Fig. 6.1
EXTEM ROTEM trace of low platelet count in a patient with cirrhosis pre and post platelet transfusion
6.2.3 Adverse Events
A comprehensive review of adverse plasma transfusion reactions is beyond the scope of this chapter. Basically, the most frequent adverse events are febrile and allergic reactions, bacterial sepsis, and TRALI [29].
6.3 Cryoprecipitate
Cryoprecipitate, originally known as cryoprecipitated antihemophilic factor, was developed for the treatment of congenital hemophilia A, von Willebrand disease, and hypofibrinogenemia.
It is a concentrate of high-molecular-weight plasma proteins that precipitate when plasma is slowly thawed at 1–6 °C and the supernatant (cryosupernatant or cryoprecipitate poor plasma) is removed. The product is refrozen and contains factor VIII, von Willebrand factor, factor XIII, fibronectin, and small amounts of other plasma proteins.
One unit of cryoprecipitate is manufactured from one unit of fresh frozen plasma and generally has a volume of 10–15 mL despite a great variability in the volume as described in literature [2, 3, 42, 43].
Despite it is believed that cryoprecipitate contains the majority of fibrinogen present in plasma, in fact it contains about one-third. Nevertheless, because of its small volume, the final fibrinogen concentration is higher than in plasma. Actually, the fibrinogen concentration derives from donors, so there is a wide variation (3.5–30 g/L) in the final content of each unit [44].
Cryoprecipitate can be infused as single unit or as a pool of 5–10 single donor units. Pooling of cryoprecipitate can be prepared before freezing or after thawing [45].
The cryoprecipitate specification requires that 75 % of units contain at least 140 mg of fibrinogen and 70 UI/ml of factor VIII [9].
6.3.1 Indications
Cryoprecipitate was historically used for the treatment of congenital factor VIII deficiency and von Willebrand disease, but now, more safe, purified, and virus-inactivated products or recombinant drugs are available, making it contraindicated for this indication in the developed world.
The most common indication for cryoprecipitate is to increase fibrinogen levels in acquired hypofibrinogenemia seen in trauma setting, in obstetric patients with postpartum hemorrhage, and in disseminated intravascular coagulopathy (DIC).
To date, it is recognized that patients with major trauma (ISS > 15) will develop, early, a relative hypofibrinogenemia, defined as a fibrinogen level <1.5 g/L [46, 47].
The use of cryoprecipitate in this setting was investigated by Morrison and associates in the MATTERs II study [48]. Over a 5-year period, 1332 patients, requiring at least 1 unit of red blood cell concentrate, were identified from the UK and US Joint Theater Trauma registries. Eleven percent of the cohort received tranexamic acid only, 12.6 % received cryoprecipitate only, 19.4 % received both tranexamic and cryoprecipitate, and 56.9 % received no treatment. Mortality was the highest in the non-treatment group (23.6 %) and the lowest in tranexamic acid/cryoprecipitate group (11.6 %). The benefit of tranexamic acid and cryoprecipitate was similar: in fact both were associated with an odds ratio for mortality of 0.61 and 95 % confidence interval of 0.42–0.89 and 0.40–0.94, respectively.
In the civilian setting, Rourke and associates [46] investigated cryoprecipitate in coagulophatic trauma patients demonstrating that the standard UK dose of 2 pool of cryoprecipitate maintained basal fibrinogen level during transfusion of red blood cells without significantly increasing the level compared with patients who had not received cryoprecipitate.
In 2007 the European guidelines [49] on the management of bleeding after major trauma recommended treatment of acquired hypofibrinogenemia if significant bleeding is accompanied by a plasma fibrinogen level <1 g/L. This trigger was increased to 1.5–2 g/L in the update to the guidelines in 2013 [50], and cryoprecipitate is a therapeutic option.
In obstetric bleeding patients, UK guidelines for the management of postpartum hemorrhage published in 2009 and updated in 2011 [40] recommend fibrinogen supplementation with cryoprecipitate if fibrinogen levels are <1 g/L. The European guidelines on the management of severe perioperative bleeding suggest a higher trigger (2 g/L) due to the physiologically elevated fibrinogen concentration in pregnancy [51].
6.3.2 Monitoring the Efficacy of Cryoprecipitate
It is possible to monitor the efficacy of cryoprecipitate as hemostatic therapy measuring fibrinogen plasma concentration by Clauss method or by viscoelastic tests such as rotational thromboelastometry (ROTEM) or thromboelastography (TEG). Cryoprecipitate transfusion significantly improves clot firmness measured by EXTEM test and FIBTEM test (Fig. 6.2). Similar changes are observed for the MA at standard TEG and functional fibrinogen test.
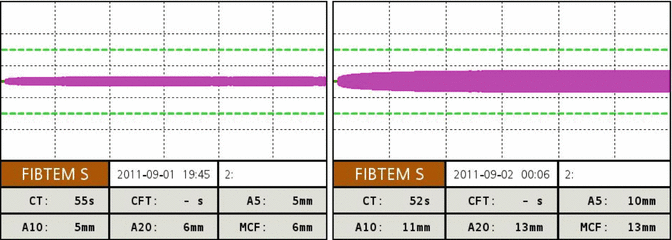
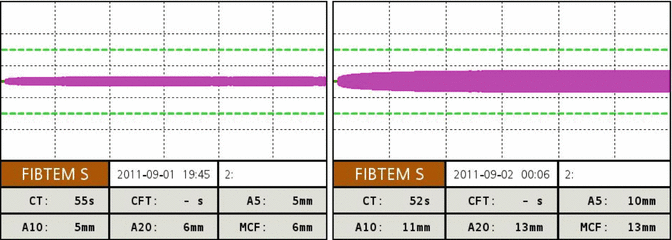
Fig. 6.2
FIBTEM ROTEM trace in a severely injured patient pre and after administration of 10 single units of cryoprecipitate
In a recent study Lee and associates [52] investigated the efficacy of cryoprecipitate in increasing plasma fibrinogen concentration (fibrinogen recovery) and quality of the fibrin-based clot in patients with bleeding during aortic surgery.
FIBTEM A10 was the test chosen to monitor the replacement therapy and the value of 6 mm as trigger for the administration of 10 units of cryoprecipitate.
The authors demonstrated that cryoprecipitate is able to increase clot firmness in FIBTEM A 10 and that the dose required to increase FIBTEM A10 by 1 mm was 13.2 mg fibrinogen/kg body weight or approximately 0.5 units per 10 kg body weight.
6.4 Antifibrinolytic Agents
Antifibrinolytic agents are commonly used to reduce blood loss and allogeneic blood transfusion during major surgery at high risk of bleeding such as cardiac, orthopedic, gynecological, urological, and neurosurgery.
The main antifibrinolytic agents are aprotinin, tranexamic acid, and e-aminocaproic acid.
6.4.1 Aprotinin
Aprotinin is a nonspecific serine protease inhibitor extracted from bovine lung acting by inhibition of the serine protease plasmin. The site of action is the active center of serine proteases. Aprotinin also inhibit trypsin, kallikrein, elastase, and thrombin and can interfere with the contact factor of coagulation (FXII).
Aprotinin was introduced for the first time in 1950 for the treatment of hyperfibrinolysis but became routinely used around 1990s in patients undergoing complex cardiac surgery [53].
The use of aprotinin came to the end after the publication of the BART (Blood conservation using Antifibrinolytic in a Randomized Trial) trial [54] conducted on 2331 patients at high risk of bleeding during cardiac surgery and randomized to receive aprotinin, e-aminocaproic acid, and tranexamic acid. The study was early interrupted because of an increased 30-day mortality rate in the aprotinin arm compared with the other two antifibrinolytic drugs (RR 1.53, 95 % CI, 1.06–2.22).
After these results, aprotinin was withdrawn from the market in 2008, but in 2011 the Canadian Health authorities [55] reviewed the BART trial results and authorized aprotinin in coronary bypass graft surgery. In 2012 the European Medicines Agency [56] removed the suspension after having reevaluated the risk profile of aprotinin.
6.4.2 Tranexamic Acid
Tranexamic acid is a synthetic lysine amino acid derivative that exerts antifibrinolytic activity reversibly binding to plasminogen and preventing its interaction with fibrin. Tranexamic acid also blocks the binding of alfa2-antiplasmin to plasma and its inactivation of plasmin and inhibits trypsin and weakly thrombin [61].
Tranexamic acid can be used intravenously, orally, or topically, and the ideal dosage for each route of administration is not well defined. In vivo and in vitro data demonstrated that the effective therapeutic plasma concentration for inhibiting fibrinolysis has been reported to be 5–10 mg/L or 10–15 mg/L, respectively [62, 63]. Intravenous administration of 1 g dose achieves plasma concentration >10 mg/l for up to 5–6 h; after oral and intramuscular administration, the maximum plasma concentration is reached at 2–3 h and 0.5 h, respectively [64]. The absorption of oral tranexamic acid is not markedly affected by food; therefore, it can be administered without regard to meals.
Tranexamic acid crosses the placenta and the blood-brain barrier, penetrates eyes, and rapidly diffuses into joint fluid and sundial membranes. It requires adjustment in case of renal impairment while there is no need in case of hepatic impairment.
Few pharmacokinetic data are available for pediatric patients. Recently the first pharmacokinetic study in children undergoing craniofacial surgery was published demonstrating that a loading dose of 10 mg/kg over 15 min followed by continuous infusion of 5 mg/kg/h is optimal to reach the minimal therapeutic plasma level of 16 μg/mL during this kind of surgery [65].
Renewed interest in tranexamic acid stems from the CRASH-2 (Clinical Randomization of an Antifibrinolytic in Significant Hemorrhage-2) trial [66] assessing the effects of early administration of tranexamic acid in trauma patients with or at risk of substantial bleeding. 20,211 trauma patients from 40 countries were randomized within 8 h of injury to receive either tranexamic acid (1 g loading dose then 1 g over 8 h) or placebo.
The study demonstrated that all-cause mortality was significantly lower in the tranexamic acid group compared to placebo (14.5 % vs 16 %; relative risk 0.91; 95 % CI 0.85–0.97, P = 0.0035). Also death due to bleeding was reduced from 5.7 to 4.9 % (p = 0.0077).
After the publication of CRASH-2 study, a number of systematic reviews, meta-analysis, and randomized clinical trials have been published in the setting of trauma [67] and surgical bleeding [68], in pediatric noncardiac surgery [69], and in orthopedic surgery [70, 71].
The topical application of tranexamic acid reduces the blood loss by 29 % (pooled ratio 0.71, 95 % CI 0.69–0.72; p < 0.0001) versus placebo as recently documented in a Cochrane systematic review [72].
6.4.3 Monitoring the Efficacy of Antifibrinolytic Drugs
Whole-blood viscoelastic tests are established methods for the detection of fibrinolysis. Fibrinolysis is usually determined by detecting more than 15 % breakdown in clot strength as compared to MCF within 1 h after clotting time (maximum lysis, ML >15 %) in INTEM, EXTEM, and FIBTEM. After the administration of antifibrinolytic drugs, INTEM, EXTEM, and FIBTEM reveal stable clot trace (Fig. 6.3). Similar response is observed at TEG tests, where the clot lysis is expressed in terms of % changes in the MA.
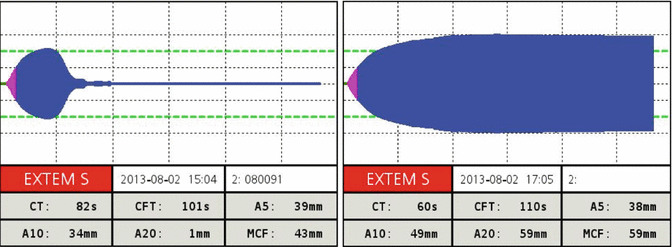
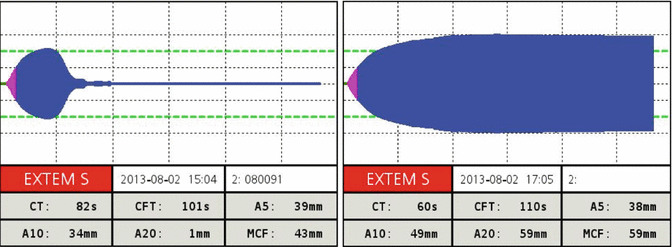
Fig. 6.3
ROTEM trace of fulminant hyperfibrinolysis in a severely injured patient pre and post administration of 2g of tranexamic acid
6.4.4 Adverse Events
The most frequently reported side effects are gastrointestinal symptoms such as nausea and vomiting. Concerns have been raised regarding the prothrombotic potential and the increased risk of vascular events related to antifibrinolytic drugs. No increase in the rate of myocardial infarction, stroke, deep vein thrombosis, or pulmonary embolism has been described in recent meta-analysis and CRASH-2 trial.
6.5 Fibrinogen
Fibrinogen (factor I) is a plasma-soluble protein synthetized in the liver. It is the first clotting factor to decrease to critically low levels during hemorrhage, because the hepatic synthesis remains inadequate to compensate for the deficit in case of massive blood loss [73].
Fibrinogen plays an essential role in coagulation and is a central element of hemostasis [74]. It has a dual role as it enhances platelet aggregation and it is converted into fibrin to form an insoluble clot. During primary hemostasis, platelets become activated, resulting in an activation of platelet GPIIb/IIIa receptors that become the docking station for fibrinogen, linking platelets to platelets and thereby stabilizing the platelet plug. Activated platelets present highly efficacious surfaces for plasma coagulation, resulting in the thrombin burst. As a result, fibrinogen is converted to fibrin and factor XIII (FXIII) is activated. The activated FXIII cross-link the soluble fibrin into solid fibrin strands [75, 76].
Low preoperative fibrinogen levels have been associated with increased perioperative bleeding and the need for transfusion of blood products [50, 77–82].
Thus, fibrinogen supplementation to restore plasma levels is an important component for normalizing clot formation in bleeding patients with low fibrinogen levels.
Fibrinogen concentrate is a plasma-derived, pasteurized, and lyophilized human fibrinogen, manufactured from pooled plasma donations that undergo purification, viral inactivation, and removal processes. The risk of immunological and allergic reactions is greatly reduced by viral inactivation and removal processes [83]. Fibrinogen concentrate is available for almost immediate use as no screening for blood type is needed and can be stored at room temperature (2–25 °C); thus, relatively high doses can be administered in a short time.
In addition to the advantages mentioned above, fibrinogen concentrate can be used to deliver a standardized dose, with a concentration of 0.9–1.3 g/vial (final concentration of 20 g/L), unlike fresh frozen plasma and cryoprecipitate, which contain variable amounts of fibrinogen.
Haemocomplettan/RiaSTAP (CSL Behring) is the only fibrinogen concentrate globally available and is licensed in a number of countries for multiple indications including treating acute bleeding episodes with hypofibrinogenemia. Other fibrinogen concentrate products with more limited availability are licensed in specific countries such as China, Japan, and France (Clottagen/Clottafact, Fibrinogen HT, FibroRAAS) [84].
Fibrinogen concentrate is widely used to correct hypofibrinogenemia with the goal of reducing coagulopathy, bleeding, and transfusion requirements. In particular, a number of clinical trials have demonstrated the efficacy of fibrinogen concentrate in aortic and cardiac surgery [85, 86]. Furthermore fibrinogen concentrate administration has been described as effective and safe in trauma patients and patients with postpartum hemorrhage by retrospective analyses and several systematic reviews [18, 87–94].
Recently, the European Society of Anaesthesiology (ESA) published guidelines for the management of perioperative bleeding which recommends substitution therapy with fibrinogen concentrate if significant bleeding is accompanied by at least suspected hypofibrinogenemia (Grade 1C: Strong recommendation, but with low quality of evidence) and suggests including fibrinogen concentrate in goal-directed treatment algorithms [51].
6.5.1 Monitoring the Efficacy of Fibrinogen Supplementation
Fibrinogen concentrate dosing should depend upon bleeding status and laboratory or point-of-care test results. The maximum clot firmness (MCF) in the FIBTEM test on the ROTEM device (or the equivalent parameter maximum amplitude, MA, in the functional fibrinogen assay on the TEG device) has been used extensively to determine fibrinogen levels [77, 85, 95, 96]. The fibrinogen dose can be calculated as follows:
![$$ \begin{array}{l}\mathrm{F}\mathrm{ibrinogen}\;\mathrm{concentrate}\;\mathrm{dose}\;\left(\mathrm{g}\right)\\ {}=\left(\begin{array}{l}\mathrm{target}\;\mathrm{F}\mathrm{IBTEM}\;\mathrm{M}\mathrm{C}\mathrm{F}\;\left[\mathrm{mm}\right]\\ {}-\mathrm{actual}\;\mathrm{F}\mathrm{IBTEM}\;\mathrm{M}\mathrm{C}\mathrm{F}\left[\mathrm{mm}\right]\end{array}\right)\\ {}\kern0.96em \times \left(\mathrm{bodyweight}\;\left[\mathrm{kg}\right]/70\right)\times 0.5\;\mathrm{g}\;/\kern0.1em \mathrm{mm}\end{array} $$](/wp-content/uploads/2017/07/A326222_1_En_6_Chapter_Equa.gif)
![$$ \begin{array}{l}\mathrm{F}\mathrm{ibrinogen}\;\mathrm{concentrate}\;\mathrm{dose}\;\left(\mathrm{g}\right)\\ {}=\left(\begin{array}{l}\mathrm{target}\;\mathrm{F}\mathrm{IBTEM}\;\mathrm{M}\mathrm{C}\mathrm{F}\;\left[\mathrm{mm}\right]\\ {}-\mathrm{actual}\;\mathrm{F}\mathrm{IBTEM}\;\mathrm{M}\mathrm{C}\mathrm{F}\left[\mathrm{mm}\right]\end{array}\right)\\ {}\kern0.96em \times \left(\mathrm{bodyweight}\;\left[\mathrm{kg}\right]/70\right)\times 0.5\;\mathrm{g}\;/\kern0.1em \mathrm{mm}\end{array} $$](/wp-content/uploads/2017/07/A326222_1_En_6_Chapter_Equa.gif)
Normal MCF values are 9–25 mm, and they should correlate with a normal fibrinogen levels [77]; however, a target MCF of 22 mm has been used in aortic surgery patients (achieved using mean fibrinogen doses of 5.7 g) [97]. In the algorithm by Weber, 25 mg/kg fibrinogen concentrate is recommended if EXTEM A10 and FIBTEM A10 are below 40 mm and 8 mm, respectively. If FIBTEM A10 is <6 mm and EXTEM A10 is <40 mm, the recommended dose increases to 50 mg/kg [98]. According to the recent ESA guidelines, the recommended initial dose for adults is 25–50 mg/kg (children: 30–50 mg/kg) in the perioperative management [51] or 3–4 g in trauma management [50] (Fig. 6.4).
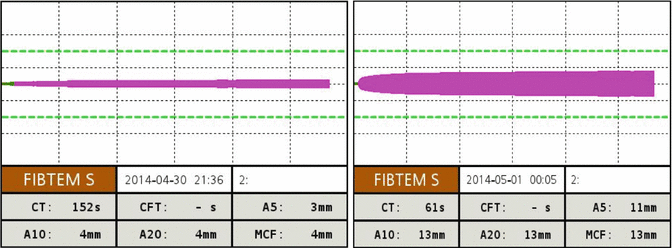
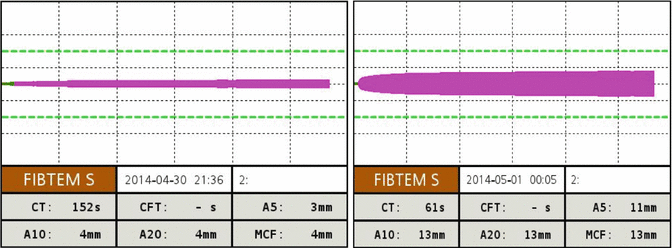
Fig. 6.4
FIBTEM ROTEM trace in a severely injured patient pre and post administration of 4 g of fibrinogen concentrate
However, the critical threshold for fibrinogen substitution and the target level to achieve are still a matter of debate, and the value of 22 mm at FIBTEM should be considered arbitrary.
6.5.2 Adverse Events
When administering a prohemostatic factor such as fibrinogen concentrate, the risk of thromboembolic complication should be considered. However, in a recent published descriptive analysis of over 27 years of pharmacovigilance, data indicates that the rate of adverse drug reactions reported following the administration of Haemocomplettan P/RiaSTAP across diverse clinical settings is low. In this analysis the authors identified approximately 4.3 thromboembolic events (TEE) per 105 treatment episodes (calculated from 28 cases reporting a possible TEE, corresponding to one case for every 23,300 standard doses of 4 g each) including all reported TEEs, regardless of their relationship to fibrinogen concentrate. Moreover, no allergic reactions or virus transmission events were identified during this literature review [99].
6.5.3 Desmopressin
Desmopressin (DDAVP, 1-deamino-8-d-arginine vasopressin) is a synthetic vasopressin derivate that increases plasma concentration of factor VIII (FVIII), von Willebrand factor (vWF), and tissue plasminogen activator (t-PA). It also has a vasodilatory action via activation of endothelial nitric oxide synthase.
Its effect on vWF and t-PA is likely explained by a direct action on the endothelium. It is postulated that activation of endothelial V2 receptors results in c-AMP-mediated exocytosis of vWF and t-PA from Weibel-Palade bodies where both are stored [100]. As a result of the increase in vWF, there is an increase in the number of FVIII binding sites; FVIII is therefore better protected against proteolytic breakdown. Desmopressin also induces the release and membrane presentation of P-selectin, which mediates platelet rolling on endothelial cells under high shear conditions [101]. A recent study demonstrated that desmopressin increases endothelial adhesiveness for platelets and platelet adhesion to collagen by releasing a proadhesive factor, likely vWF, from endothelial cells [102].
6.5.4 Indications
DDAVP has a proven hemostatic efficacy in mild hemophilia A, in von Willebrand disease (VWD), in uremia and liver cirrhosis as well as in congenital and acquired, drug-induced platelet dysfunction [103].
Desmopressin has been used as a hemostatic agent in patients without preexisting coagulation disorders undergoing high blood loss surgeries such as cardiac, spinal, and orthopedic procedures. A meta-analysis of 38 randomized, placebo-controlled trials indicated that desmopressin (usually at a dose of 0.3 μg/kg) had a statistically significant (albeit clinically modest) effect on reducing perioperative bleeding and transfusion of blood components without significant increased risk of thromboembolic complications [104].
In patients undergoing liver resection, desmopressin does not reduce transfusion requirement [105].
Although there is no evidence for DDAVP routine use after cardiac surgery, desmopressin may be considered whenever bleeding is suspected to stem from GPIIb/IIIa inhibitors and other antiplatelet agents [106] and in the setting of acquired von Willebrand disease (aortic stenosis patients) [107].
However, there is no convincing evidence that desmopressin minimizes perioperative bleeding or perioperative allogeneic blood transfusion in patients without a congenital bleeding disorder [51].
A decreased biological response has been observed after DDAVP doses repeated over short time intervals (tachyphylaxis), due likely to a V2 receptors desensitization or a slow vWf stores replenished [100].
6.5.5 Adverse Events
Adverse effects of DDAVP include mild facial flushing, headache, palpitations, and hypotension. Desmopressin should be used with caution in cases of severe congestive heart failure because of its potent antidiuretic effect. An increased risk of thrombotic events (i.e., myocardial infarction, stroke, arterial thrombosis, venous thromboembolism) has been reported [108, 109]. DDAVP should, therefore, be used with caution in patients with coronary artery disease.
6.6 Prothrombin Complex Concentrates
Prothrombin complex concentrates (PCCs) represent a highly purified concentrate of coagulation factors prepared from pooled normal plasma and contain a heterogeneous combination of coagulation factors and counterbalancing inhibitor components.
On market three-factor PCCs (containing factor II, IX and X) and four-factor PCCs (containing factor II, VII, IX and X) are available..
Initially PCCs were developed for the treatment of hemophilia B as a source of factor IX, and, to date, they are standardized on the basis of factor IX content. Concentrations of the other coagulation factors differ between batches.
6.6.1 Indications
1.
Reversal of vitamin K antagonists in major, life-threatening bleeding or for urgent surgery
PCCs replenish the coagulation factors suppressed by vitamin K antagonists (warfarin, acenocoumarol, or phenprocoumon) together with the administration of vitamin K i.v. [110–113]. The recommended dosages are related to the patient body weight and the INR value. Generally PCCs 25–50 IU/kg plus 10 mg of vitamin K are administered [114] with the goal to lower the INR below 1.5 as early as possible [113, 115]. This is particularly true in cases of intracranial hemorrhage which represent a serious anticoagulant-related bleeding complication, even though there is no solid evidence from literature of an improvement in prognosis [116, 117]. A close monitoring of INR is required in order to adjust quickly dosages in cases of unsatisfactory reversal.
There is a well-known inverse no linear relationship between factor VII levels and INR, and for INR values over 4, the level of factor VII is under 5–10 %. It is reasonably believed that the non-complete reversal of higher INR values by 3 factors PCCs is due to the low content of factor VII [118].
Although randomized control trials do not exist to compare directly the efficacy and safety of 4-factor PCCs versus 3-factor PCCs, recent guidelines suggest the use of 4-factor PCCs because of the presence of anticoagulant factor proteins C and S. These more balanced drugs achieve a rapid reversal of anticoagulation at a higher rate [114, 115, 117, 119–121].
2.
Reversal of direct oral anticoagulants (direct thrombin inhibitors, direct factor Xa inhibitors)
Direct oral anticoagulants do not have specific antidotes, although this is an area of intense research. Treatment approach for the reversal of their effects derives mainly from studies on animals or in vitro studies or on healthy volunteers [122, 123].
3.
Major bleeding without vitamin K antagonists
In the past few years, several reports have been published on the efficacy of PCCs in the management of major bleeding in patients not on oral anticoagulation.
Schols and associates [127, 128] demonstrated an increased risk of postoperative bleeding in patients with a low thrombin generation, while in vitro studies showed the possibility to restore thrombin generation through the infusion of fibrinogen concentrate and PCCs [129–131]. Based on these studies, recent guidelines [50, 51] suggest to administer PCCs at the dosages of 20–30 U/kg in bleeding patients with abnormal coagulation results (INR >1.5 or EXTEM clotting time >80 s).
4.
Treatment and prophylaxis of bleeding in congenital vitamin K-dependent factors deficiency
PCCs are the treatment of choice in congenital bleeding disorders in case the specific factor concentrate is not available.
6.6.2 Monitoring the Efficacy of PCCs Therapy
It is possible to monitor the efficacy of PCCs treatment with POC devices such as ROTEM or TEG. A reduction of vitamin K coagulation factors cause a prolongation of clotting time (EXTEM ROTEM) or (TEG) which return in normal range after the administration of PCCs (Fig. 6.5).
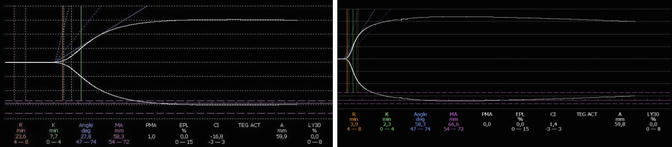
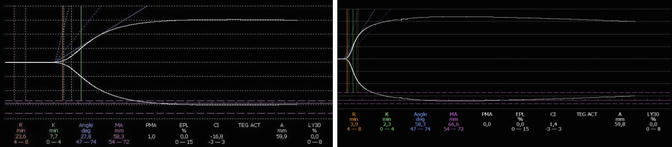
Fig. 6.5
TEG trace in a patient on oral anticoagulant therapy pre and post administration of 30UI/kg of 3-factor PCC
6.6.3 Adverse Events
Potential adverse events related to PCCs infusion are allergic reaction, alloantibody development, chills, pyrexia, disseminated intravascular dissemination, and thromboembolic events [136] (microvascular thrombosis and myocardial infarction).
6.7 Bypassing Agents: Activated Prothrombin Complex Concentrates (aPCC) and Recombinant Factor VIIa
Bypassing agents consist of activated prothrombin complex concentrates (aPCC) and recombinant factor VIIa (rVIIa). There is only one manufactured rFVIIa (NovoSeven; Novo Nordisk, Bagsvaerd, Denmark) and one factor eight inhibitor bypassing activity (FEIBA; Baxter Bioscience, Vienna, Austria).
Their bypassing activities induce and facilitate thrombin generation, via pathways that do not require factor VIII and IX, but for which factor X and factor V play critical roles. It should, however, be emphasized that the two drugs are dissimilar even though both lead to thrombin generation and fibrin formation in patients with inhibitors [137, 138].
It is important to underline that neither of these two agents are able to completely restore thrombin generation in contrast to factor VIII and IX administration. Both agents have an overall efficacy rate above 80 % with similar rates of adverse events [139, 140].
FEIBA is a mixture of vitamin K-dependent coagulation factors obtained from a pooled human plasma after removal of cryoprecipitate and include factor II (prothrombin), factor VII, factor IX, and factor X and a small amount of factor IXa, Xa, and thrombin and a larger amount of factor VIIa [141–144]. The protein complex, apart from routine tests for pharmacological products, is subjected to a two-step vapor heat-treatment procedure for inactivation of lipid and non-lipid-enveloped viruses.
Originally it was believed rFVIIa would drive the activation of factor X via TF-FVIIa complex, bypassing the need for FVIII and FIX in hemophiliac patients. Later it was demonstrated that rFVIIa can directly activate FX on a negatively charged phospholipid surface in the absence of TF [145]. Furthermore at high doses [146], it can form the complex TF-VIIa competing with zymogen FVII for binding TF and can activate factor IX directly on the surface of activated platelets independent of FVIIIa or IXa [147–149]. To date, although a TF-dependent mechanism of action is not ruled out, it is believed that the major contributor to the hemostatic effect of rFVIIa is the ability to induce the thrombin burst on the platelet surface.
6.7.1 Indications
The principal indication for FEIBA and rFVIIa is the treatment of bleeding in patients with hemophilia A and B with inhibitors.
The usual dosages for FEIBA are 50–100 U/kg every 6–12 h with a maximum daily dose of 200 U/kg, while rFVIIa is administered at the dose of 90 mcg/kg given every 2–3 h by bolus infusion until hemostasis is achieved. For severe bleeding, the treatment with rFVIIa should continue at 3–6 h intervals to maintain hemostatic plug.
In acquired hemophilia rFVIIa is infused at the dosage of 70–90 mcg/kg repeated every 2–3 h until hemostasis is achieved. For the management of bleeding in congenital factor VII deficiency, dosages of 15–30 mcg/kg every 4–6 h until hemostasis is achieved are recommended.
Recently FEIBA and rFVIIa have been proposed as reversal hemostatic drugs in patients on direct oral anticoagulants who need urgent or emergency surgery or in case of life-threatening hemorrhage (e.g., intracranial or in critical organs) at the dose of 30–50 U/kg [150–152] and 90 mcg/kg, respectively.
rFVIIa has been used also in the treatment of acquired bleeding episodes in patients on oral anticoagulants, in intracranial hemorrhage, in traumatic bleeding, in postpartum hemorrhage, in cardiac surgery [153], and in surgical bleeding as rescue therapy.
Nevertheless the published CONTROL trial [154] failed to demonstrate a mortality benefit in using rFVIIa in blunt and penetrating trauma, and the study was terminated early. In this phase 3 randomized clinical trial, patients were assigned to receive 200 mcg/kg initially and 100 mcg/kg at 1 and 3 h or a placebo. Despite the fact that the rate of allogeneic blood transfusion was reduced in the rFVIIa arm, there wasn’t any effect on mortality.
Safety data on 4468 subjects (4119 patients and 349 healthy volunteers) were recently analyzed [155] to determine the frequency of thromboembolic events. A higher rate of arterial thrombosis has been described in patients who received rFVIIa compared to placebo, while the rate of venous thromboembolism was similar in the two arms (rFVIIavs placebo).
6.7.2 Adverse Events
Further safety concerns derived from a Cochrane Systematic Review [156] of 29 randomized clinical trials comparing rFVIIa with a placebo for the prevention or treatment of bleeding in patients without hemophilia. Sixteen trials involved 1361 participants with prophylactic use of rFVIIa and 13 trials involved 2929 patients receiving therapeutic doses. The results indicated a trend toward an increased risk of arterial thromboembolic events in patients receiving rFVIIa (RR 1.14; 95 % CI 0.89–1.47).
Choice of bypassing agents depends on the current clinical situation, efficacy for single patient, and convenience.
6.7.3 Monitoring the Efficacy of Bypassing Agents
Clinical responsiveness to bypassing agents is difficult to predict, and no validated laboratory method is available for monitoring their therapeutic efficacy [157, 142].

Full access? Get Clinical Tree
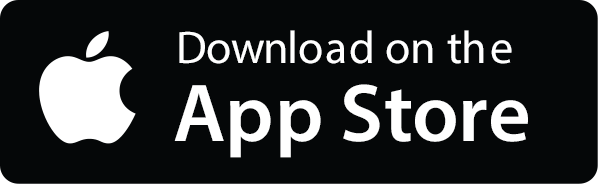
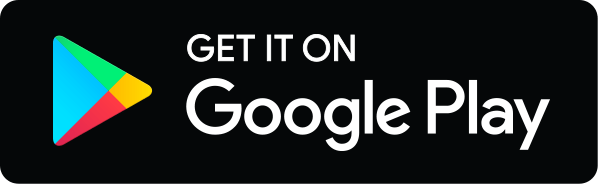