FIGURE 27.1 Dynamic equilibrium between hydrostatic (capillary) and oncotic pressures. Under normal conditions, there is a balance between the net hydrostatic pressure causing flux from the blood vessels into the interstitial space and the net oncotic pressure causing flux from the interstitial space into the blood vessels. The primary protein responsible for maintaining oncotic pressure is albumin, which occurs at a higher concentration in the blood vessels than in the interstitial space.
Albumin and Oncotic Pressure
The interstitial space functions in part as a reserve buffer of fluid, available as needed to provide additional fluid to the vascular space or accommodate excess fluid. Under normal circumstances, a constant flux of water across the capillary membranes between the vascular and interstitial spaces maintains a dynamic equilibrium. Hydrostatic pressure is higher in the vasculature than in the interstitial space, which causes water to flow out of the vascular space into the interstitial space. Counterbalancing this, the relatively higher concentration of albumin and other proteins in the vascular space results in a higher oncotic pressure, causing water to flow out of the interstitial space into the vascular space as described by Starling forces (Fig. 27.1). Albumin is the primary protein responsible for maintaining oncotic pressure (18). An adequate pool of albumin is needed to maintain the pressure gradient between the vascular and interstitial spaces, and a low enough capillary permeability is needed to keep albumin from transudating too quickly out of the circulation into the interstitial space. Normally, albumin transudates out of the plasma into the interstitial space at a rate of approximately 0.05% to 0.25% per minute (13). Albumin eventually returns to the circulation via the lymphatic system and re-enters the circulatory system via the thoracic duct. However, if too much albumin leaves the circulation too quickly, then the relative concentration of vascular albumin to interstitial albumin—and thus the oncotic pressure—decreases, causing a decrease in plasma volume. This can result in a capillary leak syndrome with a collapse of intravascular volume. Approximately 60% of the total body albumin is in the interstitial space (19).
The Kidneys and the Renin–Angiotensin–Aldosterone System
The kidneys are of particular importance in blood volume regulation. Under optimal circumstances, the kidneys’ rate of excretion of sodium and water adjusts continually to maintain a normal whole blood volume.
When the kidneys receive decreased perfusion, the rennin–angiotensin– aldosterone (RAA) system is activated. The RAA system includes both rapid- and slow-response mechanisms. The rapid response, a rise in blood pressure caused by angiotensin-mediated vasoconstriction, occurs almost immediately. The slower response, an increase in plasma volume caused by the actions of aldosterone, can occur over the course of days. The kidneys’ response is essentially primitive—they respond to changes in perfusion without being able to differentiate the cause. Thus, while the kidneys ideally function to regulate blood volume, sometimes their responses are maladaptive. For example, if an individual has a normal blood volume but has renal artery stenosis or heart failure, the RAA system is activated, vasoconstriction increases, and excess plasma volume is retained even if the individual has a normal or even expanded blood volume. In congestive heart failure patients, reduced cardiac output results in hypoperfusion of the kidneys and a maladaptive syndrome which results in increased retention of sodium and water resulting in an expanded plasma volume and blood volume (20,21).
The pituitary gland also plays a role in blood volume maintenance. It responds to increased concentration of solutes in plasma or decreased blood pressure by secreting antidiuretic hormone (ADH, also known as vasopressin), which stimulates water reabsorption in the kidneys, reducing urine output. Hyponatremia has been observed in intensive care units, particularly in septic patients. Like the kidneys, the pituitary gland responds to indicators of decreased volume without being able to differentiate the cause.
Red Blood Cell Volume Maintenance
Red cell volume is primarily maintained through a balance of production (erythropoiesis) and destruction (hemolysis). Red blood cells are created in the bone marrow and, at the end of their life span, hemolyzed in the spleen or the liver. In the presence of normal bone marrow function, the rate of red cell production is controlled by the hormone erythropoietin, which is produced by the kidney, with the rate of production affected by indicators of blood oxygenation. If red blood cells are lost (such as through hemorrhage), they can be replaced through the manufacture of new cells by the bone marrow. It can take days to months to replace lost red cells, depending on the amount lost and an individual’s capacity for creating new red cells. A study of healthy males who donated two units of blood found that the subjects took a month to replace an average of 92% of the lost blood (22).
Difficulties in Estimating Blood Volume
Many of the measurements available in a clinical setting are indicators or proxy measurements for perfusion (local or systemic), vasomotor tone (local or systemic), or blood volume. These measurements may include:
- Blood pressure and heart rate
- Blood gases, including pH, base deficit, and lactic acid as estimates of perfusion
- Hematocrit and hemoglobin as surrogate tests for red cell volume
- Blood urea nitrogen (BUN)/creatinine as an estimate of kidney function
- Urine output as an estimate of kidney function and/or perfusion
- Invasive procedures such as pulmonary artery catheter (PAC) for determination of intravascular pressures
- Clinical maneuvers such as passive leg raising have been shown not to have a high correlation in response to fluid challenges (7,8)
None of these, however, is a direct measure of volume status. The physician in the critical care setting is faced with the difficult situation of administering or withholding fluids, blood, and blood components on the basis of these surrogate tests. In particular, hemoglobin and hematocrit are frequently inaccurate surrogate markers for blood volume. When using hematocrit or hemoglobin to estimate red cell volume, it is assumed that the whole blood volume remains normovolemic (euvolemic)—for example, that fluid replacement of lost red cells via plasma expansion is rapid but may not be complete. This is frequently not the case. Review articles on fluid management discuss a variety of complex factors to consider when estimating a patient’s volume status (23,24), and clinical estimation is frequently inaccurate. Monitoring blood volume using clinical assessment and proxy measurements can be particularly misleading in the critical care setting, because compensatory responses to acute blood volume derangements occur at different rates. Changes in vasomotor tone may occur nearly instantaneously, while changes in plasma volume may occur over hours or days and may not completely compensate for lost red cells or albumin. Following acute blood loss, rapid changes in vasoconstriction, which can occur before any compensatory volume expansion takes place, may maintain a relatively normal peripheral blood pressure and hematocrit at the expense of organ perfusion. Administration of fluids, blood, or blood components can additionally complicate the diagnostic picture.
A 2003 study (25) compared clinical estimates of intravascular volume with estimates obtained by determining corrected left ventricular flow time from transesophageal Doppler imaging. Clinical estimates agreed with Doppler imaging results only 30% of the time. Therefore, it is clear that the Doppler imaging technique is not accurate for estimating blood volume.
It is a common intuitive assumption that achieving normovolemia facilitates effective perfusion and contributes to improved outcomes. Although no studies have explicitly evaluated clinical assessment against blood volume measurement in the critical care unit.
Additional recent studies have provided evidence that achieving normovolemia is a valid goal in a number of clinical settings. In a heart failure study performed at Columbia Presbyterian Hospital, among 43 nonedematous, hypervolemic patients had a 2-year mortality rate of 57%, while normovolemic and slightly hypovolemic patients had a 2-year mortality rate of 0%. The American College of Cardiology has previously recommended assessment of volume status as an important factor in the diagnosis and treatment of heart failure, but this was the first study to provide a clear association between measured blood volume and patient outcome with respect to treatment achieving normovolemia (20,21).
INTERPRETING BLOOD VOLUME MEASUREMENT RESULTS
Units of Measurement
In addition to absolute measurements, blood volume results for each compartment should be presented as the patient’s deviation from his or her normal volume, both as a percent deviation and in milliliters. The percentage indicates the severity of the patient’s blood volume, and the absolute quantity of the depletion can help guide treatment.
Relationship Between Whole Blood, Red Cell, and Plasma Volumes
When interpreting blood volume results, the whole blood volume should be considered first. Homeostatic mechanisms attempt to restore blood volume to normal even if it dilutes the remaining red cells (11). The relationship between the peripheral hematocrit and the normalized hematocrit will provide guidance for those not familiar with blood volume measurement results. Plasma volume results should be considered from the perspective of whether they are homeostatic or pathologic. For example, a patient with a diminished red cell volume should have an expanded compensatory or homeostatic expansion of the blood volume. Patients who have loss of red cells and hypoalbuminemia can be difficult to evaluate clinically unless a blood volume measurement, including red cell volume and plasma volume, is performed to elucidate the underlying derangement. Hypoalbminemia alters the normal ratio of one part plasma volume to three parts interstitial volume. Patients with severe hypoalbuminemia, therefore, will, out of necessity, require expanded interstitial volume, as manifested by peripheral edema, to achieve a normal blood volume.
The Rate of Transudation
Albumin I131 is an ideal tracer for measuring blood volume. Albumin normally transudates out of the circulation into the interstitial space at a rate of approximately 0.05% to 0.25% per minute (5,11). Obtaining a minimum of five-timed samples enables calculation of the transudation rate. Rates between 0.25% and 0.4% are suspicious for a capillary leak syndrome. Rates above 0.5% are strongly suggestive of a capillary leak syndrome. Capillary leak syndrome will result in hypoalbuminemia and hypovolemia. Sepsis and burn patients are classic examples of patients with these conditions. A major clinical error is to attempt to diurese patients who have hypoalbuminemia because they have evidence of peripheral edema, suggesting total body water. Such patients require an expanded interstitial volume to maintain a normal blood volume. Over diurising such patients to remove their peripheral edema will result in hypovolemia, hypoperfusion, and may result in renal failure or multiple-organ failure.
APPLICATIONS OF BLOOD VOLUME MEASUREMENT IN CRITICAL CARE
Undetected Hypovolemia
Early detection of hypovolemia is essential. By the time a patient becomes symptomatic, hypovolemia is often extreme, damage may have already occurred to critical organs (the gut and the kidneys are particularly susceptible), and deterioration may be rapid and unexpected (5).
In acute situations, the current primary measures used to track perfusion and evaluate fluid replacement requirements include pressure measurements (such as central venous pressure, intra-arterial or indirect auscultating blood pressure, and pulmonary artery catheter measurements) in conjunction with hematocrit/hemoglobin measurements. However, the body can respond to hypovolemia by initiating vasoconstrictive defense mechanisms, maintaining near-normal pressures even in the face of severe blood loss, and allowing the hypovolemia to remain undetected.
Hypovolemia is generally more dangerous and urgent than the same degree of hypervolemia, and sudden blood loss is more urgent than the same degree of chronic hypovolemia. A patient may tolerate a 40% increase in whole blood volume or an 80% increase in red cell volume for some time without suffering acute negative effects, but a 40% loss of blood or an 80% loss of red cells is an extreme medical emergency. A sudden loss of as little as 20% of the blood volume triggers an acute vasoconstrictive response, and a sudden 30% loss can lead to circulatory collapse. A rapid 40% to 45% loss is incompatible with life. Further, an already anemic or hypovolemic patient who experiences sudden blood loss will be less able to tolerate that loss than would a normovolemic or hypervolemic patient. Even after fluid resuscitation (whether after partially compensated shock or circulatory collapse), damage to the gut and kidneys may result in severe irreversible complications (26).
Undetected Hypervolemia
In the critical care unit, hypervolemia may be a result of comorbidities or iatrogenic causes such as excessive fluids. Chronic hypervolemia may develop slowly and is most frequently related to cardiac disease, particularly in heart failure. In contrast, acute hypervolemia is almost always iatrogenic. Particular attention must be paid to patients who are oliguric or in renal shutdown, as these patients cannot remove excess fluid through urine output. A major common clinical error is to equate evidence of fluid overload such as obvious edema as proof that the patient is hypervolemic. The patient may have paradoxical hypovolemia, particularly if the patient is also hypoalbuminemic. The patient may also have a capillary leak syndrome, which predisposes to hypovolemia. It is essential to avoid this mistake. An accurate blood volume measurement will quickly clarify the situation and save the lives of critically ill patients.
The hypervolemic patient is at risk for the development of pulmonary edema in response to increased pressure; hypoalbuminemia, which predisposes to pulmonary edema. Pulmonary hypertension as a maladaptive mechanism observed in chronic heart failure that may eventually lead to permanent pulmonary hypertension and worsening of heart failure.
Blood volume measurement can be used to accurately diagnose the presence and quantify the hypervolemia. Treatment can vary depending on the severity of the patient’s hypervolemia and the patient’s kidney function and may include fluid restriction, diuretic therapy, hemodialysis if the patient is in kidney failure, or ultrafiltration. It is important to remember that patients can usually tolerate moderate degrees of hypervolemia with minimal consequences as compared to the consequences of moderate hypovolemia (5).
The Bleeding Patient: When to Perform Blood Volume Measurement
If a patient has continuing massive bleeding such as, for example, greater than 100 mL/hr, blood volume measurement should be performed when the patient is semi-stabilized. Fluid pressures may be a helpful guide. In a patient who is bleeding at an estimated lower rate, for example, 60 mL/hr, a blood volume can be accurately performed and be quite useful. A patient losing 80 mL of blood per hour would lose 1920 mL of blood in a 24-hour period. This is a massive loss of blood. Since a blood volume measurement requires 40 minutes or less from the time of injection to completion of collection of five samples, such a patient would lose approximately 54 mL of blood. This would represent 1% to 3% of a patient’s blood volume and lend a slight increase of inaccuracy to the measurement.
Each individual blood volume has a calculated degree of accuracy for that specific blood volume. If a measured blood volume had a measured accuracy of ±4% and a reported result of −30%, the result would still be very helpful in defining the patient as severely hypovolemic. It would also quantify the degree of the deficit.
Tracking Changes in Blood Volume and Performing Follow-Up Measurements
After an initial blood volume measurement, it is possible in a nonbleeding patient to track changes in blood volume with precise hematocrit measurements. If the patient’s red cell volume remains stable, changes in the hematocrit reflect changes in blood volume as follows:
Plasma volume = red cell volume × (1 − hematocrit)/hematocrit
Whole blood volume = red cell volume/hematocrit
For example, consider a patient who is found to have a measured red cell volume of 2,000 mL, plasma volume of 4,000 mL, and hematocrit of 33%. This patient is diuresed, and the hematocrit rises to 40%. The new volume is equal to:
Plasma volume = 2,000 mL × (1 − 0.4)/0.4 = 3,000 mL
Whole blood volume = 2,000/0.4 = 5,000 mL
This relationship is particularly important to understand and can be used in analyzing changes in blood volume on patients undergoing renal dialysis. One study (27) demonstrated that in a nonbleeding patient undergoing acute volume changes, whether from dialysis or diuretics or ultra-filtration, it is possible to determine and quantify whether fluid being removed from the patient is primarily from the interstitial space or the intravascular space. This enables treatment choices to be made in direct response to blood volume compartment changes which are measured rather than rough estimates based on indirect surrogate measures.
If a nonbleeding patient receives a transfusion, the volume response may be roughly estimated based on the type of fluid transfused and its expected effect on the hematocrit; a follow-up blood volume measurement may be needed for precise quantification. If a patient is bleeding or otherwise experiences a change in red cell volume that cannot be reasonably estimated, blood volume changes cannot be tracked with precision via changes in hematocrit. A follow-up blood volume measurement should be performed 24 to 48 hours after treatment is initiated.
In general, changes in blood volume may correlate with changes in symptoms, hemodynamic measurements, or clinical status, but these relationships are not necessarily straightforward. In one study of acute decompensated heart failure patients (28) after 24 to 48 hours of treatment blood volume correlated better with some hemodynamic measurements than did brain natriuretic peptide (BNP) levels. However, no measurements correlated closely enough with blood volume results for any hemodynamic measurement to serve as a surrogate measure for volume status, or vice versa.
BLOOD VOLUME MEASUREMENTS IN COMMON CRITICAL CARE SITUATIONS
Shock
The presentation of symptoms in shock may not be straightforward and can complicate assessment of the patient’s volume status, especially in situations where several factors contribute to shock. Blood volume measurement can be of major importance in understanding the underlying cascade of events that precipitate shock and determining appropriate treatment. In a patient with hypovolemia, even in conjunction with other contributing factors, appropriate transfusion and fluid replacement are needed before severe multiple-organ hypoperfusion and failure ensue.
For example, following a myocardial infarction, patients frequently become hypotensive. While cardiac damage usually plays a major, if not the predominant, role in the ensuing shock, blood volume derangements may play a significant additional role. A patient with a myocardial infarction (MI) may develop hypovolemia from severe vomiting, profuse sweating, or the use of anticoagulants. Sometimes blood loss secondary to gastrointestinal bleeding may trigger an MI. Because the blood loss may not be recognized as a precipitating factor in the MI, the patient may not be treated to restore volume. This may progress to renal or multiple-organ damage. Accurate assessment of the volume status and prompt treatment of volume derangements are important for all types of shock, even those that do not appear initially to be volume-related.
All patients who have shock from any cause should have a blood volume measurement to define the extent to which volume derangements, particularly hypovolemia, may be a causative factor. A patient who has a myocardial infarction may have hypovolemia either as a triggering factor or a secondary factor. It is essential to rapidly diagnose the patient and correct their hypovolemia with appropriate fluids. It has been demonstrated that clinical parameters, including information derived from PACs were not reliable in predicting blood volume in surgical intensive care patients (24).
Acidosis frequently develops from hypoperfusion and a shift to anaerobic metabolism, resulting in increased lactic acid production. Under these circumstances, the body’s metabolic defense mechanisms, which are strongly geared to maintain a pH of 7.4, may be overcome. At a pH of 7.0 to 7.1, major deterioration of all functions including cardiac metabolism occurs. At a pH of 6.85 to 6.9, the body’s metabolic systems are so diminished that death is imminent (29).
Acidosis may also develop from other underlying causes. For example, in diabetic acidosis, ketoacidosis develops from hyperglycemia. Hypovolemia may be a contributing factor, though, because the severely dehydrated patient may have localized ischemia.
Blood volume measurement may be helpful in elucidating the underlying cause of acidosis and determining optimal therapy. If the acidosis is caused by hypoperfusion related to diminished blood volume, aggressive and rapid therapy is needed before irreversible deterioration occurs. In situations such as diabetic acidosis, therapy should also be directed at correcting the underlying condition (such as hyperglycemia), correction of the electrolyte imbalance, and, most importantly, restoring the patient’s blood volume derangement. There are a multitude of various combinations of red cell and plasma volume derangements which can only be defined and quantified by a blood volume measurement.
Hypoalbuminemia
Hypoalbuminemic patients, because of a shift in oncotic pressure, may be predisposed to edema formation in order to achieve a balance of hydrostatic and oncotic pressures that can maintain a normal blood volume. Rather than a normal ratio of 3:1 of extracellular to vascular volume, equilibrium between the two spaces may be reached at a ratio of 4:1 or 5:1. In such patients, the goal is to maintain a normal blood volume even if that means allowing an expanded extracellular volume. It is a common mistake to focus treatment on removal of obvious peripheral edema. Patients with hypoalbuminemia and/or capillary leak syndrome may require a larger volume of extracellular fluid in order to maintain a normal blood volume.
Therapeutic albumin is underutilized because of the inability to correctly quantify total body albumin. A common mistake is to misunderstand the size of the albumin pool and that it may take 12 to 24 hours for administered albumin to equilibrate in the albumin pool. Albumin has a half-life of 13 to 15 days (30). Approximately 60% of the body’s albumin is in the interstitial space (25). A common misconception is to measure serum albumin concentrations 3 to 4 hours after administration of albumin and then measure albumin concentration 24 to 26 hours later and interpret the drop in albumin concentration as rapid metabolism of albumin instead of the prolonged equilibration period of albumin into the intravascular and interstitial pool. Hypoalbuminemia is a common problem seen in septic, diabetic, heart failure, renal and hepatic disease patients, which causes major blood volume derangements. These derangements can be quantified and appropriate therapy administered after blood volume measurement.
Hepatorenal Syndrome
In the hepatorenal syndrome, the liver and kidneys fail simultaneously. Frequently, this syndrome originates with liver damage that progresses to cirrhosis and portal hypertension, causing edema and ascites. If the patient is over diuresed to remove the edema, the patient becomes hypovolemic and the kidneys hypoperfused. If severe enough, this can lead to kidney failure, liver failure, and circulatory collapse.
Hepatorenal syndrome is essentially part of a cascade of circulatory decompensation that, if not corrected, usually results in multiple-organ failure and death. Quantifying the blood volume compartments of red cell volume and plasma volume is essential to detecting and correcting this situation. It is usually not possible to diurese a patient with liver damage to completely remove edema, because diuresis does not correct the underlying imbalance between intravascular and interstitial volume. A normal ratio of one part intravascular volume to three parts interstitial volume may now be altered so that the new ratio may be 1:5 or 1:6. After total body water reduction, the reduced fluid simply redistributes throughout the vascular and extravascular spaces in the same ratio. This situation is similar when using paracentesis to treat ascites. Because paracentesis only removes ascitic fluid and does not address the underlying fluid and pressure imbalance, the rapid removal of a large amount of ascetic fluid causes fluid to shift quickly from the vascular to the interstitial peritoneal space, resulting in hypovolemia, a drop in blood pressure, and collapse of the circulation.
Blood volume measurement can be performed on a patient with liver problems, edema, and/or ascites to determine what quantity of diuresis is possible without precipitating hypovolemia. A patient who is hypervolemic will be able to tolerate diuresis, and an edematous normovolemic patient should be diuresed only slowly and minimally, with careful follow-up. Some patients with edema and/or ascites may require a blood volume at the upper limit of normal in order to maintain adequate perfusion pressures. A patient who is hypovolemic should not undergo diuresis! Therapeutic use of albumin should be considered to help mobilize some of the acidic fluid and help maintain blood volume. Unfortunately, in late stages of the disease, the relief is relatively limited in time.
Diuretic Resistance
The term diuretic resistance is frequently used when patients do not respond to relatively large quantities of i.v. diuretics. To some extent the term may be a misnomer, because diuretic resistance may be a reflection of severe hypoperfusion. A patient in renal shutdown will obviously not respond to diuretics, and occasionally aggressive use of diuretics precipitates renal shutdown. To differentiate true diuretic resistance from hypoperfusion of the kidneys, blood volume measurement in conjunction with renal tests can be helpful. This differentiation is particularly important because aggressive use of diuretics in a patient with marginal perfusion to the kidneys caused by hypovolemia and hypotension may precipitate total renal shutdown. Hypoalbuminemia is a major confounding factor in treating patients with expanded total body water, as it predisposes to intravascular volume depletion.
A major common error is to equate the presence of obvious peripheral edema with evidence for intravascular volume expansion. This sometimes results in aggressive diuretic treatment in patients who have paradoxical hypovolemia that is further aggravated by the use of potent diuretics. Such patients are particularly susceptible to developing iatrogenic renal failure. Direct blood volume measurement can easily identify such patients and avoid inappropriate treatment that may lead to multiple-organ failure.
Cardiogenic and Noncardiogenic Pulmonary Edema
Cardiogenic pulmonary edema (caused by increased hydrostatic pressure in the alveoli), often secondary to hypervolemia, and noncardiogenic pulmonary edema (caused by damage to the membranes of the alveoli), also known as acute respiratory distress syndrome (ARDS), have different underlying causes and require different treatment approaches. The two conditions may present similar symptoms, and both are common in the critical care setting. When physical examination and noninvasive tests do not provide a definitive distinction, pulmonary capillary wedge pressure is often used to distinguish between the two, but results may be difficult to interpret in patients with pulmonary artery hypertension related to other conditions. Additionally, patients may have a combination of both conditions; increased hydrostatic pressure does not rule out damage to the alveoli. The relationship between blood volume and wedge pressures seems at best weak, with no correlation to central venous pressures (25).
Blood volume measurement, by detecting the presence or absence of hypervolemia, can be used in the differential diagnosis of cardiogenic and noncardiogenic pulmonary edema, especially in patients known to have pulmonary hypertension from other causes and in patients for whom invasive PAC is not desirable.
Hypervolemia is more likely to be present in a patient with cardiogenic pulmonary edema, while noncardiogenic pulmonary edema may develop in a patient with normovolemia or hypovolemia. However, because both conditions may coexist, hypervolemia does not rule out ARDS. These conditions must also be reviewed in the context of evaluating albumin, as hypoalbuminemia by itself will predispose to pulmonary edema. There have been documented cases of cardiac patients with pulmonary edema and hypoalbuminemia who are hypovolemic. Such patients need to be treated with 25% albumin and not treated with diuretics. The assumption that all patients with pulmonary edema and cardiac disease are hypervolemic may lead to inappropriate diuretic treatment.
Key Points
- While fluid resuscitation and blood volume management have long been mainstays in critical care, evaluation of blood volume has traditionally relied on assessment of the patient’s clinical condition, which is often misleading, and surrogate measurements to estimate volume status, which are often inaccurate.
- Blood volume measurement has been traditionally difficult to estimate in critically ill patients, and the clinical utilization of rapid semi-automated radioisotopic blood volume measurement has shown to improve accuracy of that measurement.
- On the simplest level, blood volume measurement results can be used as an alternative guide to improve accuracy when estimating volume status.

Full access? Get Clinical Tree
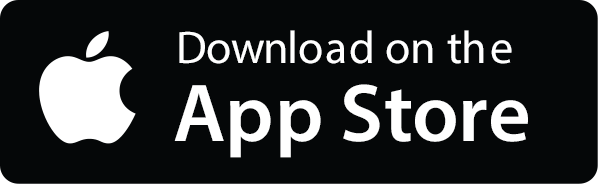
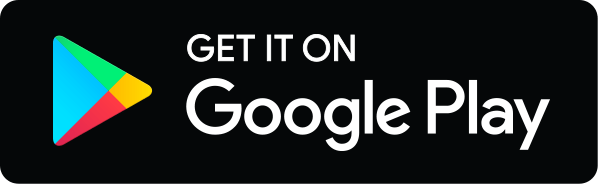