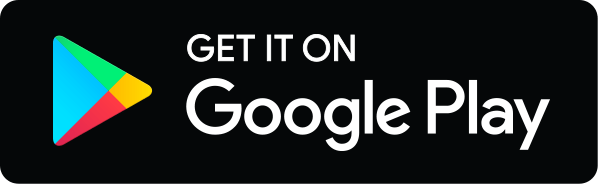